Abstract
Background
High-throughput sequencing technologies are increasingly used in research but limited data are available on the feasibility and value of these when routinely adopted in clinical practice.
Material and methods
We analyzed all consecutive cancer patients for whom genomic testing by a 48-gene next-generation sequencing (NGS) panel (Truseq Amplicon Cancer Panel, Illumina) was requested as part of standard care in one of the largest Belgian cancer networks between 2014 and 2019. Feasibility of NGS was assessed in all study patients, while the impact of NGS on the decision making was analyzed in the group of gastrointestinal cancer patients.
Results
Tumor samples from 1064 patients with varying tumor types were tested, the number of NGS requests increasing over time (p < .0001). Success rate and median turnaround time were 91.4% and 12.5 days, respectively, both significantly decreasing over time (p ≤ .0002). Non-surgical sampling procedure (OR 7.97, p < .0001), tissue from metastatic site (OR 2.35, p = .0006) and more recent year of testing (OR 1.79, p = .0258) were independently associated with NGS failure. Excluding well-known actionable or clinically relevant mutations which are recommended by international guidelines and commonly tested by targeted sequencing, 57/279 (20.4%) assessable gastrointestinal cancer patients were found to have tumors harboring at least one actionable altered gene according to the OncoKB database. NGS results, however, had a direct impact on management decisions by the treating physician in only 3 cases (1.1%).
Conclusions
Our findings confirm that NGS is feasible in the clinical setting with acceptably low failure rates and rapid turnaround time. In gastrointestinal cancers, however, NGS-based multiple-gene testing adds very little to standard targeted sequencing, and in routine practice the clinical impact of NGS panels including genes which are not routinely recommended by international guidelines remains limited.
Introduction
Over the last few decades, significant progress has been made in terms of understanding the biological processes underlying carcinogenesis, tumor growth, progression and resistance to treatment [Citation1,Citation2]. Tumor molecular characterization has increasingly been refined, and a number of genomic alterations have been identified that can act as either prognostic/predictive biomarkers or therapeutic targets [Citation3]. Recently, these advances have been accelerated by the development of sophisticated, high-throughput technologies such as massively parallel sequencing which allows comprehensive molecular profiling [Citation4,Citation5].
While these technologies have revolutionized clinical and translational research and paved the way for genomic-based medicine and precision oncology, their impact on routine decision making remains unclear. Large studies suggest the existence of a mismatch between the informative potential of next-generation sequencing (NGS) techniques and the resulting practical implications of their molecular data output. Clinical decisions are driven by the results of NGS in a minority of cases only. Even in such circumstances, the added clinical value of large-scale NGS panels is questionable, as many of the actionable genomic alterations are usually detected by less sophisticated, routine techniques such as conventional targeted sequencing. Finally, interpretation of NGS findings by the treating physicians may be challenging [Citation6], and guidelines for the design, development and analytical validation of NGS-based tests have only recently been issued by the US Food and Drug Administration (FDA) in relation to the diagnosis of suspected germline diseases [Citation7].
Despite these considerations, NGS tests have gained traction among oncologists and have increasingly been requested in routine practice. According to the US National Survey of Precision Medicine in Cancer Treatment, 76% of oncologists and hematologists in 2016–2017 used data from at least one NGS test to inform treatment decisions [Citation8]. NGS tests were especially requested for patients with advanced refractory tumors, and most commonly used to guide the use of FDA-approved drugs and to assess patient eligibility for clinical trials. Less often, NGS testing was justified by the intention to use off-label drugs or need to get diagnostic/prognostic information.
In Belgium, since July 2019 genomic analysis by NGS has been reimbursed as part of a pilot study to introduce novel sequencing technologies into the routine diagnostics in oncology and hematology. A national consortium of experts set out the framework of this initiative providing a list of clinically relevant genes related to diagnosis, treatment response, or prognosis for each clinical indication. Before July 2019, no reimbursement was available but central laboratories of cancer networks were accredited to carry out tumor molecular profiling by NGS at the request of the treating physician. Once available, results were reported back to the requesting physician who could interpret and act on these, generally after discussing the patient case at the local molecular tumor board and/or multidisciplinary team meeting.
We have analyzed the feasibility of routine use of a multi-gene NGS panel in one of the largest Belgian cancer networks during the 5 years before the start of the national pilot study, and assessed the impact that NGS data had on the clinical management of gastrointestinal cancer patients treated in a large Belgian tertiary center.
Material and methods
Eligibility and data collection
All consecutive patients for whom tumor genomic testing by a multi-gene NGS panel was requested from April 2014 to June 2019 as part of routine practice in the IRIS Network (a network of five hospitals in Brussels including Institut Jules Bordet, Saint-Pierre University Hospital, Brugmann University Hospital, Queen Fabiola Children’s University Hospital and IRIS Hospitals South) were retrospectively identified from a prospectively maintained database.
For each patient, data were collected including date of analysis, tumor type, site of origin of the sample, sampling procedure, tissue/test quality measures, mutations detected, turnaround time and reasons for failure. Also, for the group of patients with gastrointestinal cancers who were managed at the Institut Jules Bordet, additional clinical information was collected to analyze how the NGS findings influenced management decisions. The decision to limit this analysis to gastrointestinal cancers was made upfront, and it was pragmatically based on the specific research interest of the main study promoter (FS).
NGS testing
The molecular pathology laboratory at the Department of Pathology of the Institut Jules Bordet was the reference laboratory of the IRIS Network. Tumor sample preparation and sequencing analysis were conducted according to the local standard operating procedures and the NGS assay manufacturer’s instructions.
Formalin-fixed paraffin-embedded (FFPE) samples were used. Before DNA extraction, enrichment of the tumoral content was done by microdissection of the region of interest on hematoxylin and eosin (HE) slide. DNA was extracted from 5 sections of 7 µm by using QIAamp DNA FFPE Tissue Kit (Qiagen, Hilden, Germany). The samples were deparaffinised with Toluene. The digestion by proteinase K was performed at 56 °C during 1 h. Incubation during 1 h at 90 °C partially reversed formaldehyde modification of nucleic acids. The resulted lysate was transferred on silica-based column to perform the purification steps. DNA was eluted with 50 µl nuclease-free water. DNA was quantified by Qubit (Qubit™ dsDNA HS Assay Kit, ThermoFisher Scientific), and only samples with at least 12,5ng were suitable for analysis. DNA quality was evaluated by real time PCR (Infinium HD FFPE QC Assay, Illumina), and low DNA quality samples were excluded (ΔCt ≥4).
The Truseq Amplicon Cancer Panel (Illumina) was used over the study period. This is an amplicon-based multiplexed targeted resequencing assay that allows the detection of mutations in hotspot regions of the following 48 cancer-related genes: ABL, AKT, ALK, APC, ATM, BRAF, CDH1, CDKN2A, CSF1R1, CTNNB1, EGFR, ERBB2, ERBB4, FBXW7, FGFR-1, FGFR-2, FGFR-3, FLT-3, GNA11, GNAQ, GNAS, HNF1A, HRAS, IDH-1, JAK-2, JAK-3, KDR, KIT, KRAS, MET, MLH1, MPL, NOTCH-1, NPM1, NRAS, PDGFRA, PIK3CA, PTEN, PTPN11, RB-1, RET, SMAD-4, SMARCB1, SMO, SRC, STK11, TP53, VHL. The protocol for the NGS library preparation is available in the supplementary files.
The methodology for the classification of pathogenic variants as well as the databases used to check for pathogenic alterations changed over the study period. The algorithm used at the time of the analysis was the one developed by the Belgian ComPerMed Expert Panel in 2019 [Citation9]. According to this, all mutations with an allelic frequency of at least 5% and a coverage depth of ≥1000× were reported while mutations with an allelic frequency comprised between 3% and 5% or a depth coverage between 400× and 1000× were reported only if they had a clinical implication based on the College of American Pathologists (CAP) guidelines [Citation10]. Variants with an allelic frequency of at least 1% in ExAC and 1000 Genomes databases were considered as polymorphisms and not reported [Citation11,Citation12].
Study objectives
The primary objective of the study was to evaluate the feasibility of using a cancer hotspot NGS panel in routine practice. This was assessed by analyzing the proportion of patients who were successfully tested and the median turnaround time (as indicated by the time between reception of the request by the laboratory and report of the results). Secondary objectives were to analyze possible variables that affected NGS performance in the entire study population, to assess the mutational profiling of gastrointestinal cancers, and to evaluate the impact that an NGS panel that included genes which are not routinely recommended by international guidelines had on management decisions. Exploratory objectives included the analysis of the association between mutational data and clinico-pathological characteristics of gastrointestinal cancer patients.
Definition of clinical impact and actionability
Results of NGS were considered as having impacted on clinical practice if they prompted the physician to start/change treatment, to consider therapy with novel agents through early access schemes/compassionate use programs, or to propose screening for a clinical trial. The OncoKB levels of evidence were used to categorize somatic mutations according to their level of actionability (https://www.oncokb.org) (Supplementary files) [Citation13]. To further evaluate the clinical impact of the mutations detected by NGS, we hypothesized that all study patients had been tested in November 2019 and the Clinical Trials Transformation Initiative registry [Citation14] (accessed on 21 November 2019) was interrogated to search for potential clinical trial opportunities. For the analyses of clinical impact and actionability, tumor-specific genes which are recommended to be tested in routine practice by international guidelines and generally tested by targeting sequencing were not considered.
Statistical considerations
Statistical analyses were performed using R Version 3.6.1. The univariate significance of the association between variables was assessed by the χ2 test, and considered to be present when p < .05. Multivariable analysis was performed using logistic regression. The turnaround time was compared using the Mann–Whitney test. Other statistics were descriptive.
Reporting guidelines
Study design, conduct and findings were reported in accordance with the STROBE cross-sectional study reporting guidelines [Citation15]. The completed checklist can be found in the supplementary files.
Study approval
The study was approved by the Institutional Review Board at the Institut Jules Bordet and an independent Ethics Committee (CE3035). Given the design of the study, no informed consent from patients was needed.
Results
Between April 2014 and June 2019, 1064 NGS requests were received for patients with gastrointestinal cancers (42.0%), melanoma (15.2%), non-small cell lung cancer (NSCLC) (15.1%), genitourinary cancers (10.1%), breast cancer (6.7%) and other tumors (10.9%, each other tumor type accounting for <5% of total requests) (). Testing increased gradually over time with 472 requests made from April 2014 to October 2016 and 592 from November 2016 to June 2019 (p < .0001) ().
Figure 1. Study flow diagram showing number and type of NGS tests requested in the IRIS network between 2014 and 2019. BC: breast cancer; GI: gastrointestinal; GU: genitourinary; HPB: hepato-biliary-pancreatic; IJB: Institut Jules Bordet; NGS: next-generation sequencing; NSCLC: non-small cell lung cancer.
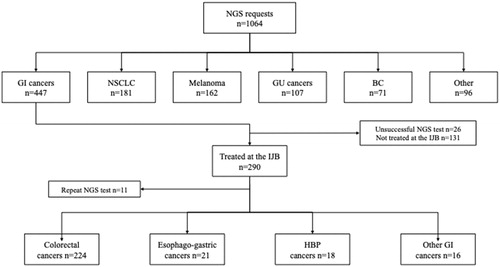
Figure 2. Requests of NGS tests by tumor type received each year by the molecular pathology laboratory at the Institut Jules Bordet over the study period. BC: breast cancer; GI: gastrointestinal cancers; GU: genitourinary cancers; NSCLC: non-small cell lung cancer.
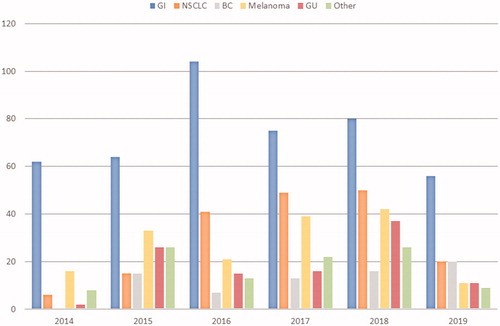
Feasibility of multi-gene NGS in the entire study cohort
A total of 972 (91.4%) tests were successfully carried out. The median turnaround time across the study period was 12.5 days, this reducing from 17 days in 2014 to 10 days in 2019 (p < .0001) (). The success rate dropped gradually over time from 97.8% in 2014 to 86.6% in 2019 (χ2 for trend 14.00, p = .0002) (). Test failures occurred in 92 (8.6%) cases. These included 16/71 breast cancers (29.1%), 29/181 NSCLCs (19.1%), 26/447 gastrointestinal cancers (6.2%), 6/107 genitourinary cancers (5.9%), 8/162 melanomas (5.2%), and 7/96 other tumors (7.3%) (χ2 38.63, p = .0002). Reasons for failure included poor quality/low quantity of DNA (58.7%), insufficient tissue sample (23.9%), lack of adequate tumor cellularity (12.0%), tumor tissue exhaustion due to previous analyses (4.3%), and unknown causes (1.1%). Reasons for failure by tumor type are reported in Supplementary Table 1. The mean time from tumor sampling to non-contributive NGS analysis due to poor quality/low quantity of DNA was 22.2 months, as compared with 6.9 months for all other causes of unsuccessful testing (p = .028).
Figure 3. Turnaround time (days) of the 48-gene Truseq Amplicon Cancer Panel by year over the study period (calculated as the time between reception of test request and report of the results).
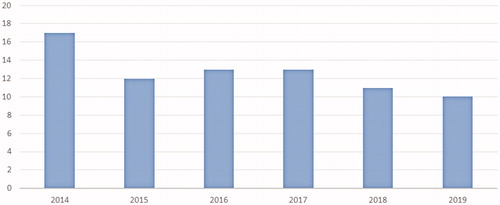
Sample collection was performed by surgery in 480 cases (45.1%), core biopsy in 398 (37.4%), and fine needle aspiration (FNA) in 28 (2.6%). Sequencing failed in 2.5% of surgical samples, 16.3% of biopsy samples, and in 35.7% of FNA samples (χ2 70.65, p < .0001). The source of DNA used for sequencing was the primary tumor for 502 (47.2%) patients and a metastasis for 411 (38.6%) patients. Primary tumor samples were successfully analyzed in 94.0% of cases, as compared with 86.1% of samples from metastatic lesions (χ2 39.91, p = .0001). Out of the 411 samples harvested from metastatic lesions, 103 (25.1%) were nodal metastases, 82 (19.9%) hepatic, 62 (15.1%) cutaneous/subcutaneous, 49 (11.9%) peritoneal, 19 (4.6%) bone, 18 (4.4%) lung, and 78 (19.0%) other metastases. The rate of NGS failure appeared lower for samples from cutaneous/subcutaneous (1.6%), and peritoneal (6.1%) metastases, as compared with those from nodal (14.6%), lung (27.8%), liver (20.7%), bone (15.8%), and other (16.7%) metastases.
When the above mentioned significant variables were assessed in multivariable analysis, non-surgical sampling procedure (odds ratio [OR] 7.97, 95% CI 4.24–14.97, p < .0001), tissue from metastatic site (OR 2.35, 95% CI 1.44–3.84, p = .0006) and more recent testing (i.e., after October 2016) (OR 1.79, 95% CI 1.07–2.98, p = .0258) remained significantly associated with an increased probability of NGS failure ().
Table 1. Association between variables and NGS success in the study population.
Results of NGS analysis in the gastrointestinal cancer study cohort
Overall, 421 out of 972 (43.3%) NGS tests were done on gastrointestinal cancer samples. Of these, 290 (68.9%) were from patients who were managed at the Institut Jules Bordet and for whom clinical data were available. Eleven patients had repeat NGS testing, this leaving 279 patients assessable for analysis. Tumor distribution was as follows: 224 colorectal (80.3%), 21 esophago-gastric (7.5%), 18 hepato-biliary-pancreatic (6.5%), 9 gastrointestinal stromal (GIST) (3.2%), 4 appendiceal (1.4%), and 3 small bowel cancers (1.1%). The vast majority of tests (244/279, 87.5%) were requested for patients with metastatic disease.
Across all gastrointestinal tumor types, TP53 was the most frequently mutated gene (45.9%), followed by APC (42.1%), KRAS (39.7%), PIK3CA (12.1%), SMAD4 (7.6%), BRAF (6.2%), and NRAS (5.5%) (Supplementary Figure 1). In the colorectal cancer cohort, mutations occurred most commonly in APC (52.2%), KRAS (43.8%), TP53 (43.3%), PIK3CA (14.4%), BRAF (8.0%), SMAD4 (7.5%), and NRAS (6.5%). shows the distribution and proportion by bowel segment of all mutations detected in colorectal cancer patients (n = 222). In the subgroup of patients with metastatic disease (n = 199), KRAS mutations were associated with lung (p = .020) and bone metastases (p = .008), NRAS mutations with liver metastases (p = .021), BRAF mutations with peritoneal metastases (p = .001), and APC mutations with lymph node metastases (p = .006).
Figure 5. Distribution and proportion by bowel segment of all mutations detected by the 48-gene Truseq Amplicon Cancer Panel in colorectal cancer patients who were managed at the Institut Jules Bordet over the study period (n = 222). The percentages next to each spot indicate the proportion of tumors harboring given mutations within each segment of the bowel.
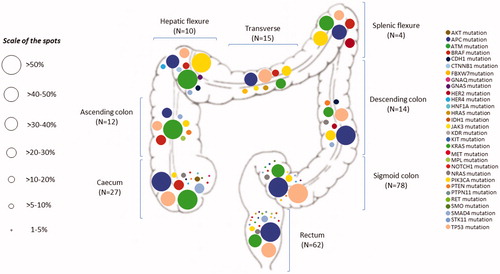
Clinical implications of NGS analysis in the gastrointestinal cancer study cohort
In 3 out of 279 (1.1%) gastrointestinal cancer patients who underwent a successful NGS analysis, the results of the NGS test had a direct impact on management decisions by the treating physician, providing information that would not have been revealed by tumor-specific standard targeted sequencing based on recommendations from international guidelines. In a cholangiocarcinoma patient, an IDH1 mutation was found which contributed to differential diagnosis and led to screening for a clinical trial. In two other cases, NGS allowed detecting rare variants of genes which were not previously searched for by targeted sequencing (KRASA146V and BRAFV590I, which supported the decision not to use anti-EGFR agents in two colorectal cancer patients).
Using the publicly available OncoKB database and excluding genes that are generally tested by targeted sequencing according to the recommendations from international guidelines (KRAS, NRAS, BRAF, KIT and PDGFRA), 57 out of the 279 patients (20.4%) had tumors harboring at least one actionable altered gene. When taking into account the tumor type in which the gene is known to be actionable, only 10 (3.6%) of the patients in the cohort had an altered gene that could be targeted. The highest level of evidence for an actionable alteration in our cohort (an IDH1R132C mutation in a cholangiocarcinoma patient) was 3 A. When we evaluated the clinical impact of the additional mutations detected by NGS by searching through the Clinical Trials Transformation Initiative registry, a genomically matched clinical trial could be offered to up to 65 patients (23.3%) if they had been tested in November 2019.
Discussion
In this study we have investigated the feasibility, performance and clinical implications of a tumor-agnostic NGS panel when used for routine genomic profiling in a large Belgian cancer network. Our findings confirm that NGS-based, multiple gene testing is feasible in the clinical setting, with acceptably low failure rates and rapid turnaround time. Nevertheless, genomic data that were additional to those recommended by international guidelines and generally provided by tumor-specific, targeted sequencing had virtually no impact on patient management.
Over the last decade, high-throughput technologies have dramatically changed cancer research and influenced oncology practice, allowing better understanding of tumor biology, detection of potential therapeutic targets and identification of mechanisms of response or resistance to treatment. NGS was first introduced in 2005 [Citation16], and since then its applications in both the research and clinical settings have rapidly increased as a result of technical improvements and reduction of costs [Citation17]. Nonetheless, some barriers still exist to its widespread use in clinical practice [Citation18]. In previous studies, failure rates have been reported to be as high as 22%, unsuccessful testing being often secondary to the poor quantity and/or quality of the tumor material, an issue that is especially relevant for certain tumor types that are less accessible and amenable to high-yielding sampling procedures [Citation19]. Furthermore, the question remains as to whether and to which extent a more comprehensive tumor genomic characterization informs clinical practice and guides management decisions. By using a sequencing platform including up to 410 recurrently altered cancer-related genes (MSK-IMPACT), investigators from the Memorial Sloan-Kettering Cancer Center prospectively analyzed 10,336 solid tumors from 62 cancer types. Thirty-seven percent of patients had potentially actionable alterations (including those that are routinely screened for by targeting sequencing and actioned with approved therapies), and only 11% were ultimately enrolled into genomically matched trials [Citation20]. Similar findings have been reported by other single-center studies investigating smaller NGS panels (i.e., up to 50 genes). In the setting of metastatic colorectal cancer, for instance, almost 60% of patients had targetable genomic alterations but only 3–6% of these were enrolled in biomarker-selected trials, and 0–17% achieved an objective response to treatment [Citation21,Citation22]. Despite encouraging results from some studies [Citation23], off-label use of targeted therapies based on matching molecular alterations did not appear to improve survival outcomes as compared to standard therapy, as shown by the randomized phase II SHIVA trial [Citation24].
While our study is limited by the relatively small sample size, especially if compared against previously published series, it has the merit of providing useful insights on the feasibility and clinical utility of tumor-agnostic NGS testing within the real-world setting of a cancer network. First of all, our data exclude major administrative or technical obstacles to the routine implementation of broader NGS panels in everyday clinical practice. The vast majority of patients could be successfully tested, genomic characterization being not possible in <9% of cases, a proportion that is in line with the failure rate of targeted sequencing techniques [Citation25,Citation26]. Importantly, the turnaround time decreased over time and the testing process reached efficiency levels that should meet the increased pressure from requesting physicians who may hold management decisions while waiting for the test results.
Previous studies have analyzed variables affecting the overall performance of NGS and reported site of sampled lesion, type of biopsy, number of cores and clinical setting (i.e., sampling at diagnosis or recurrence) as potential causes of failure [Citation19,Citation27,Citation28]. Similarly, we found that, as a likely result of the inadequate material yielded, sampling by biopsy/FNA and tumor material from certain metastatic sites (including, among others, lymph nodes, liver and lung) were predictors for NGS failure. It is interesting to note, and certainly counterintuitive, that year of testing was an independent predictive factor for NGS failure, with the success rate gradually reducing over time. These unexpected results are unlikely to be secondary to a reduced test performance, but they may actually reflect the growing interest for NGS from physicians and the resulting increased number of tests requested on tumor samples that did not meet the minimum quality standards. Not surprisingly, pre-analytical factors such as poor quality/quantity of the tumor tissue/extracted DNA, that appeared to be associated with longer intervals between tumor sampling and analysis, were the main cause of non-contributive NGS results.
As far as the clinical implications of using a broader NGS panel are concerned, the findings of our study are in line with previous reports and further support the contention that the potential of high-throughput NGS technologies is still largely unfulfilled. Only in 1% of cases, a more extended NGS analysis that would generally be recommended for clinical practice, revealed information that was additional to that provided by tumor-specific targeted sequencing, and had some impact on the patient management. In the majority of these cases, the additional information actually consisted of genetic variants of tumor-specific genes which were not routinely tested by standard sequencing techniques. Also, in relation to the case of the patient who had a BRAF V590I missense mutation, the functional effects of this alteration on the braf protein are largely unknown. Although this has been described as pathogenic in adenoid cystic carcinoma of the salivary gland [Citation29], it is debatable whether this information could have been used to inform prognosis or to decide on a standard therapy with anti-EGFR monoclonal antibodies or an investigational treatment with anti-BRAF agents. It is fair to note, however, that the limited clinical usefulness of our 48-gene NGS panel on the management of gastrointestinal cancer patients may just be the result of the scant availability and/or awareness of genomically matched clinical trials and the absence of a Tumor Molecular Board for part of the study period. Furthermore, while this is generally not a significant issue with relatively small NGS panels, possible cases of erroneous perception of genomic actionability and resulting risk of underestimation of the clinical relevance of some findings by the treating physicians cannot be excluded [Citation6]. Not surprisingly, when we checked all the genomic variants that were detected by the NGS panel in our study population (excluding established actionable genes such as KRAS, NRAS, BRAF, KIT and PDGFRA) against a universal database of currently available clinical trials, approximately one out of four patients could be proposed recruitment into a biomarker-driven investigational study. Also, in 1 out of 5 cases patients were found to have tumors harboring altered genes that, according to publicly available databases, are known or predicted to be targetable with anticancer agents.
Our study has a number of limitations including the retrospective design, the relatively small numbers, and the use of data dating back to the 2014–2019 period, which may not entirely reflect current practice. Also, the analysis of clinical implications of the routine use of a broader, non tumor-specific, NGS panel was restricted to the group of patients with gastrointestinal tumors who accounted for the relative majority of the study population. It cannot be ruled out that routine use of the same NGS panel could have a bigger impact on the management of patients with other tumors. Substantial heterogeneity has indeed been reported across tumor types with regard to the actionability of NGS-detected genetic variants, with thyroid, breast, glioma and endometrial cancers being those with the highest proportion of potentially actionable genomic alterations [Citation20]. Another limitation is that our NGS panel included only 48 genes. It is possible that use of a broader panel would have led to different results and possibly increased the clinical relevance of large-scale NGS testing. For instance, investigators of the MetAction study in Norway increased the proportion of refractory cancer patients with actionable alterations by replacing a 50-gene panel with a 143-gene panel that also allowed detection of copy number variants and gene fusion drivers [Citation30,Citation31]. Finally, it cannot be entirely ruled out that our study population was enriched for poor prognosis patients with limited chances to receive investigational therapies, especially if NGS testing was requested close to exhaustion of standard treatment options. This, however, is unlikely to apply to colorectal cancer patients whose tumors were molecularly characterized at the first evidence of metastatic disease, according to the general practice during the study period.
Precision oncology is a pressing need that requires healthcare stakeholders to keep pace with emerging technologies and rapidly adapt to new research models and care pathways. Massive amounts of raw genomic data are currently being generated for individual patients, and these are anticipated to exponentially increase in the near future by the use of more and more sophisticated sequencing techniques. As a result, researchers and physicians are faced with the challenge of transforming these data into tools for practical clinical applications. Nationwide genomic screening programs such as the SCRUM-Japan project provide a good example of how to meet this challenge by filling the gap between basic scientists and clinical researchers and implementing strong partnerships between academia and industry. It should be noted, however, that, while large-scale implementation of initiatives like this are expected to maximize the clinical impact of NGS-based tumor genomic profiling, integration of genomics with other omic technologies will be key to harnessing the power of cancer knowledge and make substantial advances toward a true care model of precision oncology.
Supplemental Material
Download MS Word (19.8 KB)Supplemental Material
Download MS Power Point (98.9 KB)Supplemental Material
Download MS Word (64.4 KB)Acknowledgments
The authors thank all the patients who were included in this study. The authors thank ESMO for supporting Dr. Giacomo Bregni.
Disclosure statement
Amelie Deleporte – Travel grants: Amgen, Ipsen. Martine Piccart-Gebhart – Board Member (Scientific Board): Oncolytics. Consultant (honoraria): AstraZeneca, Camel-IDS, Crescendo Biologics, Debiopharm, G1 Therapeutics, Genentech, Huya, Immunomedics, Lilly, Menarini, MSD, Novartis, Odonate, Periphagen, Pfizer, Roche, Seattle Genetics. Research funding: AstraZeneca, Lilly, MSD, Novartis, Pfizer, Radius, Roche-Genentech, Servier, Synthon. Ahmad Awada – Speakers, consultancy, advisory board: Amgen, BMS, EISAI, Leo Pharma, Lilly, MSD, Novartis, Pfizer, Roche. Research funding: BMS. Travel grants: Mylan, Pfizer, Roche. Alain Hendlisz – Consultancy, advisory board: Amgen, Bayer, Eli Lilly, Merck, Pierre Fabre, Servier, Sirtex. Research funding: Amgen, AstraZeneca, Ipsen, Leo Pharma, Merck, Roche, Sanofi, Teva Pharma. Travel grant: Merck, Roche, Sirtex. Philippe Aftimos – Consulting: Amcure, Boehringer Ingleheim, G1 Therapeutics Macrogenics, Novartis, Roche, Servier. Honoraria: Amgen, Novartis, Synthon. Travel grants: Amgen, MSD, Pfizer, Roche. Francesco Sclafani – Research funding: AstraZeneca, Bayer, BMS, Roche. Travel grants: Bayer, Lilly. All other authors do not have any conflicts of interests.
Additional information
Funding
References
- Hanahan D, Weinberg RA. Hallmarks of cancer: the next generation. Cell. 2011;144:646–674.
- Hawkins RD, Hon GC, Ren B. Next-generation genomics: an integrative approach. Nat Rev Genet. 2010;11:476–486.
- Berger MF, Mardis ER. The emerging clinical relevance of genomics in cancer medicine. Nat Rev Clin Oncol. 2018;15:353–365.
- Tucker T, Marra M, Friedman JM. Massively parallel sequencing: the next big thing in genetic medicine. Am J Hum Genet. 2009;85:142–154.
- Anderson MW, Schrijver I. Next generation DNA sequencing and the future of genomic medicine. Genes (Basel). 2010;1:38–69.
- Schram AM, Reales D, Galle J, et al. Oncologist use and perception of large panel next-generation tumor sequencing. Ann Oncol. 2017;28:2298–2304.
- U.S. Food and Drug Administration (FDA). Considerations for design, development, and analytical validation of next generation sequencing-based in vitro diagnostics intended to aim in the diagnosis of suspected germline diseases. [updated 2018 Apr 13]. Available from: https://www.fda.gov/downloads/MedicalDevices/DeviceRegulationandGuidance/GuidanceDocuments/UCM509838.pdf
- Froyen G, Le Mercier M, Lierman E, et al. Standardization of somatic variant classifications in solid and haematological tumours by a two-level approach of biological and clinical classes: an initiative of the belgian ComPerMed expert panel. Cancers (Basel). 2019;11:2030.
- Freedman AN, Klabunde CN, Wiant K, et al. Use of next-generation sequencing tests to guide cancer treatment: results from a nationally representative survey of oncologists in the United States. J Cin Oncol Precis Oncol. 2018.
- Li MM, Datto M, Duncavage EJ, et al. Standards and guidelines for the interpretation and reporting of sequence variants in cancer: a joint consensus recommendation of the Association for Molecular Pathology, American Society of Clinical Oncology, and College of American Pathologists. J Mol Diagn. 2017;19:4–23.
- Karczewski KJ, Weisburd B, Thomas B, et al.; The Exome Aggregation Consortium. The ExAC browser: displaying reference data information from over 60 000 exomes. Nucleic Acids Res. 2017;45:D840–D845.
- , Auton A, Brooks LD, Durbin RM, et al.; 1000 Genomes Project Consortium. A global reference for human genetic variation. Nature. 2015;526:68–74.
- Chakravarty D, Gao J, Phillips SM, et al. OncoKB: a precision oncology knowledge base. J Cin Oncol Precis Oncol. 2017.
- Tenaerts P, Madre L, Archdeacon P, et al. The Clinical Trials Transformation Initiative: innovation through collaboration. Nat Rev Drug Discov. 2014;13:797–798.
- von Elm E, Altman DG, Egger M, et al.; STROBE Initiative. The Strengthening the Reporting of Observational Studies in Epidemiology (STROBE) statement: guidelines for reporting observational studies. J Clin Epidemiol. 2008;61:344–349.
- Margulies M, Egholm M, Altman WE, et al. Genome sequencing in microfabricated high-density picolitre reactors. Nature. 2005;437:376–380.
- van Dijk EL, Auger H, Jaszczyszyn Y, et al. Ten years of next-generation sequencing technology. Trends Genet. 2014;30:418–426.
- McKenzie AJ, Dilks H, Jones SF, et al. Should next-generation sequencing tests be performed on all cancer patients? Expert Rev Mol Diagn. 2019;19:89–93.
- Al-Kateb H, Nguyen TT, Steger-May K, et al. Identification of major factors associated with failed clinical molecular oncology testing performed by next generation sequencing (NGS). Mol Oncol. 2015;9:1737–1743.
- Zehir A, Benayed R, Shah RH, et al. Mutational landscape of metastatic cancer revealed from prospective clinical sequencing of 10,000 patients. Nat Med. 2017;23:703–713.
- Stockley TL, Oza AM, Berman HK, et al. Molecular profiling of advanced solid tumors and patient outcomes with genotype-matched clinical trials: the Princess Margaret IMPACT/COMPACT trial. Genome Med. 2016;8:109.
- Moorcraft SY, Gonzalez de Castro D, Cunningham D, et al. Investigating the feasibility of tumour molecular profiling in gastrointestinal malignancies in routine clinical practice. Ann Oncol. 2018;29:230–236.
- Massard C, Michiels S, Ferté C, et al. High-throughput genomics and clinical outcome in hard-to-treat advanced cancers: results of the MOSCATO 01 trial. Cancer Discov. 2017;7:586–595.
- Le Tourneau C, Delord JP, Gonçalves A, et al.; SHIVA investigators. Molecularly targeted therapy based on tumour molecular profiling versus conventional therapy for advanced cancer (SHIVA): a multicentre, open-label, proof-of-concept, randomised, controlled phase 2 trial. Lancet Oncol. 2015;16:1324–1334.
- Tran B, Brown AM, Bedard PL, et al. Feasibility of real time next generation sequencing of cancer genes linked to drug response: results from a clinical trial. Int J Cancer. 2013;132:1547–1555.
- Moore DA, Balbi K, Arkenau HT, et al. Routine use of a modest next generation sequencing panel provides additional clinically useful data beyond single gene testing in non-small cell lung cancer and is fit for purpose as a clinical assay: collated data from a single molecular diagnostic laboratory. J Cin Oncol. 2018;36:8540–8540.
- Goswami RS, Luthra R, Singh RR, et al. Identification of factors affecting the success of next-generation sequencing testing in solid tumors. Am J Clin Pathol. 2016;145:222–237.
- Morris SM, Subramanian J, Gel ES, et al. Performance of next-generation sequencing on small tumor specimens and/or low tumor content samples using a commercially available platform. PLoS One. 2018;13:e0196556.
- Tate JG, Bamford S, Jubb HC, et al. COSMIC: the catalogue of somatic mutations in cancer. Nucleic Acids Res. 2019;47:D941–D947.
- Ree AH, Russnes HG, Heinrich D, et al. Implementing precision cancer medicine in the public health services of Norway: the diagnostic infrastructure and a cost estimate. ESMO Open. 2017;2:e000158.
- Ree AH, Nygaard V, Boye K, et al. Molecularly matched therapy in the context of sensitivity, resistance, and safety; patient outcomes in end-stage cancer – the MetAction study. Acta Oncol. 2020;59:733–740.