Abstract
Background
The treatment of centrally-located early-stage non-small cell lung cancer (NSCLC) with image-guided stereotactic body radiotherapy (SBRT) is challenging due to the proximity of critical normal structures to the tumor target. The purpose of this study was to report the results of our experience in treating centrally-located early-stage NSCLC with hypofractionated proton therapy (PT).
Material and methods
Between 2009 and 2018, 23 patients with T1–T2N0M0 NSCLC (T1, 46%; T2, 54%) were treated with image-guided hypofractionated double-scattering PT. The median age at the time of treatment was 74 years (range, 58–88). Patients underwent 4-dimensional computed tomography (CT) simulation following fiducial marker placement, and daily image guidance was performed. All patients were treated with 60 GyRBE in 10 fractions. Patients were assessed for CTCAEv4 toxicities weekly during treatment, and at regular follow-up intervals with CT imaging for tumor assessment. Overall survival, cause-specific survival, local control, regional control, and metastases-free survival were evaluated using cumulative incidence with competing risks.
Results
Median follow-up for all patients was 3.2 years (range, 0.2–9.2 years). Overall survival rates at 3 and 5 years were 81% and 50% (95% CI, 27–79%), respectively. Cause-specific survival rates at 3 and 5 years were 81% and 71% (95% CI, 46–92%). The 3-year local, regional, and distant control rates were 90%, 81%, and 87%, respectively. Three patients (13%) experienced local recurrences as their first recurrence, at a median time of 28 months from completion of radiation (range, 18–61 months). Two patients (9%) experienced late grade 3 toxicities, including 1 patient who developed a bronchial stricture that required stent placement.
Conclusion
Image-guided hypofractionated PT for centrally-located early-stage NSCLC provides excellent local control with low rates of grade ≥3 toxicities. For tumors in sensitive locations, PT may provide safer treatment than photon-based treatments due to its dosimetric advantages.
Introduction
In a recently published prospective randomized controlled trial, stereotactic body radiation therapy (SBRT) for the treatment of peripherally-located early-stage non-small cell lung cancer (NSCLC) was shown to improve overall survival and local control when compared to standard fractionated radiation therapy [Citation1]. Through the use of highly focused ablative doses of radiation to peripheral lung tumors delivered over short treatment courses, SBRT is able to achieve 5-year rates of overall survival and local control of 40% and 80%, respectively [Citation2]. Patients infrequently (<5%) experience severe late pulmonary toxicities, such as grade 3 pneumonitis [Citation3], and are often able to preserve their quality of life [Citation4]. In contrast, central tumors, defined as those that are within a 2-cm radius of large airways, present a challenge to radiation oncologists as the use of SBRT results in higher rates of toxicity [Citation5,Citation6] due to the proximity of critical normal structures to the tumor target.
There is increasing interest in the use of proton therapy for centrally-located early-stage NSCLC since its favorable dosimetric properties may allow it to reduce treatment-related toxicities [Citation7,Citation8]. Owing to the phenomena of the Bragg peak, protons are able to deposit most of their dose at a specific depth, and thus, have a finite range. By carefully selecting the energy of the proton beam and accounting for the target tissue’s density and thickness, the location of the Bragg peak can be adjusted to maximize dose deposition in the target tissue, while minimizing dose to surrounding critical normal structures.
Despite the potential advantages of proton therapy, data regarding its use in the treatment of centrally-located early-stage NSCLC are scant. The purpose of this study is to report the results of our experience treating centrally-located early-stage NSCLC with hypofractionated proton therapy, with a focus on disease control and toxicities.
Material and methods
Patients
Between October 2009 and August 2018, 23 patients with non-metastatic, centrally-located, biopsy proven T1–T2N0 NSCLC who were medically inoperable were prospectively enrolled in an institutional review board-approved outcome-tracking protocol. In keeping with the RTOG 0813 definition, central tumors were those defined as touching the proximal bronchial tree, within a 2-cm volume of the proximal bronchial tree, or those that were adjacent to the mediastinal or pericardial pleura [Citation9]. Thirteen patients were concurrently enrolled in a phase II clinical trial (NCT00875901) that evaluated the safety and efficacy of hypofractionated proton therapy for early-stage NSCLC of all locations. All patients included in this review underwent workup that included a positron emission tomography (PET) scan (96%) and/or computed tomography (CT) scan of the chest and upper abdomen, pulmonary function testing, complete blood count with differential, and comprehensive metabolic panel. All patients had histologically proven NSCLC. Mediastinal lymph node sampling was optional.
Treatment planning
Gold fiducials were typically placed around the tumor prior to CT simulation scan via bronchoscopic placement. For treatment planning, patients were immobilized in the supine position on a wing board with post hand-grips. A small Vac-Lok (CIVCO, Kalona, IA) cushion was used on the wing board to stabilize the arms and the head. The pelvis and legs were stabilized with a larger vacuum bag. The patients laid on the table with a gap between the head and pelvic vacuum bags to minimize material in the beam path. Patients underwent a 4-dimensional (4D) CT simulation scan with spacing ≤3 mm between slices. The target lesion was outlined and designated as the gross tumor volume (GTV) in all 10 phases of the 4D CT scan using the lung window, and soft tissue windows helped discern adjacent anatomy. The maximum magnitude of tumor motion permitted was 1 cm in any direction. The GTV was expanded to cover the entire space occupied by the tumor in the 4 D CT scan, and labeled as the internal tumor volume (iGTV). For patients treated earlier, a 6-mm uniform expansion was applied to the iGTV to generate an ITV, but, as our practices changed, an expansion of 0 mm was applied to the iGTV to generate an ITV. The ITV was then expanded 5 mm uniformly to generate the planning target volume (PTV) to account for set-up errors and residual motion effects. No standard modifications were made during treatment planning to account for the gold fiducial markers. All patients were treated with double-scattering proton therapy. Three-dimensional beam arrangements were custom designed for each patient to deliver highly conformal prescription dose distributions. Typically, 3–4 (non-overlapping on the skin) beams were used.
Treatment details
Patients received 10 fractions of proton therapy with 6 GyRBE per fraction. The dose distribution was normalized so that 99% of the ITV was covered by the prescription isodose surface, and the ITV received a minimum of 95% of the prescription dose. At least 85% of the PTV was covered by the prescription isodose surface. Dose-volume histograms were generated for all target volumes and normal tissue regions for analysis. Normal tissue constraints are summarized in Supplemental Table 1.
All treatments were delivered at the University of Florida Proton Therapy Institute. Treatments were delivered on consecutive days, and all treatments were completed within 2–3 weeks. Daily image guidance was utilized with fiducial markers and double exposure of orthogonal kilovoltage imaging at the peaks of inspiration and expiration. Cone-beam CT (CBCT) was not utilized, as our radiation gantries were not equipped with CBCT capabilities. The fiducial marker volume, drawn at the time of simulation based on the MIP image, was expanded 2 mm (fiducial + 2mm) and superimposed on the daily kV imaging. Adjustments to patient positioning were made to ensure that on inspiration and expiration the markers fell within the fiducial + 2-mm volume. Patients also underwent verification CT scans on days 1, 2, 4, and 6 of treatment to confirm appropriate alignment. During the course of radiation therapy, patients were assessed by the treating physician weekly, and their toxicities were scored using the Common Terminology Criteria for Adverse Events, version 4.0 [Citation10].
Surveillance
Following treatment completion, patients typically underwent follow-up at 1 month, then every 3 months for 1 year, and then every 6 months for 4 years. Patients underwent surveillance CT scan of the chest and PET-CT if disease progression was suspected. A local recurrence was defined as a recurrence or persistence of disease at the edge of the radiation field or within the same lobe. A regional recurrence was defined as disease recurrence in the mediastinal or other at-risk lymphatic regions.
Statistical analysis
All statistical analysis was conducted using SAS version 9.4 (SAS Institute, Cary, NC). Overall survival was estimated with the Kaplan–Meier product limit method. To adjust for the competing risk of intercurrent death, estimates of local recurrence, regional recurrence, and distant recurrence were instead estimated with the cumulative incidence method using the CIF macro in SAS.
Results
Patient, tumor, and treatment characteristics
Patient-, cancer-, and treatment-specific characteristics are summarized in . Twenty-two patients (96%) had a smoking history, with a median pack-year history of 60 (range, 0–150). Four patients (17%) had a prior diagnosis of NSCLC; 3 of these patients underwent prior lobectomy for treatment and 1 underwent a wedge resection. Another (4%) patient underwent wedge resection for the treatment of a lung infection 30 years prior to her primary diagnosis of lung cancer. In total, 24 tumors were treated; 1 patient (4%) had one tumor in each lung. The most common subtype of NSCLC was adenocarcinoma (46%) followed by squamous cell carcinoma (42%). Forty-six percent of tumors were classified as T1, and 54% of tumors were classified as T2 per the 8th edition of the American Joint Committee on Cancer staging manual.
Table 1. Patient and atumor characteristics (n = 23, with 24 tumors).
All patients were treated with 60 GyRBE in 10 fractions. The median duration of radiation therapy was 14 days (range, 10–16 days). No patients underwent elective regional nodal irradiation or treatment with systemic therapies as a part of their initial treatment course.
Disease control
At the time of analysis, 12 patients (52%) were alive. The median follow-up was 3.2 years for all patients (range, 0.2–9.2 years) and 3.2 years (range, 1.2–9.2 years) for living patients. No patients were lost to follow-up. The overall survival rates at 3 and 5 years were 81% and 50% (95% CI, 27%−79%), respectively (). The cause-specific survival rates at 3 and 5 years were 81% and 71% (95% CI, 46–92%), respectively (). The 3-year local, regional, and distant control rates were 90%, 81%, and 87%, respectively ().
Figure 1. Cumulative incidence rates of (A) overall survival, (B) cause-specific survival, (C) local control, (D) regional control, (E) freedom from distant metastasis, and (F) freedom from lung cancer after adjusting for competing risk events.
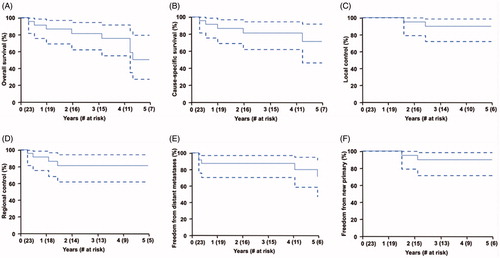
In total, 9 patients (39%) had experienced a disease recurrence as of the last follow-up: 3 (13%) had a local recurrence as their first recurrence, 4 (17%) had a regional recurrence as their first recurrence, and 2 (9%) had distant recurrence as their first recurrence. The median time to the first recurrence was 12 months (range, 2–61 months). Two of the 9 patients (22%) with recurrences were alive as of their last follow-up. The median survival after detection of recurrence was 6.4 months (range, 0.4–85.5 months). At 5 years, freedom from a new primary lung cancer was 90% (95% CI, 71–98%). Freedom from any lung cancer (primary, recurrent, or new lung cancer) was 52% (95% CI, 32−75%) at 5 years.
Local recurrences
Three patients (13%) experienced local recurrences as their first recurrence. Two patients were diagnosed radiographically, while 1 had a biopsy-proven recurrence. The median time from the development of a local recurrence was 28 months from radiotherapy completion (range, 18–61 months). Of these 3 patients, 2 had an in-field recurrence only and 1 had an in-field recurrence and development of satellite nodules. One of the 3 patients underwent retreatment with conventionally fractionated proton radiation and died from preexisting cardiac disease 10 months after completing reirradiation. The second patient underwent treatment with systemic therapy and is living 2 months after starting treatment. The third patient did not receive further treatment and died 3 months after detection of a recurrence due to progressive disease.
Regional recurrences
Four patients (17%) experienced a regional recurrence (mediastinal adenopathy) as a first recurrence. The median time to development of a regional recurrence was 8.5 months from radiotherapy completion (range, 2–17 months). One patient underwent treatment with systemic therapy, but died of unrelated cardiac disease 6 months later. Another patient underwent treatment with conventionally fractionated radiation with concurrent chemotherapy and died 46 months after the recurrence. A third patient did not receive further treatment and died several weeks after detection of a recurrence due to progressive disease and liver failure. One patient is living free of disease 86 months after the detection of regional recurrence, receiving conventionally fractionated radiation with protons with chemotherapy. The median survival time after the detection of a regional recurrence was 26 months (range, 0.4–85.5 months).
New primaries
Three patients (13%) developed new primaries during their follow-up period. All 3 patients received SBRT with photons for their new primaries. Two of the 3 patients were alive at 8 and 38 months after detection of the new primary. The third patient died from a mechanical fall 34 months after detection of a new primary.
Toxicities
One patient (4%) had a grade 3 acute toxicity unrelated to radiotherapy. This patient developed deep vein thrombosis (DVT) in the lower extremity following a 12-hour car ride. The etiology of this DVT is thought to be due to venous stasis and a hypercoaguable state from the patient’s malignancy. This DVT eventually migrated to the pulmonary vasculature and resulted in a pulmonary embolus. Consequently, the patient developed grade 3 hypoxia. No patients experienced grade 4 or 5 acute toxicities. Acute toxicity data are summarized in .
Table 2. Non-hematologic acute toxicities (n = 23).
In total, there were 2 patients (9%) with grade 3 late toxicities. One patient (4%) experienced a serious adverse event in the form of a late grade 3 bronchial stricture, which required bronchoplasty, endobroncial stent placement, and several balloon dilations. This patient had the largest PTV in the cohort, with a volume of 211 cm3. One patient (4%) experienced late grade 3 hypoxia following the development of the previously pulmonary embolism unrelated to radiotherapy. No patients experienced late esophageal ulceration, hemorrhage, or radiation-induced malignancy. Late toxicities are summarized in .
Table 3. Non-hematologic late toxicities (n = 23).
Dosimetry
The median PTV size was 72.6 cm3 (range, 14.5–211 cm3). Dosimetric data for critical normal structures, which are summarized in , reveal limited exposure to the esophagus, heart, non-target lung, spinal cord, ipsilateral proximal bronchial tree, and chest wall.
Table 4. Dosimetric data for critical structures.
Discussion
The treatment of centrally-located tumors in patients with medically inoperable NSCLC can result in significant morbidity due to the high doses of radiation required to achieve local control in an area constrained by sensitive anatomy. A systematic review of 563 patients with centrally located NSCLC treated with SBRT found a treatment-related mortality rate of 2.7%, with 9% of patients experiencing grade 3 or 4 toxicities [Citation11]. More recent studies have reported grade 5 toxicities as high as 7.5% [Citation12], and up to 21% for ‘ultra-central’ tumors [Citation13]. Further complicating the matter is that these studies employ different dose schemes with different BED10 values, and often define ‘centrally-located’ differently.
Compared to hypofractionated photon or X-ray-based treatment, hypofractionated proton therapy has the potential to reduce treatment-related toxicities due to its favorable dose distributions [Citation7]. In a previously published dosimetric comparative study, double-scatter proton therapy for peripheral early-stage NSCLC significantly reduced the dose to critical normal structures [Citation8]. It would stand to reason, then, that there is more to gain from the dosimetric advantages offered by proton therapy if used to treat centrally-located tumors, rather than small peripheral tumors [Citation14]. Though a comparison between the costs of proton and photon therapy is beyond the scope of this study, the cost difference between photon and proton treatment for stage I NSCLC with protons is relatively small, and thus, cost may not necessarily be a prohibitive factor in utilizing proton therapy [Citation15].
In our study, we found that the rate of non-hematologic toxicities using proton therapy for centrally-located tumors was acceptably low. No patients experienced grade 4 or 5 toxicities related to radiation, and none experienced esophageal ulceration, or hemorrhage. Two of three grade 3 and higher toxicities were considered unrelated to radiotherapy. A comparison of toxicities in our cohort to those in previously published series is difficult since the method of tabulating and interpreting toxicity varies across studies. However, our rate of toxicity seems to compare favorably to historic photon series, wherein grade 3 and higher toxicities have ranged from 0 to 28%, including some with grade 5 toxicities () [Citation5,Citation6,Citation9,Citation12,Citation16–26]. Published toxicity data from the NRG/RTOG 0813 trial demonstrated a greater rate of grade 3 and higher toxicities than among our cohort [Citation9], but their fractionation schemes were more aggressive than our own. Our toxicity data are comparable to previously published proton experiences from investigators at Loma Linda University [Citation18] and the Southern Tohoku Proton Therapy Center in Japan [Citation27]. Reducing treatment-related toxicities for centrally-located tumors is of critical importance, especially in patients who are too frail for surgery at baseline; proton therapy appears to offer this opportunity.
Table 5. Review of published literature that studied SBRT for centrally-located early-stage NSCLC.
While the overall survival rate at 5 years was 50%, the cause-specific survival rate was 71%, which reflects a cohort that is elderly in age, with several competing comorbidities. Our overall survival rate compares favorably to the published literature [Citation5,Citation6,Citation9,Citation12,Citation16–26], as shown in . Our local control rate was 90% at 3 years, which is comparable to the 3-year local control rate reported in the recently published NRG/RTOG 0813 study [Citation9], which reported 3-year local control rates of 86.7% and 84.7% in the high-dose arms. Our rates of local control were higher than those reported by investigators at the Southern Tohoku Proton Therapy Center, who observed a 2-year local control rate of 78.5% [Citation27]. It is worth noting that the lower rates of overall survival in other published studies may have artificially increased their rate of local control. Despite excellent local control in our study, regional recurrences, distant recurrences, and new primaries, remain challenges with early-stage NSCLC. Since the primary treatment for these patients consisted of highly conformal proton therapy plans, we were able to safely re-treat several patients who experienced localized recurrences. Nonetheless, this issue still plagues an otherwise very effective treatment.
Our study is not without its limitations. Since we did not exclude prior lung cancer diagnoses, it may be that some of the lesions treated as a new primary tumor were actually metastatic disease, thereby confounding our results. Another limitation was the relatively small number of patients in our series. With a larger cohort, we may have seen a grade 5 toxicity, as many of the photon-based studies with larger study sizes did. Despite our smaller cohort, because the literature regarding proton therapy for centrally-located tumors is scant, we believe our data nevertheless contribute valuable information to the literature. Moreover, our patients were treated using gold fiducials, which could have cast a shadow, resulting in underdosing of cells adjacent to the fiducial. Another limitation was that our image guidance was based on the orthogonal X-ray-based image guidance rather than CBCT-based image guidance, which is the standard for SBRT at many institutions today. Fortunately, CBCT scanning has become available at our institution and is available at most modern proton centers.
Overall, our results with hypofractionated proton therapy for centrally-located tumors are encouraging. We achieved excellent local control with few toxicities in a patient population that is prone to experiencing toxicity. The high conformality of proton therapy may allow for the use of dose-escalated regimens, which has already been shown to be more efficacious than lower-dose treatments [Citation18]. We anticipate that as more proton centers open and gain expertise in delivering SBRT, central tumors will be treated more frequently with proton therapy. While a randomized trial of SBRT with photons versus SBRT protons or hypofractionated protons would be a reasonable study, a recent trial like this was closed because of poor accrual [Citation28].
Supplemental Material
Download MS Word (12.6 KB)Acknowledgements
We would like to acknowledge the James E. Lockwood Jr Foundation and the following individuals for their involvement: Renard Seals, Stephanie Smith, Stephen Sibert, Lisa Ward, Julia Brumfield, Keri Hopper, Jeff Glidden, Nataliya Getman, Debbie Louis, Samatha Lambert, Jin Park, Valerie Fergusson, Jessica Kirwan, and Christopher Stich.
Disclosure statement
BSH is a Scientific Consultant for Merck & Co., Inc., and Bristol-Myers Squibb.
References
- Ball D, Mai GT, Vinod S, et al. Stereotactic ablative radiotherapy versus standard radiotherapy in stage 1 non-small-cell lung cancer (TROG 09.02 CHISEL): a phase 3, open-label, randomised controlled trial. Lancet Oncol. 2019;20(4):494–503.
- Timmerman RD, Hu C, Michalski J, et al. Long-term results of RTOG 0236: a phase II trial of stereotactic body radiation therapy (SBRT) in the treatment of patients with medically inoperable stage i non-small cell lung cancer. Int J Radiat Oncol. 2014;90(1):S30.
- Haasbeek CJ, Lagerwaard FJ, Antonisse ME, et al. Stage I nonsmall cell lung cancer in patients aged > or =75 years: outcomes after stereotactic radiotherapy. Cancer. 2010;116(2):406–414.
- van der Voort van Zyp NC, Prevost JB, Hoogeman MS, et al. Stereotactic radiotherapy with real-time tumor tracking for non-small cell lung cancer: clinical outcome. Radiother Oncol. 2009;91(3):296–300.
- Timmerman R, McGarry R, Yiannoutsos C, et al. Excessive toxicity when treating central tumors in a phase II study of stereotactic body radiation therapy for medically inoperable early-stage lung cancer. JCO. 2006;24(30):4833–4839.
- Fakiris AJ, McGarry RC, Yiannoutsos CT, et al. Stereotactic body radiation therapy for early-stage non-small-cell lung carcinoma: four-year results of a prospective phase II study. Int J Radiat Oncol Biol Phys. 2009;75(3):677–682.
- Chang JY, Zhang X, Wang X, et al. Significant reduction of normal tissue dose by proton radiotherapy compared with three-dimensional conformal or intensity-modulated radiation therapy in stage I or stage III non-small-cell lung cancer. Int J Radiat Oncol Biol Phys. 2006;65(4):1087–1096.
- Hoppe BS, Huh S, Flampouri S, et al. Double-scattered proton-based stereotactic body radiotherapy for stage I lung cancer: a dosimetric comparison with photon-based stereotactic body radiotherapy. Radiother Oncol. 2010;97(3):425–430.
- Bezjak A, Paulus R, Gaspar LE, et al. Safety and efficacy of a five-fraction stereotactic body radiotherapy schedule for centrally located non-small-cell lung cancer: NRG oncology/RTOG 0813 trial. JCO. 2019;37(15):1316–1325.
- U.S. Department of Health and Human Services. Common terminology criteria for adverse events (CTCAE). 2010. Available from: https://ctep.cancer.gov/protocolDevelopment/electronic_applications/ctc.htm
- Senthi S, Haasbeek CJ, Slotman BJ, et al. Outcomes of stereotactic ablative radiotherapy for central lung tumours: a systematic review. Radiother Oncol. 2013;106(3):276–282.
- Tekatli H, Senan S, Dahele M, et al. Stereotactic ablative radiotherapy (SABR) for central lung tumors: plan quality and long-term clinical outcomes. Radiother Oncol. 2015;117(1):64–70.
- Tekatli H, Haasbeek N, Dahele M, et al. Outcomes of hypofractionated high-dose radiotherapy in poor-risk patients with ‘ultracentral’ non-small cell lung cancer. J Thorac Oncol. 2016;11(7):1081–1089.
- Georg D, Hillbrand M, Stock M, et al. Can protons improve SBRT for lung lesions? Dosimetric considerations. Radiother Oncol. 2008;88(3):368–375.
- Peeters A, Grutters JP, Pijls-Johannesma M, et al. How costly is particle therapy? Cost analysis of external beam radiotherapy with carbon-ions, protons and photons. Radiother Oncol. 2010;95(1):45–53.
- Olsen JR, Robinson CG, El Naqa I, et al. Dose-response for stereotactic body radiotherapy in early-stage non-small-cell lung cancer. Int J Radiat Oncol Biol Phys. 2011;81(4):e299–303.
- Nuyttens JJ, van der Voort van Zyp NC, Praag J, et al. Outcome of four-dimensional stereotactic radiotherapy for centrally located lung tumors. Radiother Oncol. 2012;102(3):383–387.
- Bush DA, Cheek G, Zaheer S, et al. High-dose hypofractionated proton beam radiation therapy is safe and effective for central and peripheral early-stage non-small cell lung cancer: results of a 12-year experience at Loma Linda University Medical Center. Int J Radiat Oncol Biol Phys. 2013;86(5):964–968.
- Chang JY, Li QQ, Xu QY, et al. Stereotactic ablative radiation therapy for centrally located early stage or isolated parenchymal recurrences of non-small cell lung cancer: how to fly in a ‘no fly zone. Int J Radiat Oncol Biol Phys. 2014;88(5):1120–1128.
- Modh A, Rimner A, Williams E, et al. Local control and toxicity in a large cohort of central lung tumors treated with stereotactic body radiation therapy. Int J Radiat Oncol Biol Phys. 2014;90(5):1168–1176.
- Park HS, Harder EM, Mancini BR, et al. Central versus peripheral tumor location: influence on survival, local control, and toxicity following stereotactic body radiotherapy for primary non-small-cell lung cancer. J Thorac Oncol. 2015;10(5):832–837.
- Chaudhuri AA, Tang C, Binkley MS, et al. Stereotactic ablative radiotherapy (SABR) for treatment of central and ultra-central lung tumors. Lung Cancer. 2015;89(1):50–56.
- Schanne DH, Nestle U, Allgauer M, et al. Stereotactic body radiotherapy for centrally located stage I NSCLC: a multicenter analysis. Strahlenther Onkol. 2015;191(2):125–132.
- Ma SJ, Syed YA, Rivers CI, et al. Comparison of single- and five-fraction schedules of stereotactic body radiation therapy for central lung tumours: a single institution experience. J Radiother Pract. 2017;16(2):148–154.
- Verma V, Shostrom VK, Zhen W, et al. Influence of fractionation scheme and tumor location on toxicities after stereotactic body radiation therapy for large (>/=5 cm) non-small cell lung cancer: a multi-institutional analysis. Int J Radiat Oncol Biol Phys. 2017;97(4):778–785.
- Lindberg K, Bergström P, Brustugun OT, et al. OA24.05 The Nordic HILUS-Trial – first report of a phase II trial of SBRT of centrally located lung tumors. J Thoracic Oncol. 2017;12(1):S340.
- Ono T, Yabuuchi T, Nakamura T, et al. High dose hypofractionated proton beam therapy is a safe and feasible treatment for central lung cancer. Radiol Oncol. 2017;51(3):324–330.
- Nantavithya C, Gomez DR, Wei X, et al. Phase 2 study of stereotactic body radiation therapy and stereotactic body proton therapy for high-risk, medically inoperable, early-stage non-small cell lung cancer. Int J Radiat Oncol Biol Phys. 2018;101(3):558–563.