Abstract
Background: The aim of this study was to compare adaptive intensity modulated proton therapy (IMPT) robustness and organ sparing capabilities with that of adaptive volumetric arc photon therapy (VMAT).
Material and methods: Eighteen lung cancer patients underwent a planning 4DCT (p4DCT) and 5 weekly repeated 4DCT (r4DCT) scans. Target volumes and organs at risk were manually delineated on the three-dimensional (3D) average scans of the p4DCT (av_p4DCT) and of the r4DCT scans (av_r4DCT). Planning target volume (PTV)-based VMAT plans and internal clinical target volume (ICTV)-based robust IMPT plans were optimized in 3D on the av_p4DCT and re-calculated on the av_r4DCTs. Re-planning on av_r4DCTs was performed when indicated and accumulated doses were evaluated on the av_p4DCT.
Results: Adaptive VMAT and IMPT resulted in adequate ICTV coverage on av_r4DCT in all patients and adequate accumulated-dose ICTV coverage on av_p4DCT in 17/18 patients (due to a shrinking target in one patient). More frequent re-planning was needed for IMPT than for VMAT. The average mean heart dose reduction with IMPT compared with VMAT was 4.6 Gy (p = .001) and it was >5 Gy for five patients (6, 7, 8, 15, and 22 Gy). The average mean lung dose reduction was 3.2 Gy (p < .001). Significant reductions in heart and lung V5 Gy were observed with IMPT.
Conclusion: Robust-planned IMPT required re-planning more often than VMAT but resulted in similar accumulated ICTV coverage. With IMPT, heart and lung mean dose values and low dose regions were significantly reduced. Substantial cardiac sparing was obtained in a subgroup of five patients (28%).
Introduction
Radiotherapy results in radiation dose exposure to normal tissue with consequently the risk of radiation induced toxicity. With current photon radiotherapy of lung cancer, the radiation dose to the surrounding organs at risk (OAR), such as the heart, lungs, and esophagus can be substantial [Citation1]. It has been demonstrated that high doses delivered to the heart poses serious risks of cardiac events that may hamper overall survival [Citation2]. Furthermore, when large volumes of lung receive low dose radiation, the risk of pulmonary complications increases significantly [Citation3]. With pencil-beam scanned intensity modulated proton therapy (IMPT), the dose distribution can be designed to conform better to the target, reducing the radiation dose to the surrounding normal tissue [Citation4]. These dose reductions are expected to reduce the risk of cardiac, pulmonary, and esophageal complications [Citation5]. However, the potential major advantages of IMPT for lung cancer are challenged by respiratory motion, as well as the relatively high density gradients in the thorax region. Proton therapy is more sensitive (less robust) to uncertainties and anatomical variations than photon therapy and this may lead to inadequate coverage of the target volumes and/or may offset the dose expected to be delivered to OARs [Citation6]. In order to safely implement IMPT in clinical practice, thorough knowledge of the influence of setup errors, and inter- and intra-fraction variations is essential [Citation7]. Although randomized studies are scarce [Citation5], an increasing number of articles report on favorable results following proton therapy for lung cancer [Citation8–11]. For the comparison of IMPT with modern photon radiotherapy, mainly in silico studies are available, comparing IMPT or scattering proton techniques with modern photon techniques on the planning CT [Citation11–15]. Only a limited number of studies included inter-fractional changes in position and anatomy [Citation16], or robust treatment planning optimization for proton therapy [Citation14,Citation17].
The aim of this study was to test the robustness of IMPT for lung cancer on weekly repeated 4DCT (r4DCT), and to compare the organ sparing capabilities and robustness of IMPT to that obtained with current volumetric modulated arc photon therapy (VMAT). Our secondary aim was to investigate the impact of adaptive re-planning by evaluating target coverage and OAR dose parameter, both on weekly 4D repeat CTs and by evaluating whole-course dose accumulations.
Material and methods
Patients and imaging
The study population consisted of 18 patients with either non-small–cell lung cancer (NSCLC) of any histological subtype, or small-cell lung cancer (SCLC) stage III (). All patients were treated with photon-based VMAT with curative intent in combination with chemotherapy. All patients underwent a planning 4DCT scan (p4DCT) and weekly repeat 4DCT scans (r4DCT) without contrast agents. Time-based 4DCT scans were acquired using a large bore 64-slice CT scanner (Somatom AS Open 64-RT Pro, Siemens Medical Systems, Erlangen, Germany), in supine (treatment) position, with a 2 mm slice thickness and an in-plane resolution of 1.0 mm. The patients’ respiratory phases were determined using an Anzai belt (Anzai Medical, Tokyo, Japan). The 4DCT images were reconstructed into 10 respiratory phase bins and 3D average intensity projection (AIP) CT images were created. The analysis presented here focuses on the average p4DCTs (av_p4DCT) and the average r4DCTs (av_r4DCT). This prospective study was approved by the medical ethics review committee of the UMCG and written informed consent was obtained from all patients.
Table 1. Baseline patient characteristics.
Target definition
The gross tumor volume (GTV) was delineated by a radiation oncologist on the av_p4DCT. It included both nodal and primary tumor volumes and was expanded manually to encompass the GTV on all phases of the 4DCT. The resulting contour defined the internal gross tumor volume (IGTV) [Citation18]. It was expanded with a uniform margin of 5 mm to constitute the internal clinical target volume (ICTV) and with another 6 mm to constitute the planning target volume (PTV). The GTVs and IGTVs were copied to the av_r4DCTs using the intensity-based, anatomically constrained, deformable image registration (DIR) algorithm in the RayStation v4.99 treatment planning system (RaySearch Laboratories, Stockholm) [Citation19]. Subsequently, the IGTVs were manually adjusted by a radiation oncologist if necessary, and expanded to define the ICTV. The heart, the total lung volume and the spinal cord were delineated as organs at risk (OAR).
VMAT and IMPT treatment planning
Full VMAT treatment planning was performed using two ipsilateral 180° arcs. The prescribed total dose to the PTV was 60 Gy (25 fractions of 2.4 Gy). PTV optimized VMAT plans were created on the av_p4DCT. Plans were iteratively optimized such that (in order of priority) the mean dose to the lungs, heart, and esophagus was as low as possible without violating the PTV and spinal cord planning objectives. Hotspots with ≥107% of the prescribed dose were limited to 2 cm3. For IMPT, typically three beam directions were chosen considering plan robustness, e.g., beams avoiding high density gradients and beams with short path lengths to the target. ICTV-based robust optimized IMPT plans were created with the same planning goals as for VMAT, except that robust settings [Citation20] were used to cover the ICTV (6 mm for setup and 3% for range uncertainties) [Citation20–22] and the objective values and weights were adjusted for IMPT. When an optimal plan was reached, a robustness evaluation was performed simulating error scenarios for both photons and protons [Citation23]. The treatment plan was accepted when the voxel-wise minimum ICTV D98 was ≥57 Gy and when the voxel-wise maximum spinal cord D0.1 cm3 was ≤50 Gy. During IMPT optimization, auto-spot spacing was used to distribute the spots in the lateral direction and in depth. A treatment machine was used with a spot size (in air at isocentre) of 3 mm (σ) at 230 MeV and a minimum energy of 70 MeV and a pencil beam dose algorithm was used for dose calculation. All dose parameters are reported in Gy (RBE), assuming an RBE of 1.1 for protons.
Plan perturbations on weekly repeat CTs
The VMAT and IMPT plans created on the av_p4DCT were re-calculated on the weekly av_r4DCTs, simulating 26, and for protons 52 error scenarios (2 mm for both setup uncertainties, and 3% for IMPT range uncertainties). The 2 mm setup uncertainty has been established from an internal assessment accounting for imaging versus treatment isocentre accuracy as well as intra-fractional setup error. When the voxel-wise minimum ICTV D98 on an av_r4DCT was <56 Gy or when the voxel-wise maximum spinal cord D0.1 cm3 was >51 Gy, PTV-based (VMAT) or ICTV-based robust (IMPT) re-planning was performed on that av_r4DCT. The re-plan was then used to evaluate subsequent av_r4DCT scans.
Treatment-trajectories and evaluation of accumulated dose on the av_p4DCT
For each patient, virtual ‘treatment-trajectories’ with and without re-planning were considered, where a treatment-trajectory is the set of plans for all weeks of treatment. Patients had one treatment-trajectory when no re-planning was required, two treatment-trajectories when re-planning was needed once, etcetera. For each treatment-trajectory, the computed dose per voxel for each av_r4DCT was warped to the av_p4DCT and then accumulated and evaluated. Dose was accumulated accounting 5 fractions per av_r4DCT, taking into account adapted plans on the corresponding and subsequent av_r4DCTs.
Results
The 18 patients included in this study were a representative sample of the population of lung patients treated at our institute (). A total of 18 av_p4DCT scans and 87 av_r4DCT scans were available for the current analysis. Sixteen patients had five weekly av_r4DCTs, one patient had four (due to downtime of the CT scanner) and one patient had three av_r4DCTs (due to the fractionation schedule of 30 fractions, twice daily). The ICTV averaged over patients with five weekly scans decreased over time; for the 16 patients with five r4DCTs available, it was 119 cm3 on the av_p4DCT, and on the subsequent weekly av_r4DCTs it was 117 cm3, 109 cm3, 103 cm3, 98 cm3, and 93 cm3, respectively. The 3D displacement of the GTV during breathing, averaged over all patients and all four-dimensional (4D) CTs, was 4 mm ± 3 mm, and ranged from 1 to 15 mm.
Re-planning was indicated (ICTV D98 < 56 Gy and/or spinal cord D0.1 cm3 > 51 Gy) for two patients planned with VMAT based on the av_r4DCT in the second week. For IMPT plans, re-planning was indicated according to the weekly av_r4DCTs for eight patients, of which five patients needed re-planning once and three patients needed re-planning more than once (). When the ICTV D98 coverage on the av_r4DCTs was between 56 Gy and 57 Gy, no re-planning was performed. This was the case with VMAT for 10 av_r4DCTs (of five patients) and with IMPT for 11 av_r4DCTs (of six patients). The decision not to re-plan in these cases never compromised the accumulated av_p4DCT ICTV coverage with VMAT and IMPT. When re-planning was omitted for all patients, the accumulated av_p4DCT ICTV coverage with VMAT and IMPT would have still been adequate for 18 and 15 patients, respectively. In retrospect, three of the eight patients for whom IMPT re-planning was performed needed the plan adaptation for adequate accumulated av_p4DCT ICTV coverage. One patient who required IMPT re-planning had inadequate ICTV coverage that was caused by a dense mass in the lung, of which the volume, position and shape changed every week (Supplementary Figure 1). For another patient, the inadequate dose coverage was due to the shoulder position on the av_r4DCTs, which was different from the av_p4DCT. The third patient had a slight rotation combined with a slight position change on the av_r4DCTs, with respect to the av_p4DCT, causing inadequate dose distributions.
Figure 1. IMPT and VMAT re-planning frequencies for treatment-trajectories with and without re-planning. Decisions to re-plan were based on the weekly repeated 4DCTs only, i.e., without dose accumulation. Whole-course ICTV coverage was evaluated with dose accumulation on the average planning 4DCT.
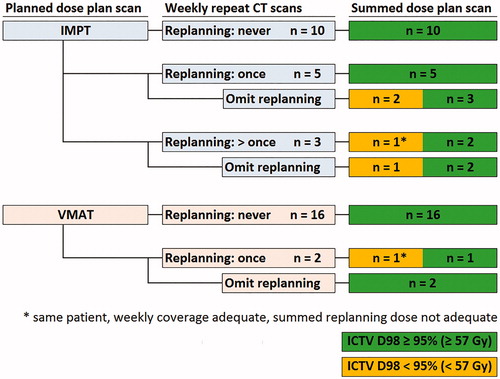
Accumulated full re-planning treatment-trajectories had adequate ICTV coverage on the av_p4DCT with both VMAT and IMPT for 17 patients (). For one patient with NSCLC, re-planning decreased the ICTV D98 on the av_p4DCT with both VMAT and IMPT. This decrease was mainly due to a shrinking ICTV over the course of treatment in combination with shrinking treatment fields after re-planning.
The accumulated average mean heart dose in all patients after full re-planning with VMAT and IMPT was 6.6 Gy and 2.0 Gy (Wilcoxon signed-rank test p = .001), respectively (). For five patients, the mean heart dose was reduced by more than 5 Gy (–6.1 Gy, –7.2 Gy, –8.0 Gy, –15.0 Gy, and –21.5 Gy) (). The average heart V5 Gy in all patients (Vx Gy is the relative volume receiving ≥ x Gy) with VMAT and IMPT was 27.1% and 8.0% (p < .001), respectively. The average mean lung dose was 9.8 Gy and 6.6 Gy (p < .001). The average lung V5Gy with VMAT and IMPT was 40.1% and 23.0% (p < .001), respectively. Mean dose reductions were also observed for the esophagus and the integral dose ().
Figure 2. Dose volume histograms for heart and lungs contours with VMAT and IMPT (accumulated dose including re-planning).
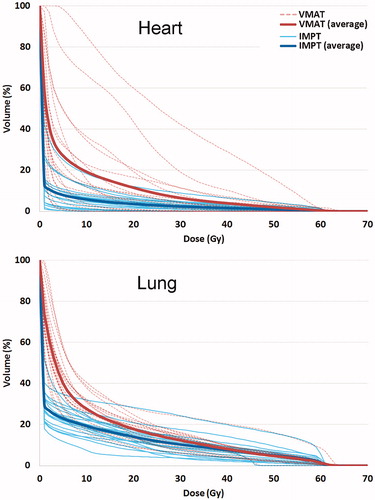
Figure 3. Accumulated dose distributions with VMAT and IMPT and mean heart dose reductions with IMPT in a subgroup of patients with substantial cardiac sparing with IMPT.
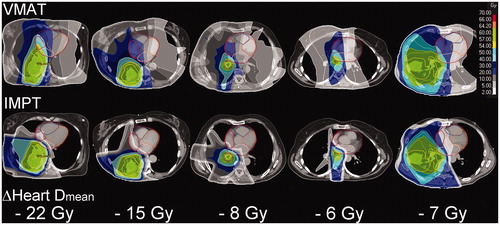
Table 2. Full re-planning trajectories ICTV D98 and OAR mean dose summed on av_p4DCT.
Discussion
To our knowledge, this is the first study to investigate the inter-fraction robustness of adaptive IMPT relative to VMAT on 4D repeat CTs obtained weekly throughout the course of treatment. The number of required and unnecessary re-planning procedures between IMPT and VMAT were compared both on the weekly 4D repeat CTs and by evaluating whole-course dose accumulations. We found that, without any re-planning, IMPT for lung cancer results in adequate coverage of the target volume for 15/18 patients. With re-planning, adequate whole-course accumulated dose coverage of the target volume was obtained in 17/18 patients with both IMPT and VMAT.
Re-planning was required more often for IMPT than for VMAT; eight patients required re-planning at least once for IMPT based on the weekly scans. However, in retrospect, five of these patients did not require any re-planning when the accumulated dose on av_p4DCT was evaluated. Additional research is needed to find the optimal decision criterion to avoid unnecessary plan adaptations. In general, more insights are required if plan adaptation decisions should be made based on day-specific dose recalculations or accumulated reconstructed doses as proposed by Meijers et al. [Citation24]. Only two patients required re-planning for VMAT. With IMPT, in three patients the main causes of decreased ICTV coverage were density changes in the lung and changes in the patient position. These three patients did not require re-planning with VMAT. Inadequate accumulated ICTV coverage on av_p4DCT was rarely caused by a shifted position of the ICTV on the av_r4DCT. For one patient, who had an ICTV that shrunk and changed position each week, re-planning was required for both IMPT and VMAT. Re-planning in this patient improved the ICTV coverage on the weekly av_r4DCTs, while the accumulated dose did not adequately cover the ICTV on the av_p4DCT.
For most patients, weekly IMPT evaluation with or without one single plan adaptation on av_r4DCTs appeared sufficient for adequate accumulated ICTV coverage on the av_p4DCT. Further research, based on daily imaging such as CBCT, may show which patients benefit from daily imaging and more frequent treatment plan adaptation [Citation16].
For IMPT, robust planning and robustness evaluation is essential [Citation25]. In this study, IMPT was robustly planned in 3D, while for VMAT optimization, PTV-based planning was used, as this best represents current clinical practice. The robustness settings for IMPT used similar geometrical uncertainties as for VMAT, which is accounted for in the CTV to PTV margin (6 mm).
In this study we defined the target volume using the ICTV approach, which is more conservative than e.g., the mid-ventilation or mid-position approaches. It is not yet clear which method is best for IMPT. Additional studies are necessary to investigate the influence of such factors. Various choices made in this study may have influenced the results of this study in a certain degree, e.g., the exact number of plan adjustments performed or the exact dose reductions obtained with IMPT relative to VMAT may be different under different circumstances. However, as we are currently preparing to implement IMPT for lung cancer, the current study, and other research being performed at this moment, offers us confidence that a robust treatment is feasible for our patients. We foresee to start with patients with limited motion amplitudes, which will be closely monitored by using 3D and 4D imaging throughout the course of fractionated proton treatment and we envision a workflow described in a recent publication of our group [Citation24].
Interplay effects could impact ICTV coverage, especially with IMPT [Citation26]. These effects were not considered in the current study. Instead we used AIP for plan generation and evaluation as we feel this is a valid representation of the positions of target and OARs over the course of treatment. This method is preferred as it appears to provide for a robust but also efficient workflow. In a connecting article, we report on the use of 4D optimization and verification and the potential benefits in terms of additional OAR sparing [Citation27]. The 4D method described in that article requires the use of treatment log files for verification and delineation of targets and OARs on each breathing phase, which is very labor intensive. Also increased calculation times needed for treatment plan optimization render this approach not yet clinically practically viable. The 4D workflow might also turn out not to be ‘required’ for the clinic. The effects due to interplay are expected to be limited for the current population as the GTV displacement was relatively small (the population average 3D-vector displacement of the GTV was largest in the first week of treatment: 5 mm ± 4 mm). Furthermore, dose fractionation is expected to mitigate the effects of interplay because the dose distribution is averaged over 25 fractions. This is consistent with the observations in the current study: although individual fractions resulted in reduced ICTV coverage in some patients, in most cases this effect disappeared when the whole treatment course dose was accumulated. Furthermore, the differences in OAR dose were minor when comparing treatment trajectories with and without re-planning.
The accumulated deformed dose distribution presented in this study may be influenced by the accuracy and validity of the DIR procedure [Citation28]. The applied DIR method has been approved for clinical contour propagation and dose accumulation at our institute and has the option to use the ICTV as controlling region for increased accuracy [Citation29]. Although all DIR matches were visually checked for validity, and the same DIR was used for VMAT and IMPT, dosimetric errors may still exist [Citation30]. It is, however, not expected that this will alter the conclusions of this study, as errors are reduced by repeated imaging and the tumor motion in this study was relatively small.
The secondary aim of this study was to investigate the organ sparing capabilities of IMPT under robust treatment conditions (robust planning, weekly plan evaluation, and re-planning when necessary) when compared with VMAT. While moderate mean dose reductions were observed for the lung and the esophagus contours with IMPT, significant reductions could be obtained for low-dose regions (V5 Gy) of the lung. Reduction of these volumes receiving 5 Gy may significantly decrease the risk of pulmonary complications, pneumonia and acute respiratory stress syndrome [Citation3,Citation31].
The reductions in V5 Gy of the heart and the mean heart dose were statistically significant. In a subgroup of five patients the mean heart dose reduction was substantial, ranging from 6 to 22 Gy. Unfortunately, no validated NTCP models for cardiovascular events are currently available for lung cancer patients, and the observed cardiac mean dose reductions with IMPT cannot be translated into reduced risks of cardiovascular complications. However, several authors have reported on reductions in overall survival of patients who received radiotherapy for lung cancer [Citation32]. In a recent randomized trial (RTOG-0617) it was demonstrated that patients who received an escalated dose (74 Gy) to the target had worse survival than patients who received the standard dose of 60 Gy. Moreover, overall survival was associated with cardiac dose, especially the heart V5 Gy and the heart V30 Gy [Citation2]. A single institution analysis of 251 NSCLC patients showed that keeping the heart V50 Gy below 25% was associated with a nearly 20% absolute improvement in 2-year overall survival [Citation33].
This indicates that a subgroup of lung cancer patients with significant reductions in incidental radiation dose to the heart is likely to substantially benefit from proton therapy. In the current study, we demonstrated that such dose reductions can be obtained with IMPT without compromising adequate target coverage.
Conclusion
We found in a group of 18 patients that robust 3D-planned IMPT required re-planning on weekly average 4DCT scans more often than for PTV-based VMAT. With re-planning, the accumulated ICTV coverage on average-intensity 4DCT scans was similar for both IMPT and VMAT. With robustly optimized IMPT, significant reductions in V5 Gy values for the lung were obtained. Furthermore, as seen in a subgroup of 5/18 patients, significant cardiac dose reductions were achieved with IMPT.
Oral presentation at ASTRO 2018: Robustness and Organ Sparing Potential of Intensity Modulated Proton Therapy for Lung Cancer. International journal of radiation oncology biology physics, vol. 102, issue 3, p. S56–S57. Hans Paul van der Laan has a MSc in epidemiology and was responsible for the statistical analysis for this article.
Supplemental Material
Download PDF (1.5 MB)Disclosure statement
The department of Radiation Oncology of the UMCG has research agreements with IBA and RaySearch Laboratories. J.A. Langendijk received non-financial support and other from IBA and RaySearch Laboratories and a fee from IBA for giving a presentation at a symposium and giving consultancy. This has been paid to UMCG Research B.V. All other authors have no conflicts of interest to disclose.
Additional information
Funding
References
- Chun SG, Hu C, Choy H. Impact of intensity-modulated radiation therapy technique for locally advanced non-small-cell lung cancer: a secondary analysis of the NRG oncology RTOG 0617 randomized clinical trial. J Clin Oncol. 2017;35(1):56–62.
- Bradley JD, Paulus R, Komaki R, et al. Standard-dose versus high-dose conformal radiotherapy with concurrent and consolidation carboplatin plus paclitaxel with or without cetuximab for patients with stage IIIA or IIIB non-small-cell lung cancer (RTOG 0617): a randomised, two-by-two factorial p. Lancet Oncol. 2015;16:187–199.
- Lee HK, Vaporciyan AA, Cox JD, et al. Postoperative pulmonary complications after preoperative chemoradiation for esophageal carcinoma: correlation with pulmonary dose-volume histogram parameters. Int J Radiat Oncol Biol Phys. 2003;57(5):1317–1322.
- Welsh J, Gomez D, Palmer MB, et al. Intensity-modulated proton therapy further reduces normal tissue exposure during definitive therapy for locally advanced distal esophageal tumors: a dosimetric study. Int J Radiat Oncol Biol Phys. 2011;81(5):1336–1342.
- Liao Z, Lee JJ, Komaki R, et al. Bayesian adaptive randomization trial of passive scattering proton therapy and intensity-modulated photon radiotherapy for locally advanced non–small-cell lung cancer. J Clin Oncol. 2018;36(18):1813–1822.
- Lomax AJ. Intensity modulated proton therapy and its sensitivity to treatment uncertainties 2: the potential effects of inter-fraction and inter-field motions. Phys Med Biol. 2008;53(4):1043–1056.
- Berman A, James S, Rengan R. Proton beam therapy for non-small cell lung cancer: current clinical evidence and future directions. Cancers 2015;7(3):1178–1190.
- Chang JY, Verma V, Li M, et al. Proton beam radiotherapy and concurrent chemotherapy for unresectable stage III non–small cell lung cancer: final results of a phase 2 study. JAMA Oncol. 2017;3(8):e172032.
- Higgins KA, O'Connell K, Liu Y, et al. National Cancer Database analysis of proton versus photon radiation therapy in non-small cell lung cancer. Int J Radiat Oncol Biol Phys. 2017;97(1):128–137.
- Nguyen Q, Ly NB, Komaki R, et al. Long-term outcomes after proton therapy, with concurrent chemotherapy, for stage II-III inoperable non-small cell lung cancer. Radiother Oncol. 2015;115(3):367–372.
- Zhang X, Li Y, Pan X, et al. Intensity-modulated proton therapy reduces the dose to normal tissue compared with intensity-modulated radiation therapy or passive scattering proton therapy and enables individualized radical radiotherapy for extensive stage IIIB non-small-cell lung cancer: a virtual clinical study. Int J Radiat Oncol Biol Phys. 2010;77(2):357–366.
- Chang JY, Zhang X, Wang X, et al. Significant reduction of normal tissue dose by proton radiotherapy compared with three-dimensional conformal or intensity-modulated radiation therapy in Stage I or Stage III non-small-cell lung cancer. Int J Radiat Oncol Biol Phys. 2006;65(4):1087–1096.
- Roelofs E, Engelsman M, Rasch C, et al. Results of a multicentric in silico clinical trial (ROCOCO): comparing radiotherapy with photons and protons for non-small cell lung cancer). J Thorac Oncol. 2012;7(1):165–176.
- Inoue T, Widder J, van Dijk LV, et al. Limited impact of setup and range uncertainties, breathing motion, and interplay effects in robustly optimized intensity modulated proton therapy for stage III non-small cell lung cancer. Int J Radiat Oncol Biol Phys. 2016;96(3):661–669.
- Liu C, Sio TT, Deng W, et al. Small-spot intensity-modulated proton therapy and volumetric-modulated arc therapies for patients with locally advanced non-small-cell lung cancer: a dosimetric comparative study. J Appl Clin Med Phys. 2018;19(6):140–148.
- Hoffmann L, Alber M, Jensen MF, et al. Adaptation is mandatory for intensity modulated proton therapy of advanced lung cancer to ensure target coverage. Radiother Oncol. 2017;122(3):400–405.
- Liu W, Schild SE, Chang JY, et al. Exploratory study of 4D versus 3D robust optimization in intensity modulated proton therapy for lung cancer. Int J Radiat Oncol Biol Phys. 2016;95(1):523–533.
- International Commission on Radiation Units and Measurements (ICRU). Prescribing, recording, and reporting photon beam therapy. ICRU Report 62 (Supplement to ICRU Report 50). Bethesda (MD): ICRU Publications; 1999.
- Weistrand O, Svensson S. The ANACONDA algorithm for deformable image registration in radiotherapy. Med Phys. 2015;42(1):40–53.
- Fredriksson A, Forsgren A, Hårdemark B. Minimax optimization for handling range and setup uncertainties in proton therapy. Med Phys. 2011;38(3):1672.
- Albertini F, Hug EB, Lomax AJ. Is it necessary to plan with safety margins for actively scanned proton therapy? Phys Med Biol. 2011;56(14):4399–4413.
- Lomax AJ. Intensity modulated proton therapy and its sensitivity to treatment uncertainties 1: the potential effects of calculational uncertainties. Phys Med Biol. 2008;53(4):1027–1042.
- Korevaar EW, Habraken SJM, Scandurra D. Practical robustness evaluation in radiotherapy – a photon and proton-proof alternative to PTV-based plan evaluation. Radiother Oncol. [cited 2019 Sep 3]. DOI:10.1016/j.radonc.2019.08.005.
- Meijers A, Jakobi A, Stützer K, et al. Log file-based dose reconstruction and accumulation for 4D adaptive pencil beam scanned proton therapy in a clinical treatment planning system: implementation and proof-of-concept. Med Phys. 2019;46(3):1140–1149.
- Unkelbach J, Bortfeld T, Martin BC, et al. Reducing the sensitivity of IMPT treatment plans to setup errors and range uncertainties via probabilistic treatment planning. Med Phys. 2009;36(1):149.
- Li Y, Kardar L, Li X, et al. On the interplay effects with proton scanning beams in stage III lung cancer. Med Phys. 2014;41:1–7.
- Ribeiro CO, Meijers A, Korevaar EW, et al. Comprehensive 4D robustness evaluation for pencil beam scanned proton plans. Radiother Oncol. 2019;136:185–189.
- Kadoya N, Nakajima Y, Saito M, et al. Multi-institutional validation study of commercially available deformable image registration software for thoracic images. Int J Radiat Oncol Biol Phys. 2016;96(2):422–431.
- Kierkels RGJ, den Otter LA, Korevaar EW, et al. An automated, quantitative, and case-specific evaluation of deformable image registration in computed tomography images. Phys Med Biol. 2018;63(4):045026.
- Ribeiro CO, Knopf A, Langendijk JA, et al. Assessment of dosimetric errors induced by deformable image registration methods in 4D pencil beam scanned proton treatment planning for liver tumours. Radiother Oncol. 2018;128(1):174–181.
- Salama JK, Pang H, Bogart JA, et al. Predictors of pulmonary toxicity in limited stage small cell lung cancer patients treated with induction chemotherapy followed by concurrent platinum-based chemotherapy and 70Gy daily radiotherapy: CALGB 30904. Lung Cancer 2013;82(3):436–440.
- PORT Meta-analysis Trialists Group. Postoperative radiotherapy in non-small-cell lung cancer: systematic review and meta-analysis of individual patient data from nine randomised controlled trials. Lancet 1998;352:257–263.
- Speirs CK, DeWees TA, Rehman S, et al. Heart dose is an independent dosimetric predictor of overall survival in locally advanced non-small cell lung cancer. J Thorac Oncol. 2017;12(2):293–301.