Abstract
Background and purpose: A collaborative network between proton therapy (PT) centres in Trento in Italy, Poland, Austria, Czech Republic and Sweden (IPACS) was founded to implement trials and harmonize PT. This is the first report of IPACS with the aim to show the level of harmonization that can be achieved for proton therapy planning of head and neck (sino-nasal) cancer.
Methods: CT-data sets of five patients were included. During several face-to-face and online meetings, a common treatment planning protocol was developed. Each centre used its own treatment planning system (TPS) and planning approach with some restrictions specified in the treatment planning protocol. In addition, volumetric modulated arc therapy (VMAT) photon plans were created.
Results: For CTV1, the average Dmedian was 59.3 ± 2.4 Gy(RBE) for protons and 58.8 ± 2.0 Gy(RBE) for VMAT (aim was 56 Gy(RBE)). For CTV2, the average Dmedian was 71.2 ± 1.0 Gy(RBE) for protons and 70.6 ± 0.4 Gy(RBE) for VMAT (aim was 70 Gy(RBE)). The average D2% for the spinal cord was 25.1 ± 8.5 Gy(RBE) for protons and 47.6 ± 1.4 Gy(RBE) for VMAT. The average D2% for chiasm was 46.5 ± 4.4 Gy(RBE) for protons and 50.8 ± 1.4 Gy(RBE) for VMAT, respectively. Robust evaluation was performed and showed the least robust plans for plans with a low number of beams.
Discussion: In conclusion, several influences on harmonization were identified: adherence/interpretation to/of the protocol, available technology, experience in treatment planning and use of different beam arrangements. In future, all OARs that should be included in the optimization need to be specified in order to further harmonize treatment planning.
Introduction
The first patient treated with proton therapy (PT) in Europe was a recurrent cervical cancer patient irradiated at The Svedberg laboratory in Uppsala, Sweden in 1957 [Citation1]. Since then more than 170 000 patients have been treated with PT [Citation2] due to the advantageous dosimetric properties of protons compared to other forms of ionizing radiation [Citation3,Citation4]. In 1990, the first hospital-based PT centre at Loma Linda University Medical Center started its operation [Citation5]. Today, the number of PT centres is permanently increasing.
Despite rapidly growing number of patients treated with PT, there is still a lack of clinical evidence for PT compared to other modern highly conformal photon therapies [Citation6–10]. The potential benefit of PT compared to the photon therapy techniques is summarized in less incident and exit radiation dose to the normal tissue presumably with reduced risk of dose-dependent late side effects including secondary cancers. For a small number of tumor types like thick posterior ocular melanomas, skull base chordomas and chondrosarcomas, there is evidence for a benefit of PT over photon therapy and for other tumors like e.g., hepatocellular carcinomas, meningiomas and prostate cancer there is evidence for the efficacy of PT but not superiority to photon therapy [Citation11–17]. In pediatric central nervous system (CNS) malignancies, PT appears to be superior to photon therapy but still more data are needed [Citation18–21]. For the majority of other malignancies like head and neck (H&N)-, lung-, breast-, gastronintestinal (GI)-, brain- and pediatric non-CNS malignancies, there is yet no clinical evidence for treatment with PT. This vicious circle with an expansion of PT treatment with no corresponding increase in clinical evidence level should and could be broken by collaborative prospective phase II trials of best available techniques and/or adequately powered collaborative phase III trials reporting agreed endpoints of clinical relevance like e.g., local control, disease free survival, overall survival, quality of life and toxicity. An attractive suggestion by Mark Lodge et al. in 2007 is to set up a proton therapy register in order to gain more data for various tumour types [Citation22]. However, such a collaborative data base or quality register requires solid organization including legislative considerations as well as need a common PT language, common design of proton treatment protocols including targets and organs at risk (OAR) delineation, delivery methods as well as treatment planning methods in order achieve consistent data outcome [Citation23–25]. Moreover, a common prescription and reporting of PT should be achieved.
The IPACS group consisting of physicians, medical physicists and treatment planners is a collaboration between five PT centres in Trento in Italy, Poland, Austria, Czech Republic and Sweden that has the goal of harmonizing PT in all above mentioned aspects, which will allow to draw conclusions from upcoming multicenter studies. This first publication reports on the initial collaborative network focused on one important aspect of our collaboration: how to harmonize modern PT treatment planning of malignancies in the sinonasal region. The aim was to show the harmonization level that can be achieved for PT planning of sinonasal cancer in this PT network. The variety between participating centers, in terms of beam delivery technology setup, treatment planning systems, experience reflects the reality of future multicentre studies for PT.
Material and methods
Participating centre
Four European particle therapy centres initiated the so called PACS collaborative group (acronym for Polish, Austrian, Czech and Swedish) by signing a Memorandum of Understanding during summer 2014. At that time only one centre treated patients and the others were in the setup phase. A PT centre in Trento joined the group at a later stage, forming the current IPACS-group, but was not involved in the initial data collection phase of this work.
A summary of the equipment at the different centres can be seen in . This table shows the variety between the centres and the diversity is very realistic for any future multicentre clinical study.
Table 1. Centre specific information. TPS is an abbreviation for treatment planning system and OIS for oncology information system.*till first submission of manuscript.
Planning protocol harmonization process
After the general agreement between the centres and the finalization of a declaration of intent, an agreement on a treatment planning protocol for PT treatment planning of the sinonasal malignancies has been reached during several on-site meetings and video conferences. Treatment plans were created individually at the four centres for the same five anonymous patient cases according to the predefined protocol. During the following meetings treatment plans and plan parameters were discussed, deviations in the interpretation of the planning protocol were identified and the planning protocol was modified for further harmonization. As a result, re-planning was occasionally necessary. The main discussion points of the treatment planning protocol were related to:
planning aims and prioritization of objectives
strict treatment planning guidelines vs. individual treatment planning approach
robustness evaluation
choice of reported parameters
centralized data evaluation vs. local evaluation
In the following sections, the outcome of those discussions will be presented.
Patient cases and contours
In total, five anonymous patient cases were included. All patients were previously treated at centre 1. The patients were scanned with a GE computed tomography (CT) scanner (Optima CT 580 W) in supine position, immobilized in a thermoplastic mask system with thermoplastic cushions for C spine fixation (no bite-block was used). The slice thickness was 2.5 mm.
Based on the CT data and further information on the medical history, contours were agreed between involved physicians. It has to be stressed that the discussion on contouring or even interobserver variability of contouring is out of scope of this study. The gross tumor volume (GTV) was defined as the primary tumour mass and clinically involved lymph nodes (ILL) visible on CT/MRI fusion. The clinical target volume (CTV) included all areas at risk of subclinical involvement in the vicinity of the GTV as well as elective treatment of lymphatic regions of the neck, if deemed necessary. CTV1 and CTV2 were defined for the initial and the boost part of the head and neck treatment, respectively. The planning target volume (PTV) was defined by the same principles used for photon treatments [Citation26] as a geometric uniform expansion of CTV. The PTV1 was defined using a uniform margin of 5 mm around the CTV1 and PTV2 was defined using a uniform margin of 3 mm around the CTV2. The contoured OARs are listed in . For each patient case, all plans were created based on the same structure set.
Table 2. Priority list for OAR and target structures dose objectives used for treatment planning of sinonasal cancer cases. The first four priority structures and the CTV need to meet also the constraints after robustness evaluation.
Treatment prescriptions and planning approaches
The prescription to CTV1&PTV1 was 56 Gy(RBE)/28 fractions and to CTV2&PTV2 14 Gy(RBE)/7 fractions following the original prescription with which patients were treated. The GTV is part of CTV2 and no separate prescription was applied. All prescribed and reported proton doses within this manuscript are expressed in terms RBE weighted absorbed dose [Citation23] using the 1.1 RBE factor.
The following treatment planning goals were agreed upon. For the nominal plan and all the PTVs, 95% of the prescribed doses supposed to cover at least 98% of the volume, i.e., 53.2 Gy(RBE) for PTV1 and additional 13.3 Gy(RBE) for PTV2. For all the CTVs, 100% of the prescribed doses supposed to cover at least 98% of the volume. Maximum allowed hotspots were 107% in the 2% of the PTVs volumes and should be located only inside the PTVs.
The treatment planners were asked to follow the objectives for the target volumes, however, higher priorities were assigned to some of the OARs. The priority order for OARs and target volumes starting with the highest priority is listed in . The planning aim was to strictly fulfill the objectives (see also ) for the first four highest priority structures also after robustness evaluation.
The plans were created by each centre using their TPS. The plan setup was decided by each centre individually but some restrictions needed to be followed: plans should consist of 2–4 fields with beam angle selections depending on target location, patient geometry and facility dependent conditions. Recommendations that were not explicitly stated but still followed included: no beam should normally point the distal fall-off towards an OAR, entry through metal parts should be strictly avoided and every chosen beam direction should pass through the homogeneous and shortest path possible avoiding CT image artifacts. Artifacts from e.g., dental fillings were differently dealt between the centers, e.g., noncommercial software MDT (ReVision Radiology) for metal artifacts reduction, contouring of artifacts and overriding them with the average HU of the neighboring tissue or artifacts stayed unchanged and a perpendicular beam direction was aimed for. The dose calculation grid size was agreed to be 3 × 3×3 mm (that was the smallest grid size possible for one of the centres due to limitations in memory capacity in the treatment planning computer). The proton plans could be performed by using either gantry or fixed beams, single field optimization (SFO) or multiple field optimization (MFO) planning techniques [Citation27] were allowed depending on the centre’s experience. The treatment plan had to be designed with one treatment plan for each treatment phase; hence, simultaneous treatment in one treatment plan was not accepted.
For each case and each treatment phase, photon VMAT plans for a Varian Clinac iX with 120 leaf collimator (VMS Palo Alto, CA, USA), based on the same CT data, structures, planning aims and objectives, were created by centre 4. For PTV1 either 3–4 full arcs (0°–360°) were used and for PTV2 3–4 arcs were used with beam angles from 90° to 270° degrees for cases 1–4 and 270°–180° for case 5. The nominal beam energy was 6 MV photons in all cases.
Robustness evaluation
We agreed to consider the robustness by evaluating the effect of a 3.5% uncertainty (according to recommendations in [Citation28]) in the CT-calibration curve in combination with rigid 2 mm isocenter shifts in each cardinal directions separately (simulating patient inter-fractional setup variations based on the assumption of daily image guidance and experiences of setup variability in the head and neck region). The choice of evaluation parameters is in accordance with previous studies, e.g., [Citation29]. Changes in patient anatomy or tumor shrinkage were not taken into account.
Evaluation of the dose to CTV and OARs were done by creating 12 perturbed plans from the nominal plan [Citation26,Citation30].
For the first four highest priority structures and the CTV, the treatment planning objectives (see ) had to be met also after robustness evaluation, i.e., in all perturbed plans. The robustness was evaluated for each treatment phase separately. The reason for that was that current treatment planning tools did not allow the combined analysis of several treatment phases at once. Each centre evaluated the robustness of the whole treatment, i.e., the combination of the plans for the two phases manually to guarantee that the objectives for the first four highest priority structures and the CTV should be met also after robustness evaluation of the whole treatment.
Reported parameters
All dose volume parameters for OARs 1–4 and 6–16 defined and listed in , were collected for analysis. The reported parameters for the target volumes (CTV and PTV) were D98%, D2%, Dmedian and V95%.
All parameters were reported for the summed plans and for each treatment phase individually, i.e., the nominal plans. Furthermore, the range of the values for all defined parameters (i.e., the worst and best value, for the 12 perturbed robustness scenario) was reported.
Results
In centre 1 and 4, all treatment plans were made by one treatment planner. At centre 2, case 1–3 were made by one and case 4–5 by another treatment planner and at centre 3 there were three different treatment planners involved in the treatment planning of the five cases. Because of the challenging cases, all centres used the MFO technique. On average, centre 4 and 3 used 4 beams for phase 1 and 3–4 beams for phase 2. As centre 2 did not plan with a gantry and has a comparatively smaller available field size, more beams than four were most often needed for phase 1, on average 5 for phase 1 and 3 for phase 2. For centre 1 on average, 2 beams were used for both phase 1 and 2. Due to the superficially located targets nearly all fields needed a range shifter. Centre 4, 3, 2 and 1 used a 35 mm, 42 mm, 30 mm or 65 mm range shifter, respectively.
Nominal plans – dose–volume parameters for CTV1 and CTV2
Values for the nominal treatment plans are presented for the whole treatment, i.e., the sum of the treatment plans for phase 1 and 2.
For the CTV1 D98% the planning aim was 56 Gy(RBE), which was nearly achieved for most of the plans with an average of 54.6 ± 2.3 Gy(RBE) for protons and 54.9 ± 0.5 Gy(RBE) for VMAT (see ). The average variation of D98% per centre for the proton plans was within 2.0 Gy(RBE). Dmedian were higher for centre 1 for cases 1 and 2 and consistent between centres for the other cases. CTV1 V95% were always above 95% with a mean value of 99.0 ± 1.4% for all cases and centres.
Figure 1. D98% for CTV1 in the first treatment phase and CTV2 in the second treatment phase for the nominal plans and the results of the robustness analysis for all 5 head and neck cases from each centre and the VMAT plan is shown. The black bars show the band width (max and min) of D98% for all the robustness scenarios. The dashed line shows the planning aim for CTV1 and CTV2 D98% which was 56 Gy(RBE) and 14 Gy(RBE), respectively as estimated for the different treatment phases separately (however, the planning aim was always defined for the total treatment, i.e., the sum of the two phases).
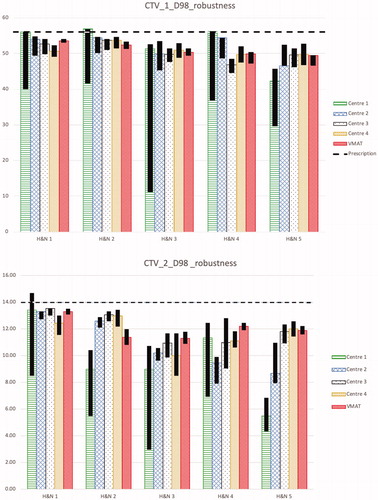
For the CTV2 D98% the planning aim was 70 Gy(RBE), which was not achieved in any plan with an average of 60.8 ± 5.7 Gy(RBE) for protons and 61.6 ± 3.4 Gy(RBE) for VMAT (see ). The low values were mainly due to the conflicting OARs with higher priority close to the target, i.e., brainstem, spinal cord and optical apparatus. The variation of average Dmedian between centres was within 2.0 Gy(RBE) for the proton plans for all cases with an average of 71.1 ± 0.9 Gy(RBE) for protons and 70.6 ± 0.3 Gy(RBE) for VMAT. CTV2 V95% was always lower than V95% for CTV1 and the variation in average CTV2 V95% between centres was up to 2.4% for the proton plans.
For CTV1 and CTV2, VMAT plans showed similar performance compared to proton plans.
Robustness analysis for CTVs
shows the robustness of D98% for CTV1 and for CTV2 for each treatment phase. As expected, photon plans show higher robustness, as they are not influenced dramatically by ±3.5% variations of HU. Larger deviations between centres are seen in the boost phase, i.e., for CTV2. In all but one case (case 5), the worst robustness for CTV1 was reported by centre 1. This might partly be explained by the less number of beams used (2 versus 3–4 for the other centres).
Nominal plans – Dose–volume parameters for OARs
For the D2% of the spinal cord, the better sparing with proton plans compared to photons is obvious (see ). From all cases, centre 1 reported the lowest D2% for the spinal cord. On average, the D2% was 25.1 ± 8.5 Gy(RBE) for protons and 47.6 ± 1.4 Gy(RBE) for VMAT.
Figure 2. D2% for spinal cord and brainstem for the first and second treatment phases separately for the nominal plans and the results of the robustness analysis for all 5 head and neck cases from each centre and the VMAT plan is shown. The black bars show the band width (max and min) of D2% for all the robustness scenarios. The dashed line shows the objective for the total treatment, i.e., for the sum of both treatment phases, which was D2% ≤ 50 Gy(RBE) for the spinal cord and D2% ≤ 54 Gy(RBE) for the brainstem.
For the D2% of the brainstem, the planning aim was achieved in all of the plans (see ). The variation of D2% between the proton plans from the different centres is up to 30 Gy(RBE). On average, the D2% was 38.4 ± 8.3 Gy(RBE) for protons and 47.7 ± 2.3 Gy(RBE) for VMAT.
For the D2% of the optical structures (chiasm, optical nerve left and right (ONL, ONR)), the planning aim to stay below 54 Gy(RBE) was always achieved. On average, the values for chiasm, ONL, ONR were 46.5 ± 4.4 Gy(RBE), 45.3 ± 5.8 Gy(RBE), 46.0 ± 4.4 Gy(RBE) for protons and 50.8 ± 1.4 Gy(RBE), 50.6 ± 1.6 Gy(RBE), 51.8 ± 0.4 Gy(RBE) for VMAT, respectively.
For the cochlea, the objective Dmean < 30 Gy(RBE), despite its priority in and its proximity to the target structures, could be achieved by protons in the majority of the cases and in three of five cases also by VMAT. One exception, where sufficient sparing was not possible was case 5 for centre 2 because of the horizontal beam setup. In this particular case, a clear advantage for a gantry was identified. Centre 3 also reported values exceeding the objective for cochlea for cases 1 and 2. On average, the values for left and right cochlea were 19.6 ± 7.9 Gy(RBE), 19.9 ± 9.3 Gy(RBE) for protons and 29.9 ± 3.7 Gy(RBE), 29.1 ± 3.5 Gy(RBE) for VMAT, respectively.
For the parotid glands, due to their priority in and their proximity to the target structures, the objective of Dmean less than 28 Gy(RBE) was only achieved in one of the parotid glands by four proton plans for cases 3 and 4. Centre 3 reported most often the highest values of Dmean to the parotid glands and centre 2 most often the lowest. On average, the values for left and right parotid gland were 39.5 ± 11.2 Gy(RBE), 40.5 ± 9.8 Gy(RBE) for protons and 42.6 ± 8.7 Gy(RBE), 44.3 ± 6.1 Gy(RBE) for VMAT, respectively.
For larynx, the Dmean objective was achieved for all plans except for two plans from centre 1 and two plans from centre 3. On average, the values for the larynx were 39.4 ± 6.6 Gy(RBE) for protons and 44.6 ± 4.7 Gy(RBE) for VMAT.
Dmean for Body-CTV indicates the integral dose and shows a benefit for PT over VMAT plans. The Dmean reported for the proton plan for case 5 from centre 2 is higher in comparison to the other proton plans. This is probably due to the lack of a gantry in centre 2. Besides that the Body-CTV Dmean was systematically higher for the proton plans from centre 3. The number of beams seem to have no influence on Body-CTV Dmean as centre 1 used usually 2 beams per phase and centre 4 used 3–4 beams per phase with nearly the same outcome. On average, the values for the Dmean of Body-CTV were 9.2 ± 2.7 Gy(RBE) for protons and 14.5 ± 3.1 Gy(RBE) for VMAT.
Robustness analysis for OARs
The objectives of the OARs with higher priorities than target structures, i.e., the spinal cord, brainstem, chiasm and the optic nerves, had to be fulfilled for all scenarios in the robustness analysis for the treatment plan to be acceptable. All plans fulfill this requirement. shows the robustness analysis for D2% for the spinal cord for the two treatment phases separately. The majority of the dose to the spinal cord is delivered in the first phase of the treatment. In all proton cases but one, case 5 from centre 2, the dose to the spinal cord is less than 1 Gy(RBE) in the nominal plan of the second treatment phase. No pattern could be found in the variation of robustness between the centres.
shows the robustness analysis for D2% of the brainstem. Again, VMAT plans with an average D2% range in the robustness analysis for the first and second phase treatment plan of 4.0 and 1.0 Gy(RBE), respectively are more robust than the proton plans with corresponding ranges 13.0 and 1.0 Gy(RBE).
For optical structures, i.e., the chiasm and the optical nerves, the results of the robustness analysis of D2% are similar and shown for the chiasm in . For chiasm, the average D2% range in the robustness analysis for the first and second phase VMAT plans are 1.9 and 1.3 Gy(RBE), respectively and are more robust than the proton plans with corresponding ranges 9.7 and 2.9 Gy(RBE). For all phases systematically the dose to optical structures is most robust for the plans from centre 4.
Figure 3. D2% for chiasm for the first and second treatment phases separately for the nominal plans and the results of the robustness analysis for all 5 head and neck cases from each centre and the VMAT plan is shown. The black bars show the band width (max and min) of D2% for all the robustness scenarios. The dashed line shows the objective for the total treatment, i.e., for the sum of both treatment phases, which was D2% ≤ 54 Gy(RBE).
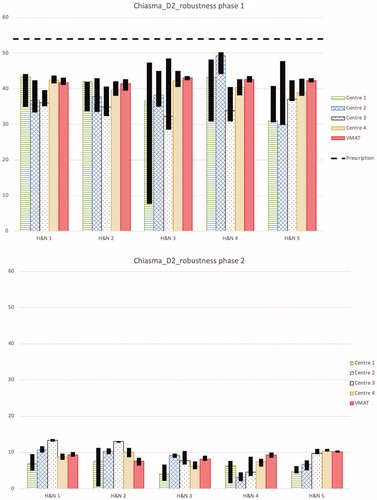
Discussion
Central concepts in hadron therapy, including PT, are equal tumor control, reduced long-term side effects and cost-effectiveness compared to modern photon therapy techniques. These concepts still need to be elucidated for several treatment sites. To work towards common treatment protocols and clinical trials in international multi-center studies a PT network has been formed (IPACS), which aims to harmonize the PT in the participating centres and also to create a solid platform for clinical trials in order to increase the evidence for PT. It should be emphasized, that for the IPACS group both multicentricity and multidisciplinary participation of physicians, medical physicists and treatment planners is essential.
The main aim for this specific study was to show the level of harmonization that can be achieved for PT planning of sinonasal cancer in this PT network, by highlighting differences in the treatment plans despite in detail defined treatment planning protocol. For patient cases considered in this study, the planning aims for the CTV1 volumes were obtained for all treatment plans. The planning aims for CTV2 were not obtained in any of the plans, due to higher prioritization of OARs adjacent to the tumour. For those OARs, the constraints were much lower than the dose prescribed to those target volumes. The variation in CTV D98% between centres was therefore larger for the high dose volume CTV2 compared to CTV1. Photon plans showed similar target volume coverage and performance to proton plans.
For OARs with higher priorities than target structures, i.e., optical structures, brainstem and spinal cord, the objectives were fulfilled in all cases. It was agreed that the planning objectives for those structures had to be fulfilled also in all robust analysis scenarios. One can argue that it is unlikely that all treatment fractions will be delivered according to one specific scenario, instead combined systematic and random variation is expected. This means that it might not be necessary to force the planning objectives to be fulfilled for all robustness scenarios. Studies on this issue have recently been published by Malyapa et al. [Citation31] and McGowan et al. [Citation32]. However, this issue was vastly discussed within IPACS group and the consensus was to be on the safe side. In all cases, the necessity to obtain those objectives made it impossible to fulfill the planning aims for the CTV2 even without taking robustness into account. The fact that the dose to those OARs varied between centres might be explained by different beam directions and optimization strategies. Similarly, for other OARs the choice of the beam arrangement, could also have an influence on the dose variation between the centres. Based on that conclusions, for future studies suggestion for the beam arrangement could be included in the treatment planning protocol, for further harmonization of treatments.
OAR dose variations were observed between proton and VMAT treatment plans. For all OARs with higher prioritization of objectives, except the spinal cord, the value of D2% were similar in both proton and VMAT treatment plans. For the spinal cord, the VMAT plans systematically had higher D2%. When comparing proton and VMAT treatment plans due to smaller low and mid dose regions OARs further away from the target volume are more spared for the proton treatment compared to OARs closer to the target volume where the differences most often are smaller. This is because the low dose bath region is expected to be smaller for protons due to less beam entries and the sharp dose fall off in the distal part of the beam. This is clearly seen in the value of Dmean for the structure Body-CTV.
The photon treatment plans were, as expected, more robust than proton treatment plans. This is true to a large extent for the CTVs but also for the OARs. The distal penumbra of a proton beam compared to the exponential decay in the beam direction for photons is influencing plan robustness. Furthermore, the fact that a proton beam is more sensitive to geometrical changes and density variations along the beam path compared to a photon beam, will result in less robust proton plans comparing to photons. Sometimes the ‘unrobustness’ of a proton plan outweighs the advantage of avoidance compared to VMAT. Robustness analysis for OARs and a two phase treatment is challenging, as it is partially influenced by the dose given to the OAR in each individual treatment phase. At the time of this work, none of the TPSs included in the study could offer a feasibility of robustness analyses in a two phase treatment simultaneously.
It was observed that centre 3 systematically reported higher doses to cochlea, parotid glands and larynx. It might be connected to the fact that lower prioritized OARs were given either low priorities in the treatment plan optimization procedure or were completely excluded from the optimization process. This was not the case for the other centres. This fact can also be related to the systematically higher values of Body-CTV Dmean reported from centre 3. The relatively small variation in target coverage between the centres indicates that even if more OARs are included in the plan optimization process, the target coverage might not be affected. To harmonize the treatment planning further, it could be stated more clearly in the treatment planning protocol, which OARs should be chosen for optimization. Another difference between centres was that the robustness was in most cases worse for the treatment plans from centre 1. This might be explained by the use of fewer beam entries in the plan design from that centre, which would mean that the robustness can be somewhat steered by the restriction in number of beam entries in the treatment plan, that should also be further highlighted in the treatment planning protocol.
In this study, effort has been made to harmonize treatment planning in a realistic multicentre scenario including the existing differences between centres. The influence of differences caused by, for example, treatment planning optimization algorithms or technology used for beam delivery is beyond the scope of this study. No statistical analysis was performed due to the limited number of cases included in the study. The difference in biological effect and the clinical relevance of the differences in a context of a multi-institutional study still needs to be investigated.
Conclusion
In conclusion, our study showed the possible harmonization of sinonasal cancer treatment planning for proton therapy in a multicentre scenario. Influences on the harmonization were identified as follows: adherence/interpretation to/of the protocol, available technology at the participating centre, experience in treatment planning and use of different beam arrangements. During the creation of the treatment planning protocol great emphasis was placed on robustness considerations and PTV, interpretation of robustness in a sequential boost setting, prioritization of OARs sparing vs target coverage, especially for OARs prioritised lower than the targets and inclusion/exclusion of different planning parameters. For excluding different parameters in the treatment planning protocol the main decision was, that it is up to the local policy of each centre to select beam angles, spot spacing, layer spacing, optimization method, use of range shifter and air gap as well as use of beam specific targets.
Disclosure statement
No potential conflict of interest was reported by the authors.
References
- Falkmer S, Fors B, Larsson B, et al. Pilot study on proton irradiation of human carcinoma. Acta Radiol. 1962;58:33–51.
- PTCOG. http://www.ptcog.ch/; 2016.
- Munzenrider JE, Austin-Seymour M, Blitzer PJ, et al. Proton therapy at Harvard. Strahlentherapie. 1985;161:756–763.
- Wilson RR. Radiological use of fast protons. Radiology. 1946;47:487–491.
- Slater JM, Archambeau JO, Miller DW, et al. The proton treatment center at Loma Linda University Medical Center: rationale for and description of its development. Int J Radiat Oncol Biol Phys. 1992;22:383–389.
- Miller RC, Lodge M, Murad MH, et al. Controversies in clinical trials in proton radiotherapy: the present and the future. Semin Radiat Oncol. 2013;23:127–133.
- Allen AM, Pawlicki T, Dong L, et al. An evidence based review of proton beam therapy: the report of ASTRO’s emerging technology committee. Radiother Oncol. 2012;103:8–11.
- Brada M, Pijls-Johannesma M, De Ruysscher D. Current clinical evidence for proton therapy. Cancer J. 2009;15:319–324.
- Combs SE, Laperriere N, Brada M. Clinical controversies: proton radiation therapy for brain and skull base tumors. Semin Radiat Oncol. 2013;23:120–126.
- Olsen DR, Bruland ØS, Frykholm G, et al. Proton therapy – a systematic review of clinical effectiveness. Radiother Oncol. 2007;83:123–132.
- Benk V, Liebsch NJ, Munzenrider JE, et al. Base of skull and cervical spine chordomas in children treated by high-dose irradiation. Int J Radiat Oncol Biol Phys. 1995;31:577–581.
- Dendale R, Lumbroso-Le Rouic L, Noel G, et al. Proton beam radiotherapy for uveal melanoma: results of Curie Institut-Orsay Proton Therapy Center (ICPO). Int J Radiat Oncol Biol Phys. 2006;65:780–787.
- Mouw KW, Trofimov A, Zietman AL, et al. Clinical controversies: proton therapy for prostate cancer. Semin Radiat Oncol. 2013;23:109–114.
- Rosenberg AE, Nielsen GP, Keel SB, et al. Chondrosarcoma of the base of the skull: a clinicopathologic study of 200 cases with emphasis on its distinction from chordoma. Am J Surg Pathol. 1999;23:1370–1378.
- Slater JD, Rossi CJ, Yonemoto LT, et al. Proton therapy for prostate cancer: the initial Loma Linda University experience. Int J Radiat Oncol Biol Phys. 2004;59:348–352.
- Weber DC, Trofimov AV, Delaney TF, et al. A treatment planning comparison of intensity modulated photon and proton therapy for paraspinal sarcomas. Int J Radiat Oncol Biol Phys. 2004;58:1596–1606.
- Wenkel E, Thornton AF, Finkelstein D, et al. Benign meningioma: partially resected, biopsied, and recurrent intracranial tumors treated with combined proton and photon radiotherapy. Int J Radiat Oncol Biol Phys. 2000;48:1363–1370.
- Archambeau JO, Slater JD, Slater JM, et al. Role for proton beam irradiation in treatment of pediatric CNS malignancies. Int J Radiat Oncol Biol Phys. 1992;22:287–294.
- Fuss M, Hug EB, Schaefer RA, et al. Proton radiation therapy (prt) for pediatric optic pathway gliomas: comparison with 3d planned conventional photons and a standard photon technique. Int J Radiat Oncol Biol Phys. 1999;45:1117–1126.
- Lin R, Hug EB, Schaefer RA, et al. Conformal proton radiation therapy of the posterior fossa: a study comparing protons with three-dimensional planned photons in limiting dose to auditory structures. Int J Radiat Oncol Biol Phys. 2000;48:1219–1226.
- Moteabbed M, Yock TI, Paganetti H. The risk of radiation-induced second cancers in the high to medium dose region: a comparison between passive and scanned proton therapy, IMRT and VMAT for pediatric patients with brain tumors. Phys Med Biol. 2014;59:2883–2899.
- Lodge M, Pijls-Johannesma M, Stirk L, et al. A systematic literature review of the clinical and cost-effectiveness of hadron therapy in cancer. Radiother Oncol. 2007;83:110–122.
- ICRU Report Committee IR. ICRU Report 78 prescribing, recording, and reporting proton-beam therapy. J ICRU. 2007;7;1-210.
- Nilsson P, Ceberg C, Kjellén E, et al. A template for writing radiotherapy protocols. Acta Oncol. 2015;54:275–279.
- Santanam L, Hurkmans C, Mutic S, et al. Standardizing naming conventions in radiation oncology. Int J Radiat Oncol Biol Phys. 2012;83:1344–1349.
- DeLuca PM, Seltzer SM. ICRU Report 83. ICRU Rep 83. 2008;8(2):1–98.
- Quan EM, Liu W, Wu R, et al. Preliminary evaluation of multifield and single‐field optimization for the treatment planning of spot‐scanning proton therapy of head and neck cancer. Med Phys. 2013;40:81709.
- Lomax AJ. Intensity modulated proton therapy and its sensitivity to treatment uncertainties 1: the potential effects of calculational uncertainties. Phys Med Biol. 2008;53:1027.
- Holliday E, Bhattasali O, Kies MS, et al. Postoperative intensity-modulated proton therapy for head and neck adenoid cystic carcinoma. Int J Part Ther. 2016;2:533–543.
- Paganetti H. Range uncertainties in proton therapy and the role of Monte Carlo simulations. Phys Med Biol. 2012;57:R99–117.
- Malyapa R, Lowe M, Bolsi A, et al. Evaluation of robustness to setup and range uncertainties for head and neck patients treated with pencil beam scanning proton therapy. Int J Radiat Oncol Biol Phys. 2016;95:154–162.
- McGowan SE, Albertini F, Thomas SJ, et al. Defining robustness protocols: a method to include and evaluate robustness in clinical plans. Phys Med Biol. 2015;60:2671–2684.