Abstract
Aim
To evaluate differences between MR-guided daily-adaptive RT (MRgRT) and image-guided RT (IGRT) with or without fiducial markers in prostate cancer (PCa) stereotactic body radiotherapy (SBRT) in terms of dose distribution on critical structures.
Material and methods
Two hundred treatment sessions in 40 patients affected by low and intermediate PCa were evaluated. The prescribed dose was 35 Gy in 5 fractions delivered on alternate days. MRgRT patients (10) were daily recontoured, re-planned, and treated with IMRT technique. IGRT patients without (20) and with (10) fiducials were matched on soft tissues or fiducials and treated with VMAT technique. Respective CBCTs were retrospectively delineated and the prescribed plan was overlaid for dosimetric analysis. The daily dose for rectum, bladder, and prostate was registered.
Results
MRgRT resulted in a significantly lower rate of constraints violation as compared to IGRT without fiducials, especially for rectum V28Gy, rectum V32Gy, rectum V35Gy, rectum Dmax, and bladder Dmax. IGRT with fiducials reported high accuracy levels, comparable to MRgRT. MRgRT and IGRT with fiducials reported no significant prostate CTV underdosage, while IGRT without fiducials was associated with occasional cases of prostate CTV under dosage.
Conclusion
MR-guided daily-adaptive SBRT seems a feasible and accurate strategy for treating prostate cancer with ablative doses. IGRT with the use of fiducials provides a comparable level of accuracy and acceptable real-dose distribution over treatment fractions. Future study will provide additional data regarding the tolerability and the clinical outcome of this new technological approach.
Introduction
In recent years, the concept of extreme hypofractionation, also known as stereotactic body radiotherapy (SBRT), has emerged as a potential alternative to hypofractionation in selected low and intermediate-risk patients [Citation1]. As compared to standard fractionation, the recent HYPO-RT PC trial reported similar disease control with SBRT, despite reporting a mild increase in genitourinary toxicity [Citation2]. Other recent experiences reported similar outcomes and comparable toxicities between SBRT and hypofractionation [Citation3,Citation4]. Modern radiotherapy, with IGRT, especially using cone beam (CBCT), allows a safe dose escalation to the tumor while minimizing radiation to the surrounding organs at risk. Linac-based SBRT may also benefit from the flattening filter free (FFF) modality, which allows a high dose rate and the delivery of the entire radiation dose in few minutes, significantly reducing the intra-fraction uncertainties [Citation5,Citation6].
The actual concept of RT planning and delivery consists of computing a single treatment plan, whose dosimetric parameters are reported in the dose-volume histogram (DVH), which is generally used for the entire treatment course. During the treatment, the patient lays on the treatment couch, which is moved to match the daily prostate position to the original in which the treatment plan was computed. This procedure is not without uncertainties, given the fact that anatomy may significantly change over the days (i.e., rectum and bladder filling) and the CBCT image quality is generally lower compared to the diagnostic CT-scan. Therefore, this treatment procedure does not account for subsequent anatomical variations. A possible solution to reduce prostate positioning uncertainties might be represented by the implant of radio-opaque fiducial markers into the prostate, whose position is coherent with the prostate [Citation7,Citation8]. Despite being invasive, this procedure can represent a solution for prostate positioning, even if the problem of daily anatomical variation of rectum and bladder still persists. Recent research reported a low consistency between different treatment fractions and the prescribed plan in PCa SBRT, leading to significant variation in the real dose delivered to surrounding organs at risk [Citation9].
More recently, a new concept of MR-guided radiotherapy has been introduced, combining the high MR image quality to the possibility to easily re-adapt treatment plans to the daily anatomy [Citation10]. This approach is characterized by longer treatment sessions, due to the procedures required for the preparation and the re-planning workflow [Citation11]. Whether MR-guided RT might improve dose delivery accuracy as compared to standard linac treatments in PCa SBRT has been not deeply explored. The aim of the present study is to determine whether MR-guided daily adaptive RT might improve DVH consistency, as compared to standard linac-based SBRT, with or without fiducial markers, in PCa patients.
Material and methods
This is a retrospective analysis of patients treated with 1.5T MR-guided RT (MRgRT) and linac-based RT. Between 2014 and 2020, 124 patients affected by low-to-intermediate PCa were treated with linac-based SBRT with or without fiducials, or MRgRT at our Institution. Inclusion criteria of the present study were: (1) diagnosis of low-to-intermediate risk PCa treated with SBRT; (2) total prescribed dose of 35 Gy in 5 fractions; (3) prostate volume <80 ccs; (4) absence of hip prosthesis; (5) completion of the entire RT course; (6) availability of the entire CBCT or MR image set. After exclusion, a total of 10 patients with MRgRT, 15 patients without fiducials, and 10 patients with fiducial were included in the analysis. Patients treated with MRgRT were enrolled in an ongoing Ethical Committee (EC) approved trial (no. 23748), while patients treated with linac-based SBRT with fiducial markers were enrolled in an EC approved PCa SBRT phase II trial (no. SBRT PROG112CESC) [Citation12].
1.5 T MR-guided radiotherapy
Simulation and planning MR-guided RT procedures were described extensively in a previous publication [Citation11]. Before simulation (planning CT and MRI) and each fraction, patients were instructed to have their bladder half full (500 cc of water 15–20 min before the session) and empty rectum. A CT simulation scan (slice thickness 3-mm) for dose calculation purposes was acquired, followed by a high-resolution MR scan acquired by Elekta Unity. A T2-weighted MR scan was acquired during the simulation and before each fraction. The clinical target volume (CTV) was the prostate gland and the first third of the seminal vesicles (SV). The planning target volume (PTV) consisted of CTV + 5 mm margins in each direction, except 3 mm posteriorly. As organs at risk, the rectum, bladder, penile bulb, urethra, and femoral heads were delineated. The SBRT schedule consisted of five fractions of 7 Gy (total prescription dose, Dp, equal to 35 Gy) for all patients delivered on five consecutive or alternate day, corresponding to normalized total doses of 2 Gy per fraction (NTD2) between 70 and 85 Gy for an α/β estimated between 3 and 1.5 Gy for PC. The dose distribution was normalized to assure that at least 95% of the PTV received at least 95% of Dp (33.2 Gy), while less than 2% of the PTV received 107% of Dp (37.5 Gy). By considering that less than 1 cc of the PTV overlapping the rectum, bladder, and urethral planning-risk-volume (i.e., 3 mm isotropic expansion from the urethra) had to receive Dp, no less than 95% Dp (33.2 Gy) had to be assured to 95% of the PTVminus-any-overlap with the rectum, bladder, or urethral PRV. However, 98% of any of such three overlapping volumes needed to receive at least 32 Gy [Citation13]. Baseline treatment plans were generated using static field intensity-modulated radiotherapy (IMRT) delivered with 16 beams. Constraints for planning approval were the following: (1) for the rectum: V18Gy ≤35%, V28Gy ≤10%, V32Gy ≤5%, Dmax ≤35Gy; (2) for the bladder: Dmax ≤35Gy; (3) for the urethral PRV Dmax ≤35Gy. Dmax was always referred to the hottest 1 cc of the conceived organ at risk. The two treatment plan ‘adaptive’ strategies available for Elekta Unity are ‘adapt-to-position’ (ATP) and ‘adapt-to-shape’ (ATS). For ATP, the shape and weight of beam segments in the reference plan are adjusted to match the current position of target and organs-at-risk (OAR) based on rigid registration. In the case of ATS, the daily MRI is re-contoured to adapt the treatment plan of the day [Citation14]. In the PCa SBRT patients group presented here, ATS was performed for all patients in every session [Citation14]. In detail, before each fraction, a new T2-weighted MRI sequence (pre-MR) was performed and rigidly registered to the simulation MR. Through deformable registration, the original set of contours was projected onto the daily pre-MR and hence edited, as necessary, by the physician. A full reoptimization, such as starting from fluence, was performed by the physicist and, within the second optimization phase (i.e., the segmentation phase), a second verification MRI scan was acquired to test whether any shift in target position or prominent changes in target and OAR shape during planning time occurred. If not, the patient was prepared again (by enema and/or drinking), and only after this repositioned for treatment. If yes, the treatment was delivered with patient monitoring by cine MRI, typically acquired on two coronal and sagittal planes. The average treatment duration was 45 min. At the end of the delivery, a further post-MRI scan was performed, to estimate the intrafraction organ motion.
Linac-based radiotherapy
Treatment characteristics were detailed in previous papers [Citation3,Citation12] and are summarized hereafter. All patients were simulated in the supine position using Combifix™ (CIVCO, Orange City, IA, USA). A non-contrast enhanced CT scan was acquired in the treatment position (slice thickness 1 mm for patients with fiducials, and 3 mm for those without fiducials). Patients were requested to follow a bladder filling protocol and to present themselves with the rectum empty at simulation (planning CT) as well as a treatment delivery. Intraprostatic fiducial markers were implanted at least 4 weeks before simulation.
Treatment was performed using TrueBeam™ Linac (Varian Medical System, Palo Alto, CA, USA). The target definition was based on MRI T2-sequence acquired in the treatment position and rigidly fused to the simulation CT to determine the position of the urethra, as well as to better define the anatomical relationships between the prostate and rectum, bladder, and penis bulb. Contours were finally checked on the planning CT. The CTV, PTV expansion strategy, dose prescription, and organs at risk constraints were explained in the previous chapter of this article.
Treatment planning and delivery were performed using a VMAT technique, performed by 2 full arcs and collimator angle set ±10°. Beam energy was equal to 10 MV in FFF mode. Couch repositioning was completed after automatic CBCT matching to the reference planning CT, followed by manual adjustments. Matching was performed on fiducials where available or on anatomical boundaries otherwise. Treatment duration ranged between 10 and 15 min.
CBCT contouring and plan evaluation
Daily imaging registration between CBCT and simulation CT were used for the contouring procedure. Original simulation CT contours were blinded. The rectum, the bladder, and the CTV were retrospectively manually contoured on the simulation CT and each of the five CBCTs by 1 observer (L.N.) to ensure consistency. A second observer (F.A.) peer-reviewed the contour. The rectum was contoured from the slice in which it connects to the anterior sigmoid to the anal verge. A verification plan on each daily co-registered CBCT was created using Eclipse Version V15.6 (Varian Medical Systems, Palo Alto, CA, USA) according to the calibration curve from CBCT HU versus electron density. Verification plans were re-calculated, setting the same Monitor Units as the reference plan, using the anisotropic analytical algorithm (AAA 15.5.07) on a calculation grid of 2.5 mm.
Data analysis
The analyzed constraints for the rectum were: V18Gy <35%, V28Gy <10%, V32Gy <5%, V35Gy <1%, Dmax35Gy <1cc. DVH parameters for bladder were: V35Gy 5%, and Dmax35Gy <1cc. The CTV coverage was considered as 98% of CTV volume covered by 95% of the prescribed dose. CTV coverage was guaranteed in every simulation plan.
The dose received to the rectum and the bladder, and the CTV was evaluated on the DVH at each individual fraction and extracted. The dose to the constraint % volume of the structure was measured, and the dose received at this volume recorded. The dose measured at each fraction was recorded and analyzed separately to precisely describe each treatment session.
Study end-point and statistics
The primary end-point of the study was the DVH variation between the original plan and treatment session among the three groups (MR-guided daily adaptive RT, CBCT with and without fiducials). Secondary end-point was the inter-patients and the interfraction and intrafraction constraints violation. CTV coverage was also assessed and the difference between groups and the interfraction and intrafraction variation were also analyzed.
Descriptive statistics were performed to report organ volume and DVH parameters as average, and standard deviation (SD). The McNemars test of proportions was used to determine differences in the proportion of specific constraints infringement at treatment in comparison to the simulation. The Kruskal–Wallis test was used to determine differences in constraints between the three groups. The Mann–Whitney test was used to determine organ and CTV volume DVH parameter differences between simulation and treatment sessions for separate groups. The Bonferroni correction was used to account for multiple testing. Statistical analysis was performed using the SPSS statistical software package version 22.0 (SPSS Inc, Chicago, IL, USA). A corrected p value ≤ .01 indicated a significant association for rectum and bladder analysis. A p value ≤ .05 indicated a significant association for CTV analysis.
Results
Patients’ characteristics
Forty patients accounting for a total of 200 treatment sessions were treated for low and intermediate PCa with SBRT. Group 1 (MR-guided daily adaptive RT) consisted of 10 patients, group 2 (CBCT without fiducials) consisted of 20 patients, and group 3 (CBCT with fiducials) consisted of 10 patients. Group 1 patients were treated between November 2019 and February 2020; group 2 was treated between 2017 and 2019; group 3 patients were treated between 2014 and 2016. The median time from pretreatment imaging (MR or CBCT) to the end of the treatment session was 48 min and 12 min, for MR and conventional linac, respectively. Patients’ characteristics are reported in .
Table 1. Patients characteristics (n = 40).
Organs at risk
There was no significant rectum volume variation between simulation and treatment imaging among the three groups. The average rectum volume at simulation was 50.36 cc, 75.47 cc, and 88.25 cc for group 1, 2, and 3, respectively. The mean rectum volume during treatment was 50.49 cc, 77.53 cc, and 97.8 cc for group 1, 2, and 3, respectively (; ). To assess the role of rectum volume variation we correlated it with DVH parameters and found a difference in rectumV32Gy (p = .001), rectumV35Gy (p = .00), and rectumDmax (p = .00). We demonstrated an effect of pretreatment imaging technique (MR, and CBCT with or without fiducials) over the following DVH parameters: rectumV32Gy (χ2(2)=16.39, p = .00), rectumV35Gy (χ2(2)=68.46, p = .00), and rectumDmax (χ2(2)=69.17, p = .00). In particular, the overall rate of rectum constraint infringement for group 1, 2, and 3 was, respectively, as follows: rectumV28Gy (0%, 38%, 0%; p = .016), rectumV32Gy (14%, 45%, 48%; p = .00), rectumV35Gy (0%, 40%, 66%; p = .00), rectumDmax (2%, 32%, 46%; p = .00). See . The rectum DVH deviation between simulation and treatment sessions was as follows: no significant difference in group 1; rectumV35Gy (p = .00) and rectumDmax (p = .00) in group 2; rectumV32Gy (p = .013), rectumV35Gy (p = .00) and rectumDmax (p = .00) in group 3.
Figure 1. Boxplot of the average rectum volume divided according to treatment group, and planning and treatment imaging.
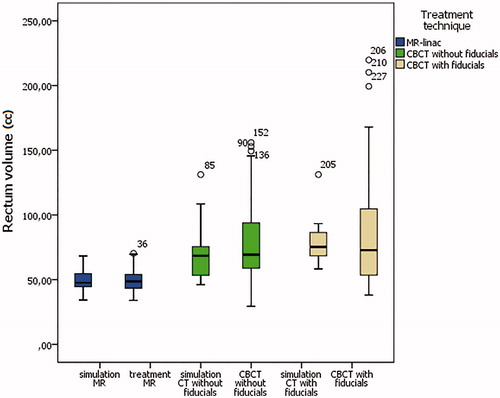
Table 2. Target and organ at risk volume.
There was no significant bladder volume variation between simulation and treatment imaging among the three groups. The average bladder volume at simulation was 261.26 cc, 275.88 ccs, and 311.73 ccs for groups 1, 2, and 3, respectively. The average bladder volume during treatment was 248.43 cc, 281.67 ccs, and 291.93 ccs for groups 1, 2, and 3, respectively (). There were no differences in the bladder constraints infringement rate according to bladder volume. We demonstrated an effect of pretreatment imaging technique (MR, and CBCT with or without fiducials) over the following DVH parameters: bladderV35Gy (χ2(2)=49.79, p = .00), bladderDmax (χ2(2)=116.38, p = .00). The overall rate of bladderDmax infringement was as follows: 0%, 50%, and 48%, for group 1, 2, and 3, respectively (p = .00) see . The bladder DVH deviation between simulation and treatment sessions was as follows: no significant difference in group 1; bladderV35Gy (p = .00) and bladderDmax (p = .00) in group 2; rectumV32Gy (p = .013), bladderV35Gy (p = .00), and bladderDmax (p = .00) in group 3.
CTV dose coverage
There was no significant prostate CTV volume variation between simulation and treatment imaging among the three groups. The average prostate CTV volume at simulation was 50.79 cc, 55.65 ccs, and 58.03 ccs for groups 1, 2, and 3, respectively. The average prostate CTV volume during treatment was 54.68 cc, 56.61 ccs, and 57.93 ccs for groups 1, 2, and 3, respectively ().
The overall rate of CTV coverage infringement during the 200 treatment sessions was as follows: 98%, 62%, and 98% for groups 1, 2, and 3, respectively (p = .00). Interestingly, the average CTV % covered by 98% of the prescribe dose during treatment was 98.09% (97.2–99%), 96.79% (69.8–100%), and 98.8% (97.63–100%), for group 1, 2, and 3, respectively (). The majority of the discrepancies were negligible but 6 treatment fractions in different patients had major deviation (<90%) in a range between 69.8% and 89.9%. These discrepancies did not correlate with biochemical failure during the follow-up. CTV volume did not correlate with CTV dose coverage. The discrepancy in the average CTV coverage between planning and treatment sessions was statistically significant in CBCT patients without fiducials (group 2; p = .024) and was not significant in MR-guided RT (group 1; p = .42) and CBCT with fiducials (group 3; p = .45).
Table 3. DVH parameters.
Discussion
The management of localized PCa significantly improved in recent years. In the localized disease, the possibility to reduce treatment sessions via hypofractionation and SBRT increased patients’ convenience with comparable oncological outcomes and safety. Nevertheless, this approach requires high personnel expertise and technological equipment [Citation1,Citation15]. Previous experiences examined the real dose delivered to OARs during prostate cancer treatment, demonstrating a significant DVH discrepancy between prescribed and administered plan with linac-based technology [Citation9,Citation16,Citation17]. The majority of these experiences analyzed conventionally or hypofractionated regimens, while very few data exist regarding SBRT. These latter focused on OARs DVH variations and organ deformations and did not analyze target dose coverage, the major oncological issue. We explored for the first time the dose delivered during daily-adaptive MR-guided SBRT compared to the linac-based approach. The results of the present analysis are in line with those previous experiences confirming the presence of major DVH discrepancies from the original treatment plan in up to 6% of linac-based treatment sessions without fiducial. The novel finding is the high constraint coherence of MR-guided treatment, were almost every session has the target covered precisely, and the OAR safely spared.
As recently reported [Citation9], linac-based prostate SBRT is characterized by a certain degree of uncertainty in some treatment fractions, which is partially counterbalanced by other fractions, so that at the end of treatment in most cases the median delivered dose and the median constraints are within the limits. Also, the linac-based approach must rely on a rigid pelvic preparation, since it cannot account for large organ variation, unless of an increase in positioning uncertainty or even target missing.
Conversely, daily-adaptive MR-guided RT allows the precise administration of the treatment due to the daily re-planning. Unfortunately, this ability is counterbalanced by longer treatment duration as compared to standard treatment (45–50 min vs. 15–20 min) and the need for MR.
We found a difference in the average rectal volume between the three groups. Our patients were all treated with bowel preparation; nevertheless, a difference in the volume was reported. This may depend on the rectum contour technique that includes the entire rectum from the rectosigmoid junction. By a retrospective review, we noticed that the part of the rectum that interfaced with the prostate was generally small, while the major variations were reported in the upper part that not received radiations. This finding strengthens our results that demonstrated no correlation between rectum volume and DVH parameters, while the treatment technique relates to DVH variation. The same consideration could be drawn for bladder filling, since their volume variation refers specifically to the upper part, outside to the treatment field. Nevertheless, we must report the different bladder filling techniques: in the linac-based approach, CBCT was acquired with the full bladder, since treatment duration is short and patients can hold urine. In the MR-guided approach, patients start with the bladder half-full, since the time from the first MR acquisition to the delivery is significantly longer and several patients cannot hold the full bladder. To ensure the correct organ positioning we perform a pre-MR, through which was evident a modification only to the upper part of the bladder [Citation11].
Interestingly, MRgRT reported the highest rectum and bladder dosimetric accuracy, as compared to SBRT without fiducials, while the difference was minimal or absent with fiducials. The major advantage was observed in the high-dose parameters. This would not surprise, since conventional radiotherapy did not account for the daily anatomical variation, therefore, despite prostate positioning is accurate, anatomical variation are not taken into account, potentially resulting in OAR overdosage. An interesting advantage of MRgRT is, therefore, the possibility to attain accuracy level comparable to those with fiducials, without invasive procedures.
The present is the first study that reported data on CTV dose coverage both with conventional linac and MR-linac. Results of the three groups showed consistent accuracy, especially for groups 1 and 3 nevertheless SBRT without fiducial might occasionally result in target underdose. In the majority of cases, these were tolerable, but with the expected increase in SBRT use after the encouraging results of a recent trial [Citation2], it is important the correct selection of patients and to acquire adequate expertise [Citation15].
MR-guided prostate SBRT with daily-adaptive planning showed high precision in our real-life experience as compared to the linac-based approach. Limitations to this technique are the actual access to this technology and the general patient limitation to MR. Even if this technique will not completely substitute conventional radiotherapy this represents a technological step forward for selected patients. According to our results, the use of fiducials can be recommended to ensure the best positioning accuracy and to reduce the risk of target under dosage.
Limitations of the study are the retrospective nature, the small sample size, the absence of a post-treatment CBCT in groups 2 and 3. Moreover, DVH analysis might have benefited from a spatial analysis, as reported elsewhere [Citation18]. This subject is still debated, since some authors reported significant modification from the pretreatment CBCT, while others reported negligible differences [Citation19–21]. The use of VMAT FFF technique minimizing delivery duration might have reduced this bias, while the treatment duration represents a not negligible lacking point in MR-guided treatment. From the patient’s point of view, in a recent experience, MR-guided treatment to the prostate demonstrated to be well tolerated with no impact on the quality of life [Citation11]. This study is of interest since MR procedures require patients’ compliance, and therefore, proposing them a treatment with a demonstrated and reproducible accuracy, in some cases comparable or higher than conventional one, might motivate and help them in orienting their decision toward a technique over another, also taking into account the objectively longer duration of MR-guided approach. Since MR-linacs have the evident actual limit of long treatment duration, shorter schedules are preferred to increase patient adherence to the treatment and comfort. Nevertheless, we are facing a change of perspective in radiation oncology, with the introduction of new technology that allows delivering precise and controlled treatments. In the next years, the ongoing clinical trials on the single fraction prostate SBRT might change the landscape in the treatment of localized PCa, and early results seem encouraging [Citation22]. If these studies will confirm the oncological equivalence to the actual hypofractionated and stereotactic schedules, MR-linac would be an interesting candidate tool to deliver this kind of surgical-like treatment.
Conclusion
The present is the first study comparing treatment delivered dose between the initial plan and treatment sessions with conventional linac and daily-adaptive strategy with MR-linac. Results showed consistent accuracy for MR-linac and the group with intraprostatic fiducials. SBRT without fiducials resulted in occasional target underdose or constraints violation, despite the average treatment accuracy was assured. MR-guided daily-adaptive radiotherapy seems a promising approach for minimizing treatment uncertainties and to improve treatment outcome.
Disclosure statement
Prof. Filippo Alongi is consultant for Elekta and received honoraria as speaker. Ruggero Ruggieri is consultant for Elekta.
References
- nccn.org [Internet]. Plymouth Meeting, PA: National Comprehensive Cancer Network [cited 2020 May 6]. Available from: https://www.nccn.org/patients/guidelines/content/PDF/prostate-patient.pdf
- Widmark A, Gunnlaugsson A, Beckman L, et al. Ultra-hypofractionated versus conventionally fractionated radiotherapy for prostate cancer: 5-year outcomes of the HYPO-RT-PC randomised, non-inferiority, phase 3 trial. Lancet. 2019;394(10196):385–395.
- Nicosia L, Mazzola R, Rigo M, et al. Moderate versus extreme hypofractionated radiotherapy: a toxicity comparative analysis in low- and favorable intermediate-risk prostate cancer patients. J Cancer Res Clin Oncol. 2019;145(10):2547–2554.
- Dearnaley D, Syndikus I, Mossop H, et al. Conventional versus hypofractionated high-dose intensity-modulated radiotherapy for prostate cancer: 5-year outcomes of the randomised, non-inferiority, phase 3 CHHiP trial. Lancet Oncol. 2016;17(8):1047–1060.
- Alongi F, Cozzi L, Arcangeli S, et al. Linac based SBRT for prostate cancer in 5 fractions with VMAT and flattening filter free beams: preliminary report of a phase II study. Radiat Oncol. 2013;8:171.
- Duffton A, Sadozye A, Devlin L, et al. Safety and feasibility of prostate stereotactic ablative radiotherapy using multimodality imaging and flattening filter free. Br J Radiol. 2018;91(1084):20170625.
- de Muinck Keizer DM, Pathmanathan AU, Andreychenko A, et al. Fiducial marker based intra-fraction motion assessment on cine-MR for MR-linac treatment of prostate cancer. Phys Med Biol. 2019;64(7):07NT02.
- O'Neill AG, Jain S, Hounsell AR, et al. Fiducial marker guided prostate radiotherapy: a review. Br J Radiol. 2016;89(1068):20160296.
- Devlin L, Dodds D, Sadozye A, et al. Dosimetric impact of organ at risk daily variation during prostate stereotactic ablative radiotherapy. Br J Radiol. 2020;93(1108):20190789.
- Corradini S, Alongi F, Andratschke N, et al. MR-guidance in clinical reality: current treatment challenges and future perspectives. Radiat Oncol. 2019;14(1):92.
- Alongi F, Rigo M, Figlia V, et al. 1.5T MR-guided and daily adapted SBRT for prostate cancer: feasibility, preliminary clinical tolerability, quality of life and patient-reported outcomes during treatment. Radiat Oncol. 2020;15(1):69.
- Alongi F, Mazzola R, Fiorentino A, et al. Phase II study of accelerated Linac-based SBRT in five consecutive fractions for localized prostate cancer . Strahlenther Onkol. 2019;195(2):113–120.
- Ruggieri R, Naccarato S, Stavrev P, et al. Volumetric-modulated arc stereotactic body radiotherapy for prostate cancer: dosimetric impact of an increased near-maximum target dose and of a rectal spacer. Br J Radiol. 2015;88(1054):20140736.
- Winkel D, Bol GH, Kroon PS, et al. Adaptive radiotherapy: the Elekta Unity MR-linac concept. Clin Transl Radiat Oncol. 2019;18:54–59.
- Guckenberger M, Baus WW, Blanck O, et al. Definition and quality requirements for stereotactic radiotherapy: consensus statement from the DEGRO/DGMP working group stereotactic radiotherapy and radiosurgery. Strahlenther Onkol. 2020;196(5):417–420.
- Wahl M, Descovich M, Shugard E, et al. Interfraction anatomical variability can lead to significantly increased rectal dose for patients undergoing stereotactic body radiotherapy for prostate cancer. Technol Cancer Res Treat. 2017;16(2):178–187.
- Pearson D, Gill SK, Campbell N, et al. Dosimetric and volumetric changes in the rectum and bladder in patients receiving CBCT-guided prostate IMRT: analysis based on daily CBCT dose calculation. J Appl Clin Med Phys. 2016;17(6):107–117.
- Cheng CW, Das IJ. Treatment plan evaluation using dose-volume histogram (DVH) and spatial dose-volume histogram (zDVH). Int J Radiat Oncol Biol Phys. 1999;43(5):1143–1150.
- Hatton JA, Greer PB, Tang C, et al. Does the planning dose-volume histogram represent treatment doses in image-guided prostate radiation therapy? Assessment with cone-beam computerised tomography scans. Radiother Oncol. 2011;98(2):162–168.
- Varadhan R, Hui SK, Way S, et al. Assessing prostate, bladder and rectal doses during image guided radiation therapy-need for plan adaptation? J Appl Clin Med Phys. 2009;10(3):2883.
- Kupelian PA, Langen KM, Zeidan OA, et al. Daily variations in delivered doses in patients treated with radiotherapy for localized prostate cancer. Int J Radiat Oncol Biol Phys. 2006;66(3):876–882.
- Zilli T, Franzese C, Bottero M, et al. Single fraction urethra-sparing prostate cancer SBRT: phase I results of the ONE SHOT trial. Radiother Oncol. 2019;139:83–86.