Abstract
Background: The SIOPE-Brain Tumor Group recently published a guideline on craniospinal target volume delineation for highly conformal radiotherapy. In order to spare critical structures like e.g., the lens or cochlea, highly conformal techniques can underdose the cerebrospinal fluid (CSF) in the dural reflections around cranial and sacral nerves. The purpose of this study is to generate evidence for CSF extension within the dural sheaths of the cranial and sacral nerves in order to improve accuracy in target volume delineation.
Material and methods: Ten healthy volunteers, age 21 till 41 years, underwent an MRI-scan of the skull-base and sacral plexus. To evaluate CSF extension, cT2-weighted images with fat suppression, low signal to noise ratio and little to no motion-related artifacts were used. Two observers measured the extension of CSF from the inner table of the skull for the cranial nerves, and outside the spinal canal for the sacral nerves.
Results: CSF extension (mean distance [95% CI]) was visible within the dural sheaths surrounding the majority of the cranial nerves: optic nerve (40 mm [38–42]), trigeminal nerve (16 mm [15–19]), facial-vestibulocochlear nerve (11 mm [11–12]), glossopharyngeal-vagus-accessory nerve (7 mm [7–9]) and hypoglossal nerve (8 mm [7–9]). No CSF was observed outside the spinal canal at sacral level. No significant difference between both observers was measured.
Conclusion: This study generates evidence for significant CSF extension outside the inner table of the skull. Despite the vicinity of the lens and cochlea, we therefore recommend the inclusion of both optic nerves and internal auditory canals in the clinical target volume for craniospinal irradiation when using highly conformal delivery techniques.
Introduction
Craniospinal irradiation (CSI) plays an important role in the treatment of patients with medulloblastoma and some other rare tumors with leptomeningeal spread, such as germ-cell tumors, atypical teratoid rhabdoid tumors and ependymomas [Citation1].
With the advent of new highly conformal delivery techniques for irradiation of the craniospinal axis, like intensity-modulated radiation therapy (IMRT), volumetric modulated arc therapy (VMAT), TomoTherapy and proton pencil beam scanning (PBS), superior dose distributions within the target volumes with less dose to the organs at risk (OARs) can be achieved [Citation2,Citation3]. Although adequately encompassed by conventional field-based techniques, highly conformal CSI techniques may underdose meninges and CSF in the dural reflections of the cranial and spinal nerves, unless these structures are specifically included in the clinical target volume (CTV) [Citation4,Citation5]. There is already evidence that inadequate coverage of the cribriform plate, temporal lobes, and the inferior aspect of the thecal sac can lead to an increased risk of recurrence after CSI [Citation6–9]. To improve consistency of craniospinal target volume delineation, experts from the SIOPE-Brain Tumor Group (BTG) recently wrote a consensus guideline on craniospinal target volume delineation [Citation1]. However, little evidence is available on the CSF distribution around the cranial and sacral nerve roots, with their individual dural sheaths. A recent study from Noble et al. investigated the CSF extension for the nerves VII-XII, but did not assess the nerves I-VI and the sacral nerves [Citation10].
The purpose of the current study was to generate evidence for the delineation of CSF extension within the dural sheaths of the cranial and sacral nerves in order to improve accuracy in target volume delineation for highly conformal CSI.
Material and methods
Study population
Ten healthy volunteers were asked to participate in this study. All candidates provided written informed consent before participation. Approval for the study was obtained from the University Medical Center Utrecht, Research Ethics Committee (NL53099.041.15).
Procedure
Healthy, adult volunteers underwent an MRI (Philips, 1.5 Tesla) scan of the skull-base and sacral plexus within the radiotherapy department of the University Medical Center Utrecht. The scans were generated in supine position on a soft Philips yellow MRI long mattress with knee support, with the neck in neutral position. For the imaging of the brain and sacral plexus a Philips dStream Headspine coil and a dStream WholeBody (posterior) coil were used, respectively. To evaluate the extension of the CSF, coronally acquired 3 D T2-weighted TSE images with fat suppression, high signal to noise ratio and little to no motion-related artifacts were used. On these images, CSF is rendered white and surrounding tissue gray.
Measurements
For all cranial nerves the extension of the CSF within the dural sheath was measured for the left and right side, thereby analyzing four measurements per nerve pair in total. The extension was noted in millimeter from the inner table of the skull. For the sacral nerves the extension of the CSF outside the spinal canal was determined. AW and GJ measured extension of the CSF for all scans.
Statistical analyses
Statistical analyses for the mean value, 95% confidence interval (CI) and interobserver variability for the CSF distribution in the dural sheaths around the cranial nerves were performed using SPSS Statistics 24 [Citation11]. For all cranial nerves with visible CSF in the dural sheaths, interobserver variability was compared. A descriptive frequency analysis was used to determine the distribution of the data. A Wilcoxon signed-rank test was performed to determine whether there was a significant difference between the observers' assessment of the liquor distribution around the cranial nerves.
Results
Ten healthy volunteers, age 21 till 41 years, four men and six women participated in this study.
Cranial nerves
CSF distribution was only observed in the dural sheaths surrounding the optic (II), trigeminal (V), facial (VII), vestibulo-cochlear (VIII), glossopharyngeal (IX), vagus (X), accessory (XI) and hypoglossal nerves (XII). For the optic nerves the CSF extended up to the posterior aspect of the eyeball in all scans, mean extension 40 mm (95% CI 38–42; ). Mean extension of the CSF around n. V, was 16 mm (95% CI 15–19; ). CSF in the internal acoustic canal, encompassing n. VII and n. VIII, was observed up to a mean distance of 11 mm (95% CI 11–12; ). In the jugular foramen, where the n. IX, n. X and n. XI are located, the mean CSF flow beyond the inner table of the skull is 7 mm (95% CI 7–9; ). CSF around the n. XII was visible in 8 out of the 20 MRI-images only and was observed at a mean distance of 8 mm (95% CI 7–9; ). A summary per cranial nerve is given in .
Figure 1. Extension of the CSF within the dural sheaths of the optic nerve (A), trigeminal nerve (B), facial and vestibulo-cochlear nerves (C), the glossopharyngeal, vagus and accessory nerves (D) the hypoglossal nerve (E) and at spinal levels L5/S1 (F) and S1/S2 (G). Images in the left column are acquired using a coronal 3D T2 TSE with a TR/TE = 8000/1094 ms, acquired resolution of 0.75 × 1.5 × 0.75 mm3 and reconstructed to 0.75 × 0.75 × 0.75 mm3 with SPIR fat suppression. The MR images in the right column are acquired using a coronal 3D T2 TSE with TR/TE = 1800/171 ms, acquired resolution of 2.0 × 2.0 × 2.0 mm3 and reconstructed to 1.0 × 1.0 × 1.0 mm3 with SPAIR fat suppression.
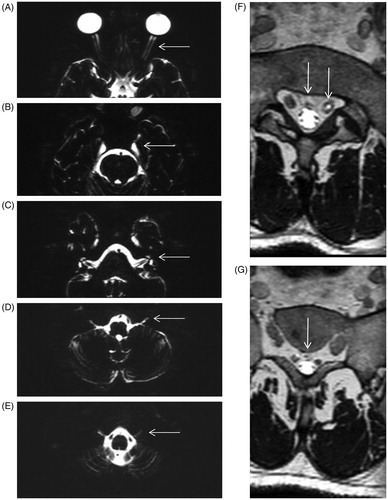
Table 1. CSF extension within the dural sheath of the cranial nerves, measured from the inner table of the skull.
Sacral nerves
Although CSF was observed around the sacral nerve roots, no CSF spread was found outside the spinal canal at sacral level. The lower limit of the spinal subarachnoid space is at the lower limit of the thecal sac, this is mostly at the bottom of the second sacral vertebra (S2). demonstrate the respective CSF distributions at L5/S1 and S1/S2 level in one patient, representative for the cohort.
Inter-observer variability
Mean measurement difference between the two observers was 0 mm for cranial nerves V, VII-VIII and IX-X-XI. For II and XII the mean difference was 1 mm, respectively (Table S1). Descriptive frequency analysis showed that the data was not normally distributed. The Wilcoxon signed-rank test showed no significant differences between the measurements of the two observers.
Discussion
Highly conformal irradiation of the craniospinal axis implies particular knowledge of the CSF distribution around the cranial and sacral nerve roots. The outcome of this study, analyzing ten healthy volunteers, demonstrates that CSF can be observed up to 45 mm outside the inner table of the skull base. In contrast, no CSF was observed around the sacral roots outside the spinal canal.
Recently, the SIOPE-BTG consensus guideline on craniospinal target volume delineation for highly conformal radiotherapy has been published [Citation1]. Conventional field-based target volumes have been translated into image-guided high-precision craniospinal target volumes by a group of expert pediatric radiation oncologists, enhanced by MR-images of CSF distribution, as part of the current study. In an attempt to spare the lens, cochlea and intrapelvic structures, special attention should be paid to the optic-, facial-, vestibulocochlear-, and sacral nerves.
For all cases, CSF extension is observed in the dural sheath of the optic nerve up to the posterior part of the eyeball. Based on this observation, arguments do exist to include the full length of both optic nerves in the clinical target volume. In contrast to leukemia, where retinal involvement is occasionally documented as a manifestation of advanced central nervous system leukemia and has led to inclusion of the posterior retina and optic nerves in the target volume, presentations or recurrences in the optic nerves for primary brain tumors are particularly rare [Citation12–16]. One explanation may be the fact that the optic nerves, even when they are not specifically targeted, usually received a therapeutic dose with conventional field-based techniques that use lateral opposed fields. Rene et al. demonstrated that with modern IMRT planning techniques, there is a potential for underdosing the optic nerves when not intentionally targeted [Citation4]. For this reason, and in the absence of a consensus in between pediatric radiation oncologists, the SIOPE-BTG guideline recommends dose registration on different parts of the optic nerve combined with relapse registration for centers not including the whole length of the optic nerves [Citation1].
Another finding with potential impact on clinical target volume delineation is the extension of the CSF around the facial- and vestibulo-cochlear nerve. The current analysis shows that the CSF extends in close vicinity of the cochlea. Patients treated with cranial radiotherapy are at risk for sensorineural hearing loss that likely worsens over time [Citation17]. It is recommended that the average cochlear dose should not exceed 30 Gy delivered over 6 weeks, in particular when cisplatin or high-dose carboplatin are part of the treatment protocol [Citation18,Citation19]. Although studies have shown that sparing the cochlea can lead to reduction of ototoxicity, in our opinion, any attempt to spare the cochlea by excluding CSF within the internal auditory canal should be avoided when the main objective remains cure [Citation20–22].
Finally, the evidence that the CSF distribution at sacral level is limited to the spinal canal makes it possible to give a limited or even no dose to the organs/structures anterior to the sacrum while respecting the inferior limit of the thecal sac, as defined by MRI. This is important, as in addition to higher conformity of modern techniques, reduction of the target volume towards the pelvic structures, can minimize or even eliminate ovarian and uterine dose, and consequently the risk for infertility due to radiotherapy [Citation2,Citation3,Citation23–25].
The CSF extension surrounding the trigeminal nerve is located between the medial part of the temporal lobe and the lateral wall of the sphenoid sinus, also called Meckels cave. Encompassing the middle cranial fossa in the clinical target volume automatically covers the CSF pouch around n. V. The limited extensions of the CSF around the glossopharyngeal-, vagus-, accessory-, and hypoglossal nerve, do project within the bony thickness of the skull base, making it at low risk for a significant underdosage, also given the absence of clinical relevant structures nearby.
Our observations are in line with Noble et al., who studied the CSF extension within the dural reflections of the posterior fossa cranial nerves VII to XII [Citation10]. Different angles at which the slices were taken and the use of different MRI-units and MRI-sequences might be a reason for some slight differences measured in CSF extension between both studies.
We do realize that our observations and corresponding recommendations are MRI-based, while the vast majority of centers performing highly conformal radiotherapy will continue to generate craniospinal target volumes based on conventional CT-scans only. Nevertheless, policy-changing items, like the inclusion of the optic nerves, and the internal auditory canals can easily be visualized on a CT-scan. Furthermore, we are aware of the fact that this study is based on ten healthy adult volunteers, and that extension of CSF in adults may be an overestimation of the extension in the growing child [Citation26]. However, it is our opinion that the message to include the optic nerves and internal auditory canals within the target volume is independent of age or any potential difference in length of CSF extension in between patients.
In conclusion, this study generates evidence for CSF extension beyond the inner table of the skull while no CSF is observed outside the spinal canal at sacral level. Therefore, we strongly recommend the inclusion of the whole length of both optic nerves and internal auditory canals as part of the clinical target volume for craniospinal irradiation in brain tumors. These findings further support the recently published SIOPE-BTG consensus guideline on craniospinal target volume delineation for highly conformal radiotherapy.
Supplemental Material
Download MS Word (27.9 KB)Disclosure statement
The authors report no conflicts of interest.
References
- Ajithkumar T, Horan G, Padovani L, et al. SIOPE- Brain tumor group consensus guideline on craniospinal target volume delineation for high-precision radiotherapy. Radiother Oncol. 2018;128(2):192–197.
- Seravalli E, Bosman M, Lassen-Ramshad Y, et al. Dosimetric comparison of five different techniques for craniospinal irradiation across 15 European centers: analysis on behalf of the SIOP-E-BTG (radiotherapy working group). Acta Oncol. 2018;57(9):1240–1249.
- Kusters JM, Louwe RJ, van Kollenburg PG, et al. Optimal normal tissue sparing in craniospinal axis irradiation using IMRT with daily intrafractionally modulated junction(s). Int J Radiat Oncol Biol Phys. 2011;81(5):1405–1414.
- Rene NJ, Brodeur M, Parker W, et al. A comparison of optic nerve dosimetry in craniospinal radiotherapy planned and treated with conventional and intensity modulated techniques. Radiother Oncol. 2010;97(3):387–389.
- Noble DJ, Ajithkumar T, Lambert J, et al. Highly conformal craniospinal radiotherapy techniques can underdose the cranial clinical target volume if leptomeningeal extension through skull base exit foramina is not contoured. Clin Oncol (R Coll Radiol). 2017;29(7):439–447.
- Jereb B, Sundaresan N, Horten B, et al. Supratentorial recurrences in medulloblastoma. Cancer. 1981;47(4):806–809.
- Miralbell R, Bleher A, Huguenin P, et al. Pediatric medulloblastoma: radiation treatment technique and patterns of failure. Int J Radiat Oncol Biol Phys. 1997;37(3):523–529.
- Carrie C, Hoffstetter S, Gomez F, et al. Impact of targeting deviations on outcome in medulloblastoma: study of the French Society of Pediatric Oncology (SFOP). Int J Radiat Oncol Biol Phys. 1999;45(2):435–439.
- Taylor RE, Bailey CC, Robinson KJ, et al. Impact of radiotherapy parameters on outcome in the International Society of Paediatric Oncology/United Kingdom Children’s Cancer Study Group PNET-3 study of preradiotherapy chemotherapy for M0-M1 medulloblastoma. Int J Radiat Oncol Biol Phys. 2004;58(4):1184–1193.
- Noble DJ, Scoffings D, Ajithkumar T, et al. Fast imaging employing steady-state acquisition (FIESTA) MRI to investigate cerebrospinal fluid (CSF) within dural reflections of posterior fossa cranial nerves. Br J Radiol. 2016;89(1067):20160392.
- IBM Corp. Released 2016. IBM SPSS Statistics for Windows, Version 24.0. Armonk (NY): IBM Corp.
- Halperin E, Laurie F, Fitzgerald TJ. An evaluation of the relationship between the quality of prophylactic cranial radiotherapy in childhood acute leukemia and institutional experience: a Quality Assurance Review Center-Pediatric Oncology Group Study. Int J Radiat Oncol Biol Phys. 2002;53(4):1001–1004.
- Garrity JA, Herman DC, Dinapoli RP, et al. Isolated metastasis to optic nerve from medulloblastoma. Ophtalmology. 1989;96(2):207–210.
- Hertle RW, Robb RM. Pineoblastoma metastatic to the optic nerve. J Clin Neuroophthalmol. 1990;10(2):95–99.
- Manor RS, Bar-Ziv J, Tadmor R, et al. Pineal germinoma with unilateral blindness. Seeding of germinoma cells in optic nerve sheath. J Clin Neuroophthalmol. 1990;10(4):239–243.
- Nakajima T, Kumabe T, Jokura H, et al. Recurrent germinoma in the optic nerve: report of two cases. Neurosurgery. 2001;48:217–218.
- Bass JK, Hua CH, Huang J, et al. Hearing loss in patients who received cranial radiation therapy for childhood cancer. JCO. 2016;34(11):1248–1255.
- Grewal S, Merchant T, Reymond R, et al. Auditory late effects of childhood cancer therapy: a report from the Children’s Oncology Group. Pediatrics. 2010;125(4):e938–950.
- Clemens E, van den Heuvel-Eibrink MM, Mulder RL, et al. Recommendations for ototoxicity surveillance for childhood, adolescent, and young adult cancer survivors: a report from the International Late Effects of Childhood Cancer Guideline Harmonization Group in collaboration with PanCare Consortium. Lancet Oncol. 2019;20(1):e29–e41.
- Huang E, The BS, Strother DR, et al. Intensity-modulated radiation therapy for pediatric medulloblastoma: early report on the reduction of ototoxicity. Int J Radiat Oncol Biol Phys. 2002;52(3):599–605.
- Paulino AC, Lobo M, Teh BS, et al. Ototoxicity after intensity-modulated radiation therapy and cisplatin-based chemotherapy in children with medulloblastoma. Int J Radiat Oncol Biol Phys. 2010;78(5):1445–1450.
- Paulino AC, Mahajan A, Ye R, et al. Ototoxicity and cochlear sparing in children with medulloblastoma: Proton vs. photon radiotherapy. Radiother Oncol. 2018;128(1):128–132.
- Lester-Coll NH, Morse CB, Zhai HA, et al. Preserving fertility in adolescent girls and young women requiring craniospinal irradiation: a case report and discussion of options to be considered prior to treatment. J Adolesc Young Adult Oncol. 2014;3(2):96–99.
- Balachandar S, Dunkel IJ, Khakoo Y, et al. Ovarian function in survivors of childhood medulloblastoma: impact of reduced dose craniospinal irradiation and high-dose chemotherapy with autologous stem cell rescue. Pediatr Blood Cancer. 2015;65:317–321.
- Harden SV, Twyman N, Lomas DJ, et al. A method for reducing ovarian doses in whole neuro-axis irradiation for medulloblastoma . Radiother Oncol. 2003;69(2):183–188.
- Bogin B, Varela-Silva MI. Leg length, body proportion, and health: a review with a note on beauty. Int J Environ Res Public Health. 2010;7(3):1047–1075.