Abstract
Background: Children with brain tumors undergoing radiotherapy are at particular risk of radiation-induced morbidity and are therefore routinely considered for proton therapy (PT) to reduce the dose to healthy tissues. The aim of this study was to apply pediatric constraints and normal tissue complication probability (NTCP) models when evaluating the differences between PT and contemporary photon-based radiotherapy, volumetric modulated arc therapy (VMAT).
Methods: Forty patients (aged 1–17 years) referred from Norwegian institutions to cranial PT abroad during 2014–2016 were selected for VMAT re-planning using the original CT sets and target volumes. The VMAT and delivered PT plans were compared by dose/volume metrics and NTCP models related to growth hormone deficiency, auditory toxicity, visual impairment, xerostomia, neurocognitive outcome and secondary brain and parotid gland cancers.
Results: The supratentorial brain, temporal lobes, hippocampi, hypothalamus, pituitary glands, cochleas, salivary glands, optic nerves and chiasm received lower mean doses from PT. Reductions in population median NTCP were significant for auditory toxicity (VMAT: 3.8%; PT: 0.3%), neurocognitive outcome (VMAT: 3.0 IQ points decline at 5 years post RT; PT: 2.5 IQ points), xerostomia (VMAT: 2.0%; PT: 0.6%), excess absolute risk of secondary cancer of the brain (VMAT: 9.2%; PT: 6.7%) and salivary glands (VMAT: 2.8%; PT:0.5%). Across all patients, 23/38 PT plans had better or comparable estimated risks for all endpoints (within ±10% of the risk relative to VMAT), whereas for 1/38 patients all estimates were better or comparable with VMAT.
Conclusions: PT reduced the volumes of normal tissues exposed to radiation, particularly low-to-intermediate dose levels, and this was reflected in lower NTCP. Of the included endpoints, substantial reductions in population medians were seen from the delivered PT plans for auditory complications, xerostomia, and risk of secondary cancers of the brain and salivary glands.
Introduction
Long-term survival following childhood cancer now exceeds 80% [Citation1], resulting in an increased emphasis on quality of life after treatment. Radiotherapy (RT) is an effective treatment of many childhood brain tumors, however, a successful cure may be associated with radiation-related side effects impacting organ function, growth and development [Citation2,Citation3]. More specifically, cranial RT increases the risk of neurocognitive decline [Citation4–7], endocrine problems [Citation8–10], auditory toxicity [Citation11–14], visual impairment [Citation14], xerostomia [Citation15] and risk of secondary cancers [Citation16–18].
Children are generally more radiosensitive than adults and are routinely considered for proton therapy (PT) intended to reduce the radiation dose to healthy tissues. Whereas several planning studies have demonstrated the dosimetric advantages and estimated outcome of PT compared to modern photon-based RT [Citation19–24], it is critical to ensure that the compared plans are clinically realistic and deliverable and should, therefore, be performed in close collaboration with treating institutions. Munck af Rosenschold et al. [Citation25] concluded that patients with intracranial tumors could benefit from collaborative comparative planning prior to referral with respect to estimated cognitive dysfunction and growth hormone deficiency (GHD). In patients with marginal benefit, this exercise might avoid the extra cost and inconvenience of long travel for patients and their families. The conclusions were limited, however, by the absence of patient-specific risks across different domains as well as consideration of low-dose effects such as radiation carcinogenesis.
The lack of conclusive follow-up data across a diverse patient population calls for methods to better quantify the benefits of PT over modern photon-based techniques [Citation26]. The model-based approach was originally designed to select adult patients for PT [Citation27] in cases with the primary aim of reducing side-effects while maintaining cure rates. With the rapid introduction of novel technology and procedures, the model-based approach has also been suggested as a promising method in the assessment of childhood cancers [Citation26]. Normal tissue complication probability (NTCP) models addressing the pediatric population are limited, therefore the aim of this study was to explore how dosimetric differences in children referred for PT are reflected in existing pediatric models when compared to treatment with volumetric modulated arc therapy (VMAT).
Methods
Patients and treatment plan material
In total, 48 children were referred to curative PT from Norwegian hospitals during 2014–2016, based on age, diagnosis and health status, i.e., the degree of urgency and fitness to travel. Of the 48 patients, 40 cases received significant radiation doses to the brain and/or head and neck region (thereby excluding extracranial tumors only) and were selected for comparison with VMAT. All treatment records and delivered PT plans were collected from the University of Florida Health Proton Therapy Institute (US) and Heidelberg Ion Beam Therapy Center (Germany). Twenty-five of the delivered proton plans were passively modulated PT optimized in Eclipse (Varian Medical Systems, Palo Alto, CA, USA) and the remaining 15 were intensity-modulated PT (IMPT) plans optimized in Syngo RT Planning (Siemens Healthcare, Erlangen, Germany). All proton plans were delivered by 3–7 fields (details in [Citation28]) with clinical target volume (CTV) dose prescriptions ranging from 50.4 to 72 Gy(RBE) in 1.8–2.0 Gy(RBE) fractions, using 3 mm margins for the PTV. Median patient age was 7.5 years (range 1–17) including 22 males and 18 females. An overview of the patient population is presented in Table S1.
Contouring and re-planning with volumetric modulated arc therapy
For each individual proton plan, a corresponding (6 MV) VMAT plan was generated in Eclipse or RayStation (RaySearch Laboratories AB, Stockholm, Sweden) using the original CT simulation, and subsequently approved according to the planning protocol (based on QUANTEC [Citation29] and Dahanca guidelines [Citation30]) by a dosimetrist/medical physicist and oncologist at the referring hospital. The target volumes and the majority of the organs at risk (OAR)s were delineated at the treating institution. The planners were blinded and matched the target coverage of the delivered PT plan. Two craniospinal irradiation (CSI) patients were excluded due to technical issues with the re-planning/original CTs (patients #11 and 12). Some of the OARs were later added for the analysis, including the supratentorial brain, parotid glands [Citation30], hippocampi [Citation11,Citation31] and temporal lobes [Citation32], but otherwise not included in the optimization. All structures were reviewed by an experienced oncologist and modified when necessary to ensure consistency across patients.
Normal tissue morbidity models
Clinical complication probabilities were estimated using pediatric-specific dose/volume criteria and NTCP models of growth hormone levels [Citation8,Citation11,Citation33], neurocognition [Citation5–7,Citation34,Citation35], hearing [Citation11–14], vision [Citation36,Citation37], salivary gland function [Citation15,Citation38] and radiation carcinogenesis of the brain and salivary glands [Citation39]. Further details can be found in Supplementary Methods.
Analysis and statistics
Cumulative dose volume histograms (DVHs) for all plans were used to estimate NTCP, as well as the proportion of patients below dose-volume thresholds associated with risks of toxicity (Table S2). Differences in estimated risk between the two techniques were presented both in terms of relative and absolute risk differences. The relative difference in risk was estimated as: risk(superior technique)/risk(inferior technique), and the number of patients exceeding 10% relative reduction in risk was presented (i.e., risk reduced to 90% of the inferior technique’s risk estimate). A relative reduction of >10% compared to the inferior technique was defined as being lower risk, whereas a relative reduction in risk <10% was defined as comparable risk between the two techniques. For the relative comparisons, a lower absolute risk cutoff to exclude the patients close to baseline risks were set at NTCP <1%, and in the case of cognitive decline <1 IQ point. In addition, the absolute difference between the risk estimates, ΔNTCP (or Δrisk), was calculated for each patient and endpoint as riskVMAT − riskPT. Statistical testing was done using Wilcoxon signed-rank tests for paired samples and p-values <.05 were considered significant. Data handling, calculations and statistical analyses were performed using Matlab- and Python-based in-house software [Citation23].
Results
Comparison of dose/volume metrics
Endocrine structures received lower mean doses in the delivered proton plans, with 47% of the patients below the indicated 16 Gy threshold of the hypothalamus for both RT modalities (), and with 66% (PT) and 58% (VMAT) below 30 Gy for the pituitary gland (see Table S3 for overview of dose/volume results). The PT plans presented with significantly lower volumes receiving 20 Gy/Gy(RBE) (V20Gy) and mean organ doses (Dmean) compared to VMAT for all cognitive structures (), whereas higher dose/volume cognitive parameters (V30Gy/V43Gy/V50Gy) were more comparable across the techniques. The medians of cochlear Dmean were 2.2 Gy(RBE) for PT versus 17.7 Gy with VMAT (Figure S1), corresponding to 82% of the PT plans within the indicated threshold for ototoxicity and 72% of the VMAT plans. For the optic nerves and chiasm, 93% of the patients were below the indicated dose threshold with both plan modalities (Figure S2). The median Dmean of the salivary glands was 8.8 Gy with VMAT and 0.0 Gy(RBE) with PT, respecting the indicated threshold of the least irradiated parotid gland in 89% (VMAT) and 95% (PT) of the patients (Figure S3). Overall, the volumes of low- and intermediate- doses were considerably reduced with the delivered PT plans compared to VMAT re-plans.
Figure 1. (a) Dose metrics for endocrine structures per patient compared for VMAT and proton plans. Pediatric dose threshold indicated. (b) Estimated probability of complication per patient. Equal dose and risk indicated by solid line, ±10% relative difference in risk indicated by dashed line. Excluding patients with risk <1%, gives 14/38 patients in favor of PT by 10% relative difference in risk (upper left side), and 6/38 in favor of VMAT (lower right side).
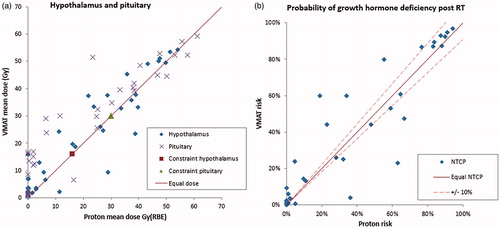
Figure 2. Dose metrics related to cognition compared for VMAT and proton plans per patient for (a) the supratentorial brain, (b) the temporal lobes and (c) the hippocampi. (d) Estimated probability of decline in IQ points complication for a patient. Equal dose and risk indicated by solid line, ±10% relative difference in risk indicated by dashed line.
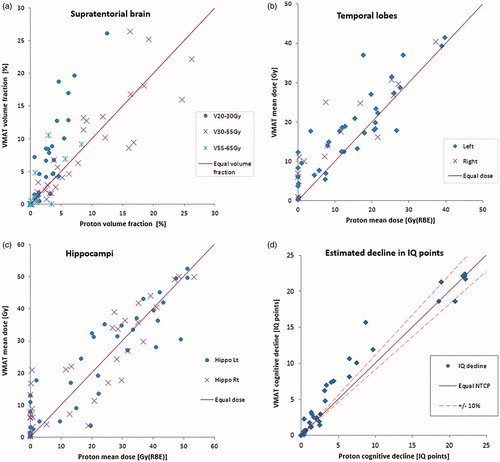
Normal tissue complication probability
The estimated reduction in population median risks was statistically significant for all investigated endpoints except for GHD. For this endpoint, we found median NTCPs of 21% with PT and 23% with VMAT, and 14/38 patients had a relative reduction >10% with PT versus 6/38 with VMAT (/). The estimated population median GHD risk was reduced in both PT and VMAT plans when excluding the six patients receiving CSI, yielding a statistically significant difference in NTCP of PT (7%) and VMAT (11%) for the remaining patients (Table S4).
Table 1. Estimated risk of complications.
The estimated decline in IQ (/) ranged from 0 to 22 points for both RT modalities and with 19/38 patients having >10% lower estimated risk with PT compared to VMAT, whereas 4/38 patients were better with VMAT. NTCP of tinnitus was lower with PT for 39/76 of the audio structures in the patients, compared to 5/76 for VMAT (Figure S1). The median risk of visual complications was close to baseline risk with both modalities (Figure S2), resulting in noteworthy NTCP values in only 3/38 patients for both PT and VMAT plans. Risk of xerostomia was lower in 20/38 patients with PT compared to 4/38 with VMAT (Figure S3). Estimated risk of radiation-induced secondary cancer () of the brain was >10% lower for 21/38 patients with PT, and vice versa in 4/38 patients with VMAT. Corresponding values for secondary cancers of the salivary glands were >10% reduction in 23/36 patients with PT compared to VMAT, and in 1/38 with VMAT compared to PT.
Figure 3. Estimated risk of radiation-induced secondary cancer of the (a) brain and (b) the parotid glands. Solid line indicates equal risk, with ±10% relative deviation in risk indicated by the dashed line.
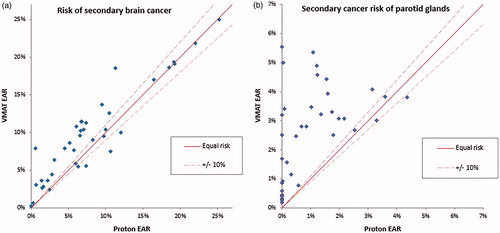
In terms of relative differences between risks across all patients (Figure S4), 23/38 PT plans were better or comparable within 10% of the estimated risk relative to VMAT, 14/38 patients had estimated risks in favor of both PT and VMAT, whereas for 1/38 patients the estimates were comparable or better with VMAT.
The absolute difference (ΔNTCP) in estimated risks revealed trends both across patients and endpoints (Figure S5), with individual patients and patient groups with a stronger indication for one technique with respect to specific endpoints. Whereas ΔNTCP also demonstrated the overall indication in favor of PT, some individual endpoints were in favor of VMAT. In particular, some of the patients receiving CSI (#5–9) had lower NTCP for GHD with VMAT, although with auditory NTCP strongly in favor of PT. Three ependymoma patients (#15–17) had 3–5 endpoints in favor of VMAT, as well as 4–5 endpoints in favor of PT.
Discussion
Across the organs reviewed, we consistently observed a reduction in low-to-intermediate doses in the clinically delivered proton plans compared to VMAT, whereas the high-dose volumes were more comparable. Broadly, the estimated NTCP and risk of secondary malignancy for the studied population were lower from PT than from VMAT. Organs located in the low-to intermediate-risk regions with VMAT were often reduced to baseline risk with PT, yet occasional patients with organs at high risk demonstrated lower NTCP with VMAT.
GHD is usually the first and most frequent endocrine problem occurring after pediatric cranial RT [Citation9], and may reach 50–100% at doses of 30–50 Gy to the pituitary gland and/or hypothalamus [Citation8]. GHD also resulted in the highest risk for our patient cohort for both PT and VMAT (ranging up to 97%), and although this is a challenge to avoid, hormone replacements are available to childhood cancer survivors who are in remission. The variation in estimated GHD across our patients was wide, also including patients with lower NTCP from VMAT compared to PT. These patients included medulloblastoma and ependymoma patients, mainly found at intermediate GHD risk levels with both treatment modalities, a finding similar to a previous comparison of photon-based RT and PT in 10 medulloblastoma patients [Citation25].
Most protocols do not include the parotid glands as OARs in case of CSI, which both could influence the risk of xerostomia and secondary cancers. King et al. [Citation40] found that relevant parotid dose constraints were not respected in half of the patients receiving photon-based CSI. The majority of the patients in our cohort were, however, well below the indicated dose thresholds. In addition, the plans demonstrating the low-to-intermediate risk of xerostomia with VMAT had substantially lower risk from PT. Nevertheless, two CSI patients had a higher risk with PT, reflecting the potential of considering the parotid as an OAR in PT.
Whereas both protons and VMAT have advantages with respect to sparing normal tissue doses compared to conventional techniques, the major concern about the broad implementation of VMAT has been the increase in low-dose volumes [Citation41], with potential consequences for secondary cancer incidence in children. While the value of PT in reducing low-dose volumes for large extracranial volumes (e.g., CSI [Citation23]) is widely acknowledged, our data suggests that the value of such low-dose reduction extends also to children with CNS and skull base tumors requiring focal radiation. Compared to VMAT, the estimated median EAR in our cohort was reduced by 27 and 82% for radiation carcinogenesis of the brain and salivary tumors, respectively. In fact, this represents one of the largest advantages of PT among the studied endpoints (only exceeded by a reduction in auditory toxicity). Whereas the dose-response relationship of secondary cancers has been much debated, particularly for high-dose volumes, it is well known that low-dose exposure increases the risk of secondary malignancies [Citation42,Citation43]. Now with the option to reduce low-dose exposure to the brain, we have a clear opportunity to reduce this feared toxicity.
Despite the dosimetric superiority of PT, VMAT provides better-estimated outcomes compared to conventional photon-based techniques [Citation41]. Similar to earlier research [Citation26], our data support that reasonable alternative treatment may be provided with VMAT for many patients where PT is not a practical option due to difficulties with travel, treatment urgency, or palliative settings where the patient would not be expected to realize the long-term benefits of PT.
Whereas the model-based approach was not originally intended for pediatric patients, our methodology and findings suggest that model-based plan selection could play a valuable role in selecting children who would benefit the most from PT. Moreover, robust modeling will allow optimization of plan design within each modality, for example, CSI with an added emphasis on parotid sparing dose distribution. Model-based plan selection in pediatric patients requires accurate information on dose thresholds and clear ideas of endpoint prioritization. A prerequisite for reliable models in small, heterogeneous patient populations such as pediatric tumors is having high-quality input data to generate robust models. This is the rationale behind the current efforts of large registries [Citation44–46] and the planned PENTEC reports [Citation47].
Since PT both has a different physical dose distribution as well as a variable RBE [Citation48], models based on photon techniques may be inadequate. Including RBE models in risk, estimates could capture more of the variations in tissue sensitivity, and better reflect plan-specific RBE depending on tumor location and beam configuration [Citation49]. This could potentially adjust the risk for some of our investigated endpoints, however, the biological uncertainties should be relatively modest [Citation48] compared to the estimated risk differences between PT and VMAT, and therefore would not influence the overall observed trends across patients and endpoints. Nevertheless, RBE models may be used to refine risk optimization in PT and further advice selectivity of organs that would benefit additional dose sparing. Ultimately, combining follow-up data from different techniques may provide missing data spanning dose levels not reachable from one technique alone and thus provide more versatile models applicable across RT modalities.
In conclusion, PT reduced the volumes of normal tissues exposed to radiation, also reflected in the estimated model-based risks. Future models with increased sensitivity across both low and high dose levels may offer more accurate toxicity prediction, which in turn would inform rational strategies of dose reduction and technology utilization.
Ethics approval
The study has been reviewed and approved by the Regional Committees for Medical and Health Research Ethics (REC) according to Norwegian law and regulations (REK No. 2016/1636).
Supplemental Material
Download PDF (702.7 KB)Disclosure statement
No potential conflict of interest was reported by the authors.
Additional information
Funding
References
- Mody R, Li S, Dover DC, et al. Twenty-five-year follow-up among survivors of childhood acute lymphoblastic leukemia: a report from the Childhood Cancer Survivor Study. Blood 2008;111:5515–5523.
- Robison LL, Hudson MM. Survivors of childhood and adolescent cancer: life-long risks and responsibilities. Nat Rev Cancer. 2014;14:61–70.
- Armstrong GT, Stovall M, Robison LL. Long-term effects of radiation exposure among adult survivors of childhood cancer: results from the childhood cancer survivor study. Radiat Res. 2010;174:840–850.
- Lawrence YR, Li XA, el Naqa I, et al. Radiation dose-volume effects in the brain. Int J Radiat Oncol Biol Phys. 2010;76:20–27.
- Merchant TE, Kiehna EN, Li C, et al. Modeling radiation dosimetry to predict cognitive outcomes in pediatric patients with CNS embryonal tumors including medulloblastoma. Int J Radiat Oncol Biol Phys. 2006;65:210–221.
- Armstrong GT, Jain N, Liu W, et al. Region-specific radiotherapy and neuropsychological outcomes in adult survivors of childhood CNS malignancies. Neuro Oncol. 2010;12:1173–1186.
- Redmond KJ, Mahone EM, Horska A. Association between radiation dose to neuronal progenitor cell niches and temporal lobes and performance on neuropsychological testing in children: a prospective study. Neuro Oncol. 2013;15:1455.
- Darzy KH. Radiation-induced hypopituitarism after cancer therapy: who, how and when to test. Nat Rev Endocrinol. 2009;5:88–99.
- Mulder RL, Kremer LC, van Santen HM, et al. Prevalence and risk factors of radiation-induced growth hormone deficiency in childhood cancer survivors: a systematic review. Cancer Treat Rev. 2009;35:616–632.
- Laughton SJ, Merchant TE, Sklar CA, et al. Endocrine outcomes for children with embryonal brain tumors after risk-adapted craniospinal and conformal primary-site irradiation and high-dose chemotherapy with stem-cell rescue on the SJMB-96 trial. JCO. 2008;26:1112–1118.
- Scoccianti S, Detti B, Gadda D, et al. Organs at risk in the brain and their dose-constraints in adults and in children: a radiation oncologist’s guide for delineation in everyday practice. Radiother Oncol. 2015;114:230–238.
- Paulino AC, Lobo M, Teh BS, et al. Ototoxicity after intensity-modulated radiation therapy and cisplatin-based chemotherapy in children with medulloblastoma. Int J Radiat Oncol Biol Phys. 2010;78:1445–1450.
- Lee TF, Yeh SA, Chao PJ, et al. Normal tissue complication probability modeling for cochlea constraints to avoid causing tinnitus after head-and-neck intensity-modulated radiation therapy. Radiat Oncol. 2015;10:194.
- Mayo C, Martel MK, Marks LB, et al. Radiation dose-volume effects of optic nerves and chiasm. Int J Radiat Oncol Biol Phys. 2010;76:28–35.
- Moiseenko V, Wu J, Hovan A, et al. Treatment planning constraints to avoid xerostomia in head-and-neck radiotherapy: an independent test of QUANTEC criteria using a prospectively collected dataset. Int J Radiat Oncol Biol Phys. 2012;82:1108–1114.
- Bhatia S, Sklar C. Second cancers in survivors of childhood cancer. Nat Rev Cancer. 2002;2:124–132.
- Neglia JP, Robison LL, Stovall M, et al. New primary neoplasms of the central nervous system in survivors of childhood cancer: a report from the Childhood Cancer Survivor Study. J Natl Cancer Inst. 2006;98:1528–1537.
- Boukheris H, Stovall M, Gilbert ES, et al. Risk of salivary gland cancer after childhood cancer: a report from the Childhood Cancer Survivor Study. Int J Radiat Oncol Biol Phys. 2013;85:776–783.
- Merchant TE, Hua CH, Shukla H, et al. Proton versus photon radiotherapy for common pediatric brain tumors: comparison of models of dose characteristics and their relationship to cognitive function. Pediatr Blood Cancer. 2008;51:110–117.
- Brower JV, Indelicato DJ, Aldana PR, et al. A treatment planning comparison of highly conformal radiation therapy for pediatric low-grade brainstem gliomas. Acta Oncol. 2013;52:594–599.
- Boehling NS, Grosshans DR, Bluett JB, et al. Dosimetric comparison of three-dimensional conformal proton radiotherapy, intensity-modulated proton therapy, and intensity-modulated radiotherapy for treatment of pediatric craniopharyngiomas. Int J Radiat Oncol Biol Phys. 2012;82:643–652.
- Brodin NP, Vogelius IR, Maraldo MV, et al. Life years lost-comparing potentially fatal late complications after radiotherapy for pediatric medulloblastoma on a common scale. Cancer 2012;118:5432–5440.
- Stokkevåg CH, Engeseth GM, Ytre-Hauge KS, et al. Estimated risk of radiation-induced cancer following paediatric cranio-spinal irradiation with electron, photon and proton therapy. Acta Oncol. 2014;53:1048–1057.
- Zhang R, Howell RM, Taddei PJ, et al. A comparative study on the risks of radiogenic second cancers and cardiac mortality in a set of pediatric medulloblastoma patients treated with photon or proton craniospinal irradiation. Radiother Oncol. 2014;113:84–88.
- Munck af Rosenschold P, Engelholm SA, Brodin PN, et al. A retrospective evaluation of the benefit of referring pediatric cancer patients to an external proton therapy center. Pediatr Blood Cancer. 2016;63:262–269.
- Weber DC, Habrand JL, Hoppe BS, et al. Proton therapy for pediatric malignancies: Fact, figures and costs. A joint consensus statement from the pediatric subcommittee of PTCOG, PROS and EPTN. Radiother Oncol. 2018;128:44–55.
- Langendijk JA, Lambin P, De Ruysscher D, et al. Selection of patients for radiotherapy with protons aiming at reduction of side effects: the model-based approach. Radiother Oncol. 2013;107:267–273.
- Haas-Kogan D, Indelicato D, Paganetti H, et al. National Cancer Institute Workshop on proton therapy for children: considerations regarding brainstem injury. Int J Radiat Oncol Biol Phys. 2018;101:152–168.
- Marks LB, Yorke ED, Jackson A, et al. Use of normal tissue complication probability models in the clinic. Int J Radiat Oncol Biol Phys. 2010;76:10–19.
- Danish Head and Neck Cancer Group. DAHANCA Radiotherapy Guidelines 2018 [Internet]. Aarhus, Denmark: DAHANCA; [cited 2019 Feb 1]. Available from: https://www.dahanca.dk/
- Radiation Therapy Oncology Group. RTOG Contouring Atlases 2019 [Internet]. Philadelphia, PA: RTOG. [cited 2019 Feb 15]. Available from: https://www.rtog.org/CoreLab/ContouringAtlases.aspx
- Sun Y, Yu XL, Luo W, et al. Recommendation for a contouring method and atlas of organs at risk in nasopharyngeal carcinoma patients receiving intensity-modulated radiotherapy. Radiother Oncol. 2014;110:390–397.
- Merchant TE, Rose SR, Bosley C, et al. Growth hormone secretion after conformal radiation therapy in pediatric patients with localized brain tumors. JCO. 2011;29:4776–4780.
- Jalali R, Mallick I, Dutta D, et al. Factors influencing neurocognitive outcomes in young patients with benign and low-grade brain tumors treated with stereotactic conformal radiotherapy. Int J Radiat Oncol Biol Phys. 2010;77:974–979.
- Zureick AH, Evans CL, Niemierko A, et al. Left hippocampal dosimetry correlates with visual and verbal memory outcomes in survivors of pediatric brain tumors. Cancer 2018;124:2238–2245.
- Burman C, Kutcher GJ, Emami B, et al. Fitting of normal tissue tolerance data to an analytic function. Int J Radiat Oncol Biol Phys. 1991;21:123–135.
- Emami B, Lyman J, Brown A, et al. Tolerance of normal tissue to therapeutic irradiation. Int J Radiat Oncol Biol Phys. 1991;21:109–122.
- Houweling AC, Philippens ME, Dijkema T, et al. A comparison of dose-response models for the parotid gland in a large group of head-and-neck cancer patients. Int J Radiat Oncol Biol Phys. 2010;76:1259–1265.
- Schneider U, Sumila M, Robotka J. Site-specific dose-response relationships for cancer induction from the combined Japanese A-bomb and Hodgkin cohorts for doses relevant to radiotherapy. Theor Biol Med Model. 2011;8:27.
- King MT, Modlin L, Million L, et al. The parotid gland is an underrecognized organ at risk for craniospinal irradiation. Technol Cancer Res Treat. 2016;15:472–479.
- Teoh M, Clark CH, Wood K, et al. Volumetric modulated arc therapy: a review of current literature and clinical use in practice. Br J Radiol. 2011;84:967–996.
- Ron E, Modan B, Boice JD, et al. Tumors of the brain and nervous system after radiotherapy in childhood. N Engl J Med. 1988;319:1033–1039.
- Brenner DJ, Hall EJ. Cancer risks from CT scans: now we have data, what next? Radiology 2012;265:330–331.
- Pediatric Proton/Photon Consortium Registry (PPCR) 2019 [Internet]. [cited 2019 Mar 1]. Available from: http://www.pedsprotonregistry.org/
- Pixberg C, Koch R, Eich HT, et al. Acute toxicity grade 3 and 4 after irradiation in children and adolescents: results from the IPPARCA collaboration. Int J Radiat Oncol Biol Phys. 2016;94:792–799.
- Grau C, Baumann M, Weber DC. Optimizing clinical research and generating prospective high-quality data in particle therapy in Europe: Introducing the European Particle Therapy Network (EPTN). Radiother Oncol. 2018;128:1–3.
- Pediatric Normal Tissue Effects in the Clinic (PENTEC). 2019. [cited 2019 Jan 3]. Available from: https://www.pentecradiation.org
- Rorvik E, Fjaera LF, Dahle TJ, et al. Exploration and application of phenomenological RBE models for proton therapy. Phys Med Biol. 2018;63:185013.
- Fjæra LF, Li Z, Ytre-Hauge KS, et al. Linear energy transfer distributions in the brainstem depending on tumour location in intensity-modulated proton therapy of paediatric cancer. Acta Oncol. 2017;56:763–768.