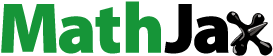
Introduction
Stereotactic body radiation therapy (SBRT) is an effective treatment procedure allowing the delivery of high radiation doses in a small number of fractions to produce a high biological effective dose (BED) [Citation1]. To minimize the normal tissue toxicity, the delivered doses are strictly conformed to the target, with a rapid dose falloff away from the tumor; therefore, the administration of SBRT requires a high accuracy of dose delivery to the tumor.
The overall accuracy of dose delivery can be compromised by tumor motion [Citation2], which must be accounted for during simulation, planning, and delivery. 4DCT has been used as part of the simulation process to evaluate the extent of tumor motion and to derive an internal target volume (ITV) for lung cancer [Citation3,Citation4]. However, it has been shown that 4DCT may fail to accurately estimate the tumor motion due to a number of factors, such as irregular breathing, short acquisition time, and/or anatomical changes between simulation and treatment [Citation5,Citation6]. Steiner et al. reported that 4DCT underpredicted lung target motion by factors of 1.7, 1.9 and 1.7 in the superior–interior (SI), left–right (LR) and anterior–posterior (AP) directions [Citation7].
Tumor motion is complicated; its amplitudes and patterns are dependent on tumor location [Citation8]. Schmidt et al. [Citation9] pointed out that the treatment sometimes involves both a primary tumor and involved LN targets, but their motion patterns are different, and individual evaluations are needed. Michalski et al. [Citation10] evaluated the relationship between tumor size and tumor motion amplitude, but no correlation was found. Furthermore, tumor motion amplitude variability and baseline shift have also been evaluated, typically by additional 4DCT and 3D/4D CBCT [Citation11,Citation12]. In recent years, clinical implementation of real-time tumor localization has gained increasing attention [Citation13–15], as it may provide tumor motion data that is closer to real-world situations.
In this study, our primary goal was to investigate tumor motion amplitudes and variations over the entire course of treatment by analyzing a large set of real-time tumor motion data extracted from the logfiles generated by the Synchrony Respiratory Tracking System (Accuray, Inc., Sunnyvale, CA). Our second goal is to evaluate the reliability of using 4DCT to represent tumor motions and treatment margins at different tumor locations. We believe that the results will provide a useful framework for us to determine PTV expansion in lung stereotactic treatment for both CyberKnife (Accuray, Inc., Sunnyvale, CA) and LINAC.
Material and methods
Patients
Between April 2017 and August 2018, 83 patients (55 men, 28 women), comprising 86 peripheral lung lesions treated over a total of 428 fractions, were included in this study. All patients were treated with a CyberKnife and lung-optimized treatment (LOT) module; the mean (thereafter represented by mean ± 1SD) treatment time was 41.4 ± 6.6 min The group consisted of 53 primary lung lesions and 30 lung metastasis lesions. The mean prescription dose was 46.5 ± 8.7 Gy over 4.8 ± 1.4 fractions.
Because Knybel et al. [Citation16] did not observe a significant difference between the mean motion amplitudes in the left and right lungs, in this study, all lesions were grouped by upper, middle and lower lobes, which included 51, 4 and 31 lesions, respectively. As our sample included only 4 middle lobes, we divided the patients into two groups, the upper-middle-lobe group (group A) and the lower-lobe group (group B).
In our clinical protocol, the patients were placed in the supine position with their arms along their sides and were immobilized with a customized vacuum body mold. A breath-hold simulation CT scan (1.5 mm slice thickness) and a pretreatment 4DCT with 2-mm slice thickness were acquired for all lung SBRT patients. A 3–5 mm isotropic margin was used to generate the PTV.
Tumor motion evaluation
An in-house program was developed to extract the real-time target position data from the log files for analysis, which contained information on the target position derived from orthogonal X-ray images and the target position trajectory derived from the output from correlation and prediction models [Citation17–19].
The logfile-based target position trajectory and a moving average of 1560 points (the number of sample points approximately 1 min, with a sampling rate of 26 Hz) were used to establish the baseline [Citation20]. The baseline shift was defined as the maximum change along the vertical position on the baseline in a specific duration.
Three types of tumor motion amplitudes (TMAorg, TMAbs and TMA4D) were obtained from the original target position trajectory, the baseline-corrected target position trajectory and the 4DCT, respectively. For calculating TMAorg, the cumulative distribution function (CDF) of the original target positions trajectory was derived, and the range corresponding to the probabilities of 5% and 95% was calculated to obtain the TMAorg [Citation21] (Supplementary Figure S1(a)). TMAbs was defined as the same way as TMAorg, but using the baseline-corrected target positions trajectory. TMA4D was defined as the maximum distance (based upon the center of mass) between different breathing phases.
Figure 1. The differences in TMAs in the SI direction for each fraction and patient: (a) the amplitude difference between TMAorg and TMA4D and (b) the amplitude difference between TMAbs and TMA4D. The error bars are the SDs of amplitude difference over fractions for a patient.
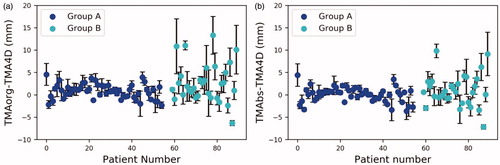
The intrafraction tumor motion amplitude variability (TMV) was defined as the standard deviation (SD) of the peak-to-peak distances of all breathing cycles based upon the baseline-corrected target position trajectory within a single fraction (Supplementary Figure S1(b)). The peak-to-peak distance was defined as the distance of each inhale peak to the next exhale peak. The interfraction TMV was defined as the SD of the TMAbs between fractions.
Margin evaluation
The ITV-to-PTV margin was determined for patients for whom real-time tumor localization was unavailable. In the original formulation by van Herk et al. [Citation22], the margin (M) was calculated using the equation below, which assumes 95% isodose coverage and a 90% probability level.
where ∑ represents the SD of the systematic error and σ represents the SD of the random error. In lung SBRT, due to the broader penumbra and sharp falloff,
and β were selected to be 6.4 mm and 0.84 [Citation18,Citation23], respectively.
In this study, the PTV takes into account the following components: the accuracy of TMA4D representing the tumor motion, the intrafractional TMV and the baseline shift. Furthermore, we hypothesized two scenarios with respect to the baseline corrected or not. For some monitoring systems, the baseline shifts could be measured and corrected by adjusting the treatment couch [Citation24].
For no baseline corrected scenarios, the ∑ and σ are obtained using the equations below:
The systemic and random baseline shifts were evaluated over 10, 20 and 30 mins after treatments because these time points cover the treatment duration in most treatments. For the baseline-corrected scenario, the component of baseline shift was excluded.
Statistical analysis
Statistical analysis was performed using SPSS software (version 21). Pearson's correlation analysis was used to show the dependence of mean TMAbs on TMVs and baseline shift. The Wilcoxon signed-rank test was used to determine whether TMAorg (or TMAbs) and TMA4D were significantly different.
Results
Tumor motion amplitude
The mean TMAorg, TMAbs and TMA4D for the two groups are listed in Supplementary Table T1. In the SI direction, the mean TMAorg was significantly larger than the mean TMA4D in both groups (p < .05). When comparing the mean TMAbs with the mean TMA4D in the SI direction, no significant differences were observed by the Wilcoxon signed-rank test (group A: p = .446; group B: p = .491). The mean TMAorg–TMA4D for groups A and B were 0.8 ± 1.6 and 2.7 ± 4.3 mm, respectively. The mean TMAbs–TMA4D for groups A and B were 0.1 ± 1.6 and 0.9 ± 3.5 mm, respectively. However, large deviations of TMAbs–TMA4D can be observed in most patients in group B, ranging from –7.2 to 9.8 mm in the SI direction ().
Table 1. The PTV margins calculated for the two groups in the SI, LR and AP directions.
TMV
The intra- and interfraction TMVs for the two groups are listed in Supplementary Table T2. The mean intrafraction TMVs over all fractions in the SI direction are 0.7 ± 0.6 and 2.7 ± 1.9 mm for groups A and B, respectively. The mean interfraction TMVs over all fractions in the SI direction were 0.8 ± 0.6 and 1.7 ± 1.0 mm for groups A and B, respectively. A strong correlation was observed in Pearson's correlation analysis between the intrafraction TMV and TMAbs (r = 0.936, p < 0.001) and between the interfraction TMV and TMAbs (r = 0.721, p < .001) (Supplementary Figure S2).
Baseline shift
Time-trend increases in baseline shift over all fractions for both groups are shown in Supplementary Figure S3. A larger baseline shift in the SI direction in group B compared to group A can be observed. A positive correlation (r = .784, p < .001) between mean baseline shift (within 30 min) and mean TMAbs over fractions is shown in Supplementary Figure S4, indicating that the baseline shift increased as the TMAbs increased.
Margin determination
The system and random errors for different components are summarized in Supplementary Table T3. The PTV margin results are listed in . For group A, no margin larger than 5.0 mm was observed in all directions and for all scenarios. However, for group B, SI was the predominant direction for margin expansion and was much larger than that for group A. In the SI direction, a 9.6 mm margin was needed to compensate the accuracy of TMA4D representing the tumor motion and the intrafractional TMV; after taking the baseline shift into account, the margin might increase up to 13.9 mm.
Discussion
At our institution, only 55% of lung SBRT patients are administered LOT by CyberKnife. For the remaining 45% of patients with small lesions (<15 mm) or tumors obstructed by other organs, the use of LOT is not available. For these patients, the SPINE tracking method is used for alignment [Citation25,Citation26], and a relatively large margin is added to the ITV to compensate for tumor motion variation. In this circumstance, it is desirable to have a framework to quantitatively study TMAs and TMVs over the entire course of treatment.
In this study, the tumor motion amplitude, variability and baseline shift from fraction to fraction were evaluated. Our data showed that the TMAorg was significantly larger than predicted in 4DCT. This result is consistent with the work of Steiner et al. [Citation7], in which lung tumor position was monitored using Calypso transponder beacons implanted in patients. Our data also demonstrated that if the baseline shift can be corrected by treatment couch or other facilities, the tumor motion range is comparable to that decided by 4DCT. Nevertheless, when we evaluate the data from individual patients, noticeable differences remain between TMAbs and TMA4D in a number of cases, especially in lower-lobe cases, which are likely caused by larger tumor motion and variation.
This study also reveals that the TMVs and the baseline shift occur primarily along the SI direction and increase with the increase in tumor motion range. The results indicated that the PTV margin added to the ITV should be evaluated based on tumor location. Additionally, the baseline shift has a time trend and increases as time progresses and can become a non-negligible factor for treatments with long time durations, such as CyberKnife or using gating techniques. Therefore, the baseline shift should also be included in the PTV.
For the upper-middle-lobe cases, our results indicate that an isotropic margin no larger than 5.0 mm is recommended, but the findings are limited by the number of middle-lobe cases which bias our results to some extent. In contrast, the margin for the lower-lobe cases shows strong asymmetry. In the SI direction, the margin increases from 9.6 mm (baseline-corrected) to 13.9 mm (30 min free-breathing treatment), in good agreement with Dhont et al. [Citation27] (∼13.7 mm, but no treatment time reported). For this scenario, using SPINE tracking methods to treat patients with a large tumor motion amplitude is not recommended, and fiducial tracking should be deployed.
Conclusions
We assessed tumor motion variability and PTV margins by groups using real-time monitoring for lung SBRT. Significant intra- and interfraction TMV and baseline shifts can be observed in lung cancer patients, especially for lower-lobe lung cases. Tumor location and treatment time are factors that impact the determination of the ITV-to-PTV margin.
Supplemental Material
Download MS Word (227.5 KB)Disclosure statement
All authors state that they have no conflicts of interest.
Additional information
Funding
References
- Benedict SH, Yenice KM, Followill D, et al. Stereotactic body radiation therapy: the report of AAPM Task Group 101. Med Phys. 2010;37:4078–4101.
- Nioutsikou E, Richard N-T, Bedford JL, et al. Quantifying the effect of respiratory motion on lung tumour dosimetry with the aid of a breathing phantom with deforming lungs. Phys Med Biol. 2006;51:3359–3374.
- Keall PJ, Mageras GS, Balter JM, et al. The management of respiratory motion in radiation oncology report of AAPM Task Group 76. Med Phys. 2006;33:3874–3900.
- Underberg RW, Lagerwaard FJ, Cuijpers JP, et al. Four-dimensional CT scans for treatment planning in stereotactic radiotherapy for stage I lung cancer. Int J Radiat Oncol Biol Phys. 2004;60:1283–1290.
- Harada K, Katoh N, Suzuki R, et al. Evaluation of the motion of lung tumors during stereotactic body radiation therapy (SBRT) with four-dimensional computed tomography (4DCT) using real-time tumor-tracking radiotherapy system (RTRT). Phys Med. 2016;32:305–311.
- Cai J, Read PW, Sheng K. The effect of respiratory motion variability and tumor size on the accuracy of average intensity projection from four-dimensional computed tomography: an investigation based on dynamic MRI. Med Phys. 2008;35:4974–4981.
- Steiner E, Shieh C-C, Caillet V, et al. Both four-dimensional computed tomography and four-dimensional cone beam computed tomography under-predict lung target motion during radiotherapy. Radiother Oncol. 2019;135:65–73.
- Seppenwoolde Y, Shirato H, Kitamura K, et al. Precise and real-time measurement of 3D tumor motion in lung due to breathing and heartbeat, measured during radiotherapy. Int J Radiat Oncol Biol Phys. 2002;53:822–834.
- Schmidt ML, Hoffmann L, Moller DS, et al. Systematic intrafraction shifts of mediastinal lymph node targets between setup imaging and radiation treatment delivery in lung cancer patients. Radiother Oncol J Eur Soc Ther Radiol Oncol. 2018;126:318–324.
- Michalski D, Sontag M, Li F, et al. Four-dimensional computed tomography-based interfractional reproducibility study of lung tumor intrafractional motion. Int J Radiat Oncol Biol Phys. 2008;71:714–724.
- Shah C, Grills IS, Kestin LL, et al. Intrafraction variation of mean tumor position during image-guided hypofractionated stereotactic body radiotherapy for lung cancer. Int J Radiat Oncol Biol Phys. 2012;82:1636–1641.
- Bissonnette JP, Franks KN, Purdie TG, et al. Quantifying interfraction and intrafraction tumor motion in lung stereotactic body radiotherapy using respiration-correlated cone beam computed tomography. Int J Radiat Oncol Biol Phys. 2009;75:688–695.
- Bertholet J, Toftegaard J, Hansen R, et al. Automatic online and real-time tumour motion monitoring during stereotactic liver treatments on a conventional linac by combined optical and sparse monoscopic imaging with kilovoltage X-rays (COSMIK). Phys Med Biol. 2018;63:055012.
- Grimwood A, McNair HA, O'Shea TP, et al. In vivo validation of Elekta's clarity autoscan for ultrasound-based intrafraction motion estimation of the prostate during radiation therapy. Int J Radiat Oncol Biol Phys. 2018;102:912–921.
- Keall PJ, Nguyen DT, O'Brien R, et al. The first clinical implementation of real-time image-guided adaptive radiotherapy using a standard linear accelerator. Radiother Oncol J Eur Soc Ther Radiol Oncol. 2018;127:6–11.
- Knybel L, Cvek J, Molenda L, et al. Analysis of lung tumor motion in a large sample: patterns and factors influencing precise delineation of internal target volume. Int J Radiat Oncol Biol Phys. 2016;96:751–758.
- Chan M, Grehn M, Cremers F, et al. Dosimetric implications of residual tracking errors during robotic SBRT of liver metastases. Int J Radiat Oncol Biol Phys. 2017;97:839–848.
- Descovich M, McGuinness C, Kannarunimit D, et al. Comparison between target margins derived from 4DCT scans and real-time tumor motion tracking: insights from lung tumor patients treated with robotic radiosurgery. Med Phys. 2015;42:1280–1287.
- Pepin EW, Wu H, Zhang Y, et al. Correlation and prediction uncertainties in the cyberknife synchrony respiratory tracking system. Med Phys. 2011;38:4036–4044.
- Jensen CA, Acosta Roa AM, Lund JA, et al. Intrafractional baseline drift during free breathing breast cancer radiation therapy. Acta Oncol. 2017;56:867–873.
- Liang Z, Liu H, Xue J, et al. Evaluation of the intra- and interfractional tumor motion and variability by fiducial-based real-time tracking in liver stereotactic body radiation therapy. J Appl Clin Med Phys. 2018;19:94–100.
- Van HM, Remeijer P, Rasch C, et al. The probability of correct target dosage: dose-population histograms for deriving treatment margins in radiotherapy. Int J Radiat Oncol Biol Phys. 2000;47:1121.
- Malinowski K, McAvoy TJ, George R, et al. Incidence of changes in respiration-induced tumor motion and its relationship with respiratory surrogates during individual treatment fractions. Int J Radiat Oncol Biol Phys. 2012;82:1665–1673.
- McNamara JE, Regmi R, Michael Lovelock D, et al. Toward correcting drift in target position during radiotherapy via computer-controlled couch adjustments on a programmable Linac. Med Phys. 2013;40:051719.
- Gerszten PC, Burton SA. Clinical assessment of stereotactic IGRT: spinal radiosurgery. Med Dosim. 2008;33:107–116.
- Ho AK, Fu D, Cotrutz C, et al. A study of the accuracy of cyberknife spinal radiosurgery using skeletal structure tracking. Neurosurgery. 2007;60:ONS147–ONS156.
- Dhont J, Vandemeulebroucke J, Burghelea M, et al. The long- and short-term variability of breathing induced tumor motion in lung and liver over the course of a radiotherapy treatment. Radiother Oncol J Eur Soc Ther Radiol Oncol. 2018;126:339–346.