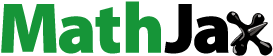
Abstract
Purpose: To evaluate the rate and dose response of brachial plexus toxicity post stereotactic body radiation therapy (SBRT) of apically situated lung lesions.
Material/methods: We retrospectively identified all patients with apically located tumors, defined by the epicenter of the tumor being located superiorly to the aortic arch, and treated with SBRT between 2008 and 2013. Patients with a shorter follow-up than 6 months were excluded. Primary aim was to evaluate radiation-induced brachial plexopathy (RIBP). Dose to the plexus was assessed by a retrospective delineation of the brachial plexus on the CT used for treatment planning. Then, Dmax, D0.1cc, D1cc and D3.0cc of the brachial plexus were collected from the dose-volume histograms (DVH) and recalculated to the biologically effective dose (BED) using α/β = 3 Gy. A normal tissue complication probability (NTCP) model, based on four different dose-volume parameters (BED3,max, BED3,0.1cc, BED3,1.0cc, BED3,3.0cc) was fitted to the data.
Results: Fifty-two patients with 56 apically located tumors were identified. Median prescription dose per fraction was 15 Gy (range 6–17) and median number of fractions was 3 (3–10). With a median follow-up of 30 months (6.1–72) seven patients experienced maximum grade 2 (scored 3 times) or 3 (scored 4 times) RIBP after a median of 8.7 months (range 4.0–31). Three patients had combined symptoms with pain, sensory and motor affection and four patients had isolated pain. Median BED3,max for the patients experiencing RIBP was 381 Gy (range 30–524) versus BED3,max of 34 Gy (range 0.10–483) for the patients without RIBP. The NTCP models showed a very high predictive ability (area under the receiver operating characteristic curve (AUC) 0.80–0.88).
Conclusion: SBRT of apically located lung lesions may cause severe neurological symptoms; for a three-fraction treatment, we suggest that the maximum dose to the plexus should be kept ≤30 Gy (130 Gy BED3).
Introduction
During the last decade stereotactic body radiation therapy (SBRT) has emerged as an efficient and well-tolerated treatment option for patients with medically inoperable early stage non-small cell lung cancer (NSCLC) and lung metastases. Results from clinical trials have shown acceptable side effects [Citation1,Citation2] but also witness of an increased risk for adverse events when treating centrally [Citation3] and apically located lung tumors [Citation4]. Radiation-induced brachial plexopathy (RIBP) – a disorder characterized by numbness, pain, paresthesia and motor deficit [Citation5] – may develop if the tolerance dose of the brachial plexus is exceeded. Historically, RIBP has mostly been addressed in hypofractionated radiotherapy of breast [Citation6–12] and lung cancer [Citation9,Citation13,Citation14], with fraction doses in the range of 2.5–6.0 Gy increasing the risk [Citation8] of severe physical impairment and suffering of the affected patients. Risk factors for RIBP in conventionally fractionated radiotherapy include higher total dose [Citation15,Citation16], shortened overall treatment time [Citation16] and larger than standard fraction doses (>2Gy/fraction) [Citation12]. Cytotoxic treatment has also been found to exacerbate the effect of radiotherapy [Citation12]. The current knowledge regarding the tolerance of the brachial plexus of large fraction doses in the context of SBRT is limited [Citation17–19]. It is important to further enlighten this issue due to the rapid spread of SBRT. We performed this retrospective analysis to evaluate the occurrence of RIBP in correlation to absorbed doses to the brachial plexus after SBRT of apically situated lung lesions.
Material and methods
Patient cohort
All patients treated with SBRT at Karolinska University Hospital, Stockholm, Sweden, between 2008 and 2013 were retrospectively reviewed (approximately 1000 patients) and all apically located treated lesions were considered for inclusion in the study. Apical location was defined as the epicenter of the tumor being localized superior to the aortic arch. No minimum prescription dose was imposed but patients with a follow-up less than 6 months post SBRT were excluded. Primary aim was to evaluate radiation-induced brachial plexopathy (RIBP) defined as pain, sensory alterations and/or motor weakness in the upper extremity presenting post radiotherapy, ipsilateral to the treated tumor and with no other likely cause. Clinical data were retrieved from the patient records and radiotherapy data from the treatment planning system. Toxicity was scored according to the CTCAE v 4.0 where grade 1 indicates asymptomatic effects, grade 2 indicates moderate symptoms limiting instrumental ADL (activity of daily living) and grade 3 indicates severe symptoms limiting self-care ADL. Grade 4 and 5 do not exist in this scale. Follow-up of the patients was done according to clinical routine i.e., regular CT-scans and out-patient visits approximately every three months during the first two years and thereafter every six months for patients with curatively treated primary NSCLC, while metastatic patients were followed on an individual basis. The study was approved by the regional ethical committee (Dr: 2014/1581-31) in Stockholm.
Radiotherapy
The technique used for SBRT immobilization and treatment planning has been described in detail in previous reports [Citation1,Citation20,Citation21]. In summary, the clinical target volume (CTV) comprised the gross tumor volume (GTV) including the diffuse growth at the borders and the margin to the PTV was 5–10 mm in the transversal plane and 10 mm in the longitudinal plane. SBRT was delivered with 6 MV from a linear accelerator, after immobilizing the patients in a stereotactic body frame (Elekta AB, Stockholm, Sweden). Fifty tumors were treated with 4–12 static fields and 6 tumors were treated with VMAT technique using 2–7 arcs. The dose was prescribed to about the 67% isodose line encompassing the planning target volume (PTV). Tumor movement was assessed with 4D-CT and image guidance was used by daily online cone-beam CT. The patients were planned using the AAA dose-calculation algorithm in the Eclipse treatment planning system (Varian). Dmax (maximum dose), D0.1cc (dose to the ‘hottest’ 0.1 cc), D1cc (dose to hottest 1 cc) and to D3.0cc (dose to the hottest 3 cc) of the brachial plexus were collected from the dose-volume histograms (DVH) and recalculated to the biologically effective dose using α/β = 3Gy, i.e., BED3, max, BED3, 0.1cc, BED3, 1cc, and BED3, 3cc. Prescribed tumor doses were recalculated to BED10.
Delineation of the brachial plexus
The inferior parts of the brachial plexus ( depicted in red), being at risk of exposure to significant doses from SBRT of superiorly located lung lesions, were delineated retrospectively on the non-contrast-enhanced CT used for treatment planning. First, adjacent critical structures (the 7th cervical vertebrae (C7), the 1st and 2nd thoracic vertebras (Th1, Th2), the subclavian and axillary neurovascular bundle, the first rib and the anterior and middle scalene muscles) were identified. Next, the brachial plexus was delineated as shown in , starting at the neural foramina C8 – Th1 (in a few cases even Th2) and eventually ending in the axilla or proximal arm. The arm nerves were included in the delineation to the extent that they could be identified as a coherent bundle and verified on the treatment-planning CT. When required to improve the accuracy of the delineation, existing diagnostic images (CT-scans with or without contrast and MRI) were used and the delineation was approved by a radiologist especially designated the study (V.G). The greatest uncertainty in anatomical definition of the plexus prevailed where the upper and medial trunks pass between the scalene muscles, an area where the absorbed doses were typically low.
Figure 1. Anatomical definition and delineation of the brachial plexus. We used a modified version of the RTOG-contouring guidelines to delineate the inferior parts of the plexus, which are at risk for high absorbed doses at lung-SBRT. The delineated parts are marked with red and comprise the parts of the plexus in level with and inferior to C8, including the inferior trunk and the cords extending along the subclavian and axillary artery, a short fragment of the upper trunk running between mm scalenus anterior et medium, as well as parts of the proximal arm nerves distally to the plexus. Source: Ferrante MA [Citation16]. Wiley Periodicals, Inc.© 2004 Wiley Periodicals, Inc. Modified with permission
![Figure 1. Anatomical definition and delineation of the brachial plexus. We used a modified version of the RTOG-contouring guidelines to delineate the inferior parts of the plexus, which are at risk for high absorbed doses at lung-SBRT. The delineated parts are marked with red and comprise the parts of the plexus in level with and inferior to C8, including the inferior trunk and the cords extending along the subclavian and axillary artery, a short fragment of the upper trunk running between mm scalenus anterior et medium, as well as parts of the proximal arm nerves distally to the plexus. Source: Ferrante MA [Citation16]. Wiley Periodicals, Inc.© 2004 Wiley Periodicals, Inc. Modified with permission](/cms/asset/78ec3688-d758-4ca3-a3c0-bf78c9bd6085/ionc_a_1601255_f0001_c.jpg)
NTCP-modeling
A normal tissue complication probability (NTCP) model with the outcome set to ≥ grade 2 RIBP, based on each of the dose-volume-parameters, was fitted to the data, using the maximum likelihood method. A cumulative normal function was used:
where
Here, φ represents each dose/volume parameter in turn (Dmax, D0.1cc, D1cc and D3.0cc) , φ50 is the value of the dose/-volume-parameter which results in NTCP = 50%, and m is a parameter relating to the slope of the sigmoid function. The model was fitted with φ converted to both BED (α/β =3 Gy) and Single Fraction Equivalent Dose (SFED). The latter was used as an additional parameter for biological response, as the LQ model used in BED calculations has been questioned for high dose/fraction[Citation22]. SFED was calculated with the Universal Survival Curve model (USC) as [Citation22]
Where d is the dose per fraction and nr is the number of fractions. The model parameter values used were α/β = 3 Gy, α = 0.206 Gy−1, n = 10 and Do=1.0 Gy and dT = 5.8 Gy [Citation23].
As a measure of model performance, the area under the receiver operating characteristic curve (AUC) was calculated for each model fit.
Results
Patient cohort
Fifty-two patients treated for 56 tumors were eligible for the study. In these patients, 55 brachial plexuses were at risk for developing RIBP. Thirty patients were treated with curative intent of a primary non-small cell lung cancer (T1a = 15, T1b = 6, T2a = 9) (See for details of the patient cohort). Four patients were treated for two tumors; three patients for bilateral tumors at separate occasions and one patient was treated for two left-sided lesions (BED3, max <1.3 Gy to the plexus at the first treatment). Prior to SBRT, one patient had mild tumor-related pain in the upper extremity. Post SBRT, one patient developed neurological symptoms post-treatment which however were attributed to a large recurrence, and the patient was therefore not scored as having treatment-induced toxicity. Median follow-up of the cohort was 30 months (6.1–72). Median survival was 30 months (7.4–72). Two patients suffered local failures.
Table 1. Patient-, tumor- and treatment characteristics.
Dose characteristics
Fifty-six tumors were treated within the study with a median prescribed dose per fraction of 15 Gy (6–17 Gy) and a median number of fractions of 3 (3–10). Please see for details on tumor characteristics and doses to different parts of the brachial plexus. There was a large variability in the dose delivered to the brachial plexus within the patient cohort; ) shows dosimetric data for each brachial plexus at risk. Twenty patients exceeded a BED3,max of 113 Gy which corresponds to 68 Gy EQD2 (a commonly used dose in curative treatment of NSCLC) and 6 (30%) of these patients developed RIBP (median follow-up 18.5 months).
Figure 2. Doses to the brachial plexus for each patient. BED3,max (A), BED3,0.1cc (B), BED3,1cc (C) and BED3,3cc (D) for each treatment. The patients with RIBP are presented in red columns. The patient marked with an * previously received BED3,max to the plexus of 123 Gy and BED3,3cc of 111 Gy, why the true dose to the plexus for this patient is underestimated in these graphs.
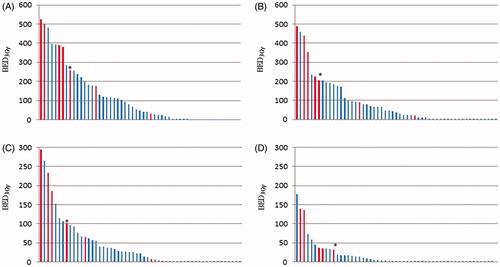
Radiotherapy regimens outside the analysis
Thirteen patients received additional radiotherapy to the lung ipsilaterally to the ‘study tumor’; ten with clinically non-significant doses to the brachial plexus (BED3,max of ≤3.1 Gy to the plexus). One patient had previously received 90 Gy to the plexus followed by 116 Gy from the treatment included in the study; another patient had previously received 103 Gy to the plexus and then 2.4 Gy from the treatment included in the study (all BED3,max). The last patient, who had previously received BED3,max=123 Gy to the plexus and 260 Gy from the treatment included in the study, subsequently developing RIBP (see below). Unfortunately, the plexus was not taken into consideration as an organ at risk (OAR) at the time of either of these treatments. All previous treatments rendering clinically significant doses were given with conventionally fractionated radiotherapy (CFRT) with 2 Gy/fraction.
Radiation-induced brachial plexopathy
We identified 7 out of 52 patients (13%) with symptoms consistent with RIBP; four patients with grade 3 and three patients with grade 2. The first sign of symptom appeared after a median time of 5.8 months (0.70–13 months) and the worst recorded grade appearing at 8.7 months (4–31 months) post treatment. The prescription doses for these patients were 15 Gy × 3 (n = 5), 10 Gy × 5 (n = 1) and 6 Gy × 10 (n = 1) corresponding to a BED10 ranging between 96–113 Gy. None of these patients had local failure, received neurotoxic chemotherapy or had diabetes as potential contributors to the development of RIBP. The diagnosis of radiation-induced neurological symptoms was made either clinically (n = 4) or combined with neurophysiologic examinations (n = 3).
presents tumor characteristics and doses to the plexus for the patients with and without RIBP respectively. On the average, the patients who developed RIBP had a shorter distance between the tumor and the plexus (distance CTV-plexus 4 mm vs 24 mm) and an increased frequency of overlapping of the PTV and the brachial plexus (57% vs 15%) as compared to patients without these symptoms. (Supplementary table I presents maximum recorded overall toxicity)
Table 2. Characteristics of patients with and patients without radiation induced brachial plexus symptoms.
Patient descriptions
The treatment-planning CT:s for the patients with RIBP are shown in (the patient numbers are also valid for and supplementary figure 1). Three patients had sensory alterations and/or motor weakness in combination with extremity pain (no 1–3) and 4 patients had isolated upper extremity pain (no 4–7). One patient (no 2) previously received radiochemotherapy (68Gy) for a locally advanced NSCLC and was 15 months later re-irradiated with SBRT to an apical recurrence. The SUV-uptake on the PET-CT was used as guidance for the target delineation at SBRT and unintentionally, both the CTV and PTV overlapped the plexus. The patient received 123 Gy (BED3,max) at the first treatment and 260 Gy (BED3,max) at the second treatment. Neurophysiologic examinations showed signs of brachial plexopathy corresponding to the level C7-Th1 and affecting the ulnar and median nerves. Patient no 3 also developed severe medical impairment and neurophysiologic examinations showed signs of a pronounced plexus lesion in the lower part of the plexus. Patient no1 presented with signs of RIBP 13 months post SBRT, eventually developing to a total paralysis of the arm. She was diagnosed with RIBP by neurophysiological examinations, and also with a rupture of the supraspinatus tendon, both findings clinically reflecting severe over-dosage of the region.
Figure 3. Dose-planning CT-scans of the patients with brachial toxicity. All these patients except for patient number 5 had a PTV-plexus distance of ≤2 mm and received BED3,max between 177–524 Gy. The same patient numbers (1–7) are also valid for and Supplementary Figure 1.
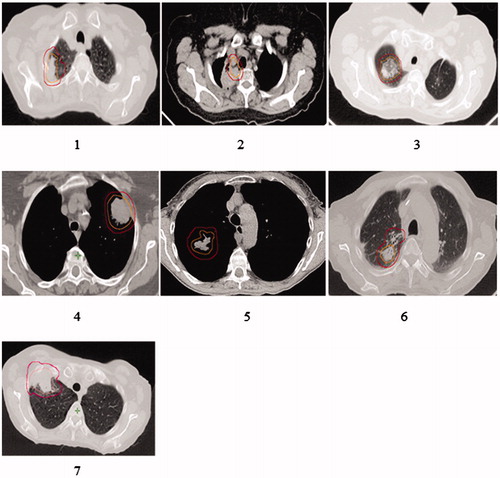
For this study, we retrieved clinical follow-up-data on the patients from the medical records and monitored the development of the symptoms. Follow-up from the first sign of symptoms until last follow-up ranged between 0.8–57 months for these patients, making it possible to evaluate the dynamics of the symptoms for a long time. The symptoms started with either pain or numbness, and over time progressed to pain in combination with numbness and eventually pain, numbness and motor weakness developed. (Electronic Supplement Figure I). The worst affected patients had progressive symptoms, showing no sign of recovery. Interestingly, one patient (no 6) only had severe pain presenting at one occasion at 8.7 months post-SBRT, and another patient (no 4) had pain which resolved after 11 months.
NTCP-modeling
The NTCP models give good fits to the dataset both for BED and SFED: shows the fit for BED3,max. The high model performance is confirmed by the AUC values listed in together with model parameter values. The maximum dose models seem to perform particularly well, but this is not statistically significant (p > .05).
Figure 4. The BED3,max NTCP-model for the brachial plexus. The green diamonds represent cases without RIBP and the red crosses represent cases with RIBP. BED3,max of 130 Gy is marked with a dotted blue line.
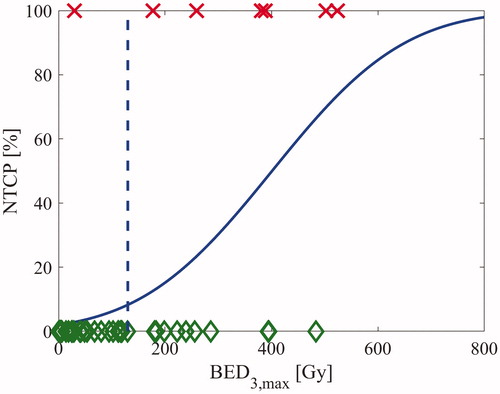
Table 3. Performance of the NTCP-modeling.
Discussion
We here report the results of a retrospective analysis of SBRT of superiorly located lung lesions in 52 patients with 55 brachial plexuses at risk for developing RIBP. Seven patients (13%) developed grade 2–3 RIBP and the NTCP-model showed a good fit of the data for BED3,max as dosimetric parameter.
Treating apically located lung lesions with radiotherapy is a difficult task; the importance of delivering an ablative dose to the tumor to avoid tumor-induced brachial plexopathy – a condition even more disabling than RIBP [Citation14] – has to be balanced against the potential risk of causing brachial plexus morbidity. The tolerance dose of the brachial plexus with CFRT has been considered in the range of 60–62 Gy (for 3/3–1/3 irradiation of the organ) in 1.8–2-Gy-fractions (BED3,max =96-110 Gy) [Citation24] and recent RTOG-protocols for head and neck cancer use a planning constraint of Dmax ≤ 60–66 Gy [Citation25]. One trial on NSCLC has suggested the possibility to push these constraints even further, reporting no toxic events with Dmax ≤78 Gy in 1.8–2-Gy-fractions (BED3,max = 125–130 Gy) [Citation14] . To our knowledge, RIBP post SBRT has been limitedly addressed in the literature [Citation4,Citation26–30]. Based on 36 patients with seven cases of RIBP, Forquer et al [Citation4] estimated the 2-year-risk (KM-method) of grade 2–4 RIBP to 46% if Dmax > 26 Gy versus 8% if Dmax ≤ 26 Gy for 3–4 fractions (statistically significant) and they recommended a planning constraint of Dmax < 26 Gy for three- or four-fraction treatments (corresponding to BED3,max=101Gy and 82 Gy respectively). Another group recommended a higher dose constraint of Dmax ≤ 35 Gy (BED3,max = 137 Gy) and V30Gy ≤ 0.2 cc (BED3 = 105 Gy) for four fractions [Citation27]. A recently published analysis of 31 apically located lung tumors treated with SBRT in 3–5 fractions, did not report any brachial-plexus toxicity, the median total Dmax to the brachial plexus being 15.8 Gy (1.7–66.5 Gy) and only four patients receiving a Dmax/fraction greater than 10 Gy [Citation29]. One report assessing radiation-induced brachial plexus injury post stereotactic spine radiosurgery (SRS), showed a 2.5% occurrence of peripheral nervous system injury (including brachial plexus injury) after SRS, but the prescribed dose was not a significant risk factor and no doses to organs-at-risk were presented [Citation28]. In our analysis, 6 of the 7 patients (86%) experiencing RIBP had BED3,max > 130 Gy, but there was a large gap of patients at risk between the doses BED3,max = 177 Gy and 129 Gy (). The NTCP model predicted a complication probability of <10% for BED3,max = 130 Gy. Hence, for 3 fractions, the true Dmax tolerance level is probably >26 Gy and our model suggest Dmax ≤ 30 Gy as a reasonable planning constraint. Our proposed constraint is higher as compared to that suggested in the recently updated National Comprehensive Cancer Network (NCCN) version 3 guidelines, which recommends a maximum dose to the plexus of 24 Gy and 27.2 Gy for a 3- and 4-fraction treatment respectively. This recommendation is based on dose constraints from protocols in recent RTOG-trials (RTOG 0618, 0813, 0915) [Citation31]. It should also be mentioned that although the development of RIBP may be affected by the dose to a certain volume of the plexus [Citation13,Citation14], the BED3,max NTCP-model showed the best fit of the dataset () out of the dose-volume parameters considered.
Interestingly, despite receiving BED3,max >130 Gy to the plexus, 10 patients (median follow-up 19 months (6.6–49) in our cohort did not develop RIBP (). The lack of toxicity in this high-dose subgroup may be explained by several factors such as the uncertainty of dose assessment (considering the small OAR and steep dose gradients), the underreporting of side effects in a retrospective cohort, the distribution of the high-dose area, the use of ‘risk-adapted’ fractionation schedules, the limited follow-up and the individual radiosensitivity.
A challenging question is whether ‘risk-adapted’ fractionation schedules with potentially wider therapeutic window for better protection of the normal tissue, exist. The NTCP modeling in this analysis was performed using both the Linear Quadratic model and the Universal Survival Curve model, the latter theoretically predicting a greater benefit (compared to LQ) in therapeutic window from increased fractionation, at doses/fraction above dT (in this study 5.8 Gy was used) [Citation23]. However, no difference was seen between the models and therefore the traditional LQ-model was presented graphically (). Among the ten patients without RIBP receiving BED3,max >130 Gy 8 patients were treated with 3 fractions and 2 patients were treated with 8 fractions. Unfortunately, four patients, including all patients treated with 8 fractions, had a follow-up of less than 13 months, a time frame within which all patients with diagnosed RIBP in our cohort presented with their first symptom. If the follow-up had been longer, there is still a possibility that these patients eventually would have developed RIBP. Hence, we could not confirm the protective normal tissue effect of increasing the number of fractions based on our cohort.
The plexus is a fine structure directly adjacent to other soft-tissue structures, complicating the delineation of it. The conclusions of this study rely on the accuracy of the delineation of the plexus and on whether the doses extracted from the DVH:s (based on the planning CT) are representative of the doses actually delivered to the plexus. We used a modified version of the RTOG-contouring protocol [Citation32], which has been criticized for inter-observer variability, however, only resulting in minimal inconsistency of maximum doses [Citation33]. The greatest uncertainty in delineation prevailed in the lateral apical parts of the plexus, generally far from the high dose region in this study. Further, the accuracy of the delineation of the plexus could have been improved by using a contrast-enhanced treatment-planning CT and the usage of thin slices for delineation. Also, although knowing that apical structures of the lung have limited breathing motions, it cannot be ruled out that the plexus may have shifted positions during the breathing cycle, an uncertainty that we cannot foresee or correct for. Taking these uncertainties and limitations into consideration in addition to set-up- and intra-treatment-motion uncertainties, we believe that a planning organ at risk volume (PRV) to the plexus of 2 mm may be advisable to use, with a BED3,max planning constraint in the order of 170 Gy. The sparing of a PRV, however, has to be balanced in each case against the risk of tumor growth into the plexus, causing pronounced cancer morbidity symptoms.
There are some limitations to our study. The analysis is based on retrospective data from a small patient cohort with limited follow-up which may lead to uncertainties in interpreting toxicity symptoms and limits the possibilities of statistical testing. Except for the SBRT treatment included in the study, some patients also received other courses of radiotherapy delivering dose to the plexus. The impact of these other radiotherapy regimens is difficult to evaluate and this uncertainty in dose assessment is a limitation of the analysis. However, only one of the patients with RIBP had received additional radiotherapy affecting the plexus with a clinically significant dose and our conclusion is therefore that RIBP for six of the seven patients was caused by the current treatment only. Another consideration is the diagnostic accuracy of RIBP. The patients with sensory/motor alterations (n = 3) had all been diagnosed with neurophysiologic examination showing signs of plexopathy attributed to radiation therapy. Four patients, developing pain only, were graded as RIBP based on clinical symptoms and no objective signs could be established, which is a notable limitation. However, a strength of the study is that two independent observers (S.L and K.L) graded the toxic symptoms and the delineation of the plexus was performed/approved by one person (V.G).
Conclusion
RIBP may cause severe medical impairment and it is therefore important to consider the brachial plexus as an organ-at-risk at delineation. However, a balance between saving the plexus and delivering an ablative dose to the tumor must be kept to avoid local failure and tumor-induced brachial plexopathy, which may be utterly handicapping [Citation14]. Based on the results from this analysis, we suggest that the maximum dose to the plexus should be kept ≤30 Gy (BED3,max = 130 Gy) for a 3-fraction schedule.
Supplemental Material
Download Zip (197 KB)Disclosure statement
No potential conflict of interest was reported by the authors.
Additional information
Funding
References
- Baumann P, Nyman J, Hoyer M, et al. Stereotactic body radiotherapy for medically inoperable patients with stage I non-small cell lung cancer - a first report of toxicity related to COPD/CVD in a non-randomized prospective phase II study. Radiother Oncol. 2008;88:359–367.
- Timmerman R, Paulus R, Galvin J, et al. Stereotactic body radiation therapy for inoperable early stage lung cancer. JAMA. 2010;303:1070–1076.
- Timmerman R, McGarry R, Yiannoutsos C, et al. Excessive toxicity when treating central tumors in a phase II study of stereotactic body radiation therapy for medically inoperable early-stage lung cancer. JCO. 2006;24:4833–4839.
- Forquer JA, Fakiris AJ, Timmerman RD, et al. Brachial plexopathy from stereotactic body radiotherapy in early-stage NSCLC: dose-limiting toxicity in apical tumor sites. Radiother Oncol. 2009;93:408–413.
- Schierle C, Winograd JM. Radiation-induced brachial plexopathy: review. Complication without a cure. J Reconstr Microsurg. 2004;20:149–152.
- Dropcho EJ. Neurotoxicity of radiation therapy. Neurol Clin. 2010;28:217–234.
- Delanian S, Lefaix JL, Pradat PF. Radiation-induced neuropathy in cancer survivors. Radiother Oncol. 2012;105:273–282.
- Friberg S, Ruden BI. Hypofractionation in radiotherapy. An investigation of injured Swedish women, treated for cancer of the breast. Acta Oncol. 2009;48:822–831.
- Harper CM, Jr., Thomas JE, Cascino TL, et al. Distinction between neoplastic and radiation-induced brachial plexopathy, with emphasis on the role of EMG. Neurology. 1989;39:502–506.
- Kori SH, Foley KM, Posner JB. Brachial plexus lesions in patients with cancer: 100 cases. Neurology. 1981;31:45–50.
- Mondrup K, Olsen NK, Pfeiffer P, et al. Clinical and electrodiagnostic findings in breast cancer patients with radiation-induced brachial plexus neuropathy. Acta Neurol Scand. 1990;81:153–158.
- Olsen NK, Pfeiffer P, Johannsen L, et al. Radiation-induced brachial plexopathy: neurological follow-up in 161 recurrence-free breast cancer patients. Int J Radiat Oncol Biol Phys. 1993;26:43–49.
- Amini A, Yang J, Williamson R, et al. Dose constraints to prevent radiation-induced brachial plexopathy in patients treated for lung cancer. Int J Radiat Oncol Biol Phys. 2012;82:e391–e398.
- Eblan MJ, Corradetti MN, Lukens JN, et al. Brachial plexopathy in apical non-small cell lung cancer treated with definitive radiation: dosimetric analysis and clinical implications. Int J Radiat Oncol Biol Phys. 2013;85:175–181.
- Stoll BA, Andrews JT. Radiation-induced peripheral neuropathy. Br Med J. 1966;1:834–837.
- Ferrante MA. Brachial plexopathies: classification, causes, and consequences. Muscle Nerve. 2004;30:547–568.
- Kang KH, Okoye CC, Patel RB, et al. Complications from stereotactic body radiotherapy for lung cancer. Cancers. 2015;7:981–1004.
- De Rose F, Franceschini D, Reggiori G, et al. Organs at risk in lung SBRT. Phys Med. 2017;44:131–138.
- Hanna GG, Murray L, Patel R, et al. UK consensus on normal tissue dose constraints for stereotactic radiotherapy. Clin Oncol. 2018;30:5–14.
- Baumann P, Nyman J, Hoyer M, et al. Outcome in a prospective phase II trial of medically inoperable stage I non-small-cell lung cancer patients treated with stereotactic body radiotherapy. JCO. 2009;27:3290–3296.
- Baumann P, Nyman J, Lax I, et al. Factors important for efficacy of stereotactic body radiotherapy of medically inoperable stage I lung cancer. A retrospective analysis of patients treated in the Nordic countries. Acta Oncol. 2006;45:787–795.
- Park C, Papiez L, Zhang S, et al. Universal survival curve and single fraction equivalent dose: useful tools in understanding potency of ablative radiotherapy. Int J Radiat Oncol Biol Phys. 2008;70:847–852.
- Wennberg B, Lax I. The impact of fractionation in SBRT: analysis with the linear quadratic model and the universal survival curve model. Acta Oncol. 2013;52:902–909.
- Emami B, Lyman J, Brown A, et al. Tolerance of normal tissue to therapeutic irradiation. Int J Radiat Oncol Biol Phys. 1991;21:109–122.
- Mutter RW, Lok BH, Dutta PR, et al. Constraining the brachial plexus does not compromise regional control in oropharyngeal carcinoma. Radiat Oncol. 2013;8:173.
- Chang JY, Balter PA, Dong L, et al. Stereotactic body radiation therapy in centrally and superiorly located stage I or isolated recurrent non-small-cell lung cancer. Int J Radiat Oncol Biol Phys. 2008;72:967–971.
- Chang JY, Li QQ, Xu QY, et al. Stereotactic ablative radiation therapy for centrally located early stage or isolated parenchymal recurrences of non-small cell lung cancer: how to fly in a "no fly zone". Int J Radiat Oncol Biol Phys. 2014;88:1120–1128.
- Stubblefield MD, Ibanez K, Riedel ER, et al. Peripheral nervous system injury after high-dose single-fraction image-guided stereotactic radiosurgery for spine tumors. Neurosurg Focus. 2017;42:E12.
- Sood SS, McClinton C, Badkul R, et al. Brachial plexopathy after stereotactic body radiation therapy for apical lung cancer: dosimetric analysis and preliminary clinical outcomes. Adv Radiat Oncol. 2018;3:81–86.
- Sharma M, Bennett EE, Rahmathulla G, et al. Impact of cervicothoracic region stereotactic spine radiosurgery on adjacent organs at risk. Neurosurg Focus. 2017;42:E14.
- https://www.nccn.org/professionals/physician_gls/pdf/nscl.pdf [last accessed 19 March 2019].
- Hall WH, Guiou M, Lee NY, et al. Development and validation of a standardized method for contouring the brachial plexus: preliminary dosimetric analysis among patients treated with IMRT for head-and-neck cancer. Int J Radiat Oncol Biol Phys. 2008;72:1362–1367.
- Min M, Roos D, Keating E, et al. External evaluation of the Radiation Therapy Oncology Group brachial plexus contouring protocol: several issues identified. J Med Imaging Radiat Oncol. 2014;58:360–368.