Abstract
Background
Proton therapy (PT) is sensitive towards anatomical changes that may occur during a treatment course. The aim of this study was to investigate if anatomically robust PT (ARPT) plans incorporating patient-specific target motion improved target coverage while still sparing normal tissues, when applied on locally advanced prostate cancer patients where pelvic irradiation is indicated.
Material and methods
A planning computed tomography (CT) scan used for dose calculation and two additional CTs (acquired on different days) were used to make patient-specific targets for the ARPT plans on the eight included patients. The plans were compared to a conventional robust PT plan and a volumetric modulated arc therapy (VMAT) photon plan, which were derived from the planning CT (pCT). Worst-case robust optimisation was used for all proton plans with a setup uncertainty of 5 mm and a range uncertainty of 3.5%. Target coverage (V95% and D95%) and normal tissue doses (V5–75 Gy) were evaluated on 6–8 rCTs per patient.
Results
The ARPT plans improved the prostate target coverage for the most challenging patient compared to conventional robust PT plans (20% point increase for V95% and 31 Gy increase for D95%). Across the whole cohort the estimated mean value for V95% was 97% for the ARPT plans and 95% for the conventional robust PT plans. The ARPT plans had a slight, statistically insignificant increase in normal tissue doses compared to the conventional robust proton plans. Compared to VMAT, the ARPT plans significantly reduced the normal tissue doses in the low-to-intermediate dose range.
Conclusions
While both proton plans reduced the low-to-intermediate normal tissue doses compared to VMAT, ARPT plans improved the target coverage for the most challenging patient without significantly increasing the normal tissue doses compared to conventional robust PT plans.
Introduction
The favourable depth dose distribution of proton therapy (PT) compared to photon-based radiotherapy (RT) reduces the low-to-intermediate doses delivered to organs at risk (OARs) and normal tissues, potentially reducing the risk of treatment-induced morbidities [Citation1–3]. However, this makes PT more sensitive towards anatomical changes in the patient, as well as to range and patient set-up uncertainties. Such variations can cause dose degradations during the course of treatment, reducing the chance of tumour control and increasing the risk of normal tissue morbidity [Citation4,Citation5]. Robust optimisation has been introduced to make plans less sensitive by mitigating range and set-up uncertainties compared to plans optimised with a conventional planning target volume (PTV) approach [Citation6,Citation7]. However, these robust optimisation strategies often use standardised cohort-based uncertainties and are not per se developed to take the often complex internal organ motion patterns into account.
To account for daily anatomical variations, on-line daily plan adaptation can be employed, e.g., dose-guidance, but it is time- and resource-consuming [Citation8–12]. On-line adaptation needs to be performed in a short time-span before further severe changes to the anatomy occur. To reduce the need for adaptation, anatomical motion patterns can be included in the optimisation process. Wang et al. [Citation13] explored the use of two CTs in the optimisation of intensity-modulated proton therapy (IMPT) on lung cancer patients, with the second CT taken halfway through the treatment. In the optimisation process, both anatomical information and structures were incorporated followed by a re-optimisation of the original dose distribution. They found this method to improve plan robustness towards anatomical changes. van de Water et al. [Citation14] more explicitly explored anatomical robust PT (ARPT) planning to account for variations in nasal cavity filling on patients with tumours in the sino-nasal region. In the optimisation process, anatomical changes were accounted for by including synthetic CTs with varying nasal cavity densities as anatomical variation scenarios. They concluded that anatomical robust optimisation could effectively account for variations in nasal cavity filling during PT. Cubillos-Mesias et al. [Citation15] recently investigated anatomical robust optimisation using three CTs in the optimisation process for head and neck patients using IMPT. They found that including random anatomical variations in the optimisation improved plan robustness.
The internal organ motion patterns in the pelvis are complex, with variations in organ filling and position of key OARs, including the bowels, the bladder, and the rectum [Citation16–18]. Inter-fractional organ motion can cause target dose degradations which are difficult to account for either by increasing margins or changing the patient set-up approach [Citation4]. We propose an ARPT planning strategy where patient-specific organ motion patterns are incorporated to account for pelvic organ motion. The strategy was explored for use in PT planning of patients with locally advanced prostate cancer, to increase plan robustness (i.e., improve target coverage) while still sparing normal tissues.
Material and methods
Anatomical vs. conventional robust proton therapy planning
The ARPT plans were created using the target contours for both the high dose and the elective targets in three ‘planning’ CTs. The two latter CTs were rigidly registered based on bony anatomy to the first CT. The clinical target volumes (CTVs) were delineated in all three CTs, and then transferred from the consecutive CTs to the first CT and their union created new patient-specific target volumes for use in the first CT. Subsequently, the overlap between the patient-specific targets and the normal tissues were removed from the new targets (Supplementary Material A). The new high dose patient-specific targets increased in volume size with 11–49% compared to the original CTVs in the first CT. The three CTs were acquired on different days to capture inter-fractional organ motion.
For comparison, conventional robust PT plans were made using the original targets from the first CT, while the ARPT plans were optimised using the patient-specific targets. All proton plans used spot scanning PT delivery, and were made using worst-case robust optimisation with Eclipse treatment planning system (TPS) (Varian Medical systems, Palo Alto, CA, USA) and a setup uncertainty of ±5 mm along the three cardinal axes and our local range uncertainty setting of ±3.5% resulting in 12 scenarios, in addition to two scenarios with a setup uncertainty of zero and a range uncertainty of ±3.5%. A five-field beam arrangement was used for both the ARPT and conventionally robust PT plans, with two lateral opposing fields at 90 and 270 degrees targeting the prostate and seminal vesicle targets, while three posterior oblique fields at 150, 180, and 210 degrees were targeting the lymph node target. The plans were approved by a senior clinical medical physicist. Both proton plans were calculated and optimised on the planning CT (pCT) geometry, hence the additional CTs were not used in the optimisation process but only for target generation.
Patient cohort and dose prescriptions
Eight patients with locally advanced prostate cancer originally treated at Haukeland University Hospital, Norway with intensity-modulated RT were included in the study. The patients were originally included in a clinical study approved by the relevant local ethics committee [Citation16]. A pCT and between 8 and 10 repeated CTs (rCTs) (with 2–3 mm slice thickness) had been acquired during the treatment course (approximately two scans per week). No bladder or rectum protocol was used; however, seven of the eight patients had contrast in their bladder when the pCT scan was acquired. This contrast was overwritten to the mean Hounsfield unit from the first rCT. Two experienced radiation oncologists contoured the targets and OARs using Eclipse TPS. The bowel cavity, bowel loops, and the lymph node/seminal vesicle CTV (ln/sv CTV), which included the presacral lymph nodes, were contoured following the Radiation Therapy Oncology Group (RTOG) contouring guidelines. Detailed information on how the prostate CTV, bladder, and rectum were contoured can be found in Thörnqvist et al. [Citation19].
A dose of 78 Gy (RBE) was prescribed to the prostate while 56 Gy (RBE) was prescribed to the ln/sv target in 39 fractions, with the in-house photon-based clinical protocol for the OARs used when approving the plans (Supplementary Material B). However, D95% ≥ 98% were used for both the nominal CTVs and the 14 uncertainty scenarios: the ±5 mm setup/±3.5% range uncertainty and the 0 mm/±3.5% scenarios.
Reference photon-based treatment plan
Photon-based treatment plans were made with volumetric arc therapy (VMAT) using three 15 MV arcs: the first and third arc in a clockwise rotation from 181 to 179 degrees and the second arc in a counter-clockwise rotation from 179 to 181 degrees. For the third arc, the collimator was rotated 95 degrees as compared to the other arcs.
For the VMAT plans, the prostate CTV was expanded 7 mm in all directions, except in the superior–inferior (SI) direction where it was expanded 9 mm to create the corresponding PTV, while the ln/sv CTV was expanded 5 mm, except in the SI direction, where 8 mm was used. The VMAT plans and PTVs were re-created according to our clinical protocol.
Data analysis
All three plans for the eight cases were re-calculated on 6–8 rCTs after a bony anatomy-based rigid registration, not including the two CTs used in the ARPT plans. Doses to the target volumes were evaluated using V95% and D95% across the rCTs. For the OARs, the relative volume fraction receiving doses above 5, 10, and up to 75 Gy (in 5 Gy intervals, i.e., V5–75 Gy) were evaluated across the rCTs, along with the mean dose. In addition, normal tissue complication probabilities (NTCPs) for the rectum and bladder and the bowel cavity were investigated (Supplementary Material C) [Citation20–24]. The endpoints for the rectum NTCP models were late rectal bleeding ≥ 2 and the endpoints for the two bladder NTCP models were bladder urgency and grade 3+ late urinary morbidity. For the bowel, the risk of grade 2+ acute diarrhoea was investigated.
To statistically compare the three plans, a linear mixed-effect model with a fixed effect of modalities and random effects of patients and repeated scans per patient was used to estimate the mean values and 95% confidence interval (CI) for the different dose-volume metrics. The model took into account that the same patient group was used in each plan and that each patient had a varying number of rCTs. Normality was evaluated based on a quantile–quantile plot of the residuals.
Results
The two proton plans were overall similar with respect to coverage of the targets and doses to the OARs. However, the ARPT plans improved the dose to the prostate CTV compared to the conventional robust PT plans for in particular one patient (patient 3) where the conventional robust PT plan did not secure target coverage. For this case, there was an increase of 20% points for V95% and 30.9 Gy for D95% ( and ). Across the whole cohort, the estimated mean values for V95% were 97% (95% CI: 89–105%) for the ARPT plans, 95% (95% CI: 87–103%) for the conventional robust PT plans, and 95% (95% CI: 87–103%) for the VMAT plans. The estimated mean values for D95% were 72.3 Gy (95% CI: 67.2–77.3 Gy) for the ARPT plans, 75.3 Gy (95% CI: 70.2–80.3 Gy) for the conventional robust PT plans, and 74.6 Gy (95% CI: 69.5–79.6 Gy) for the VMAT plans (). The ARPT plans did not significantly improve the ln/sv CTV target dose. The estimated mean values for V95% were 99% and D95% were around 55 Gy for both proton plans as well as with VMAT.
Figure 1. DVH-curves for the planned dose and the dose in one of the rCTs for patient three for the targets and OARs with the conventional robust PT plan (solid line) and the ARPT plan (dotted line).
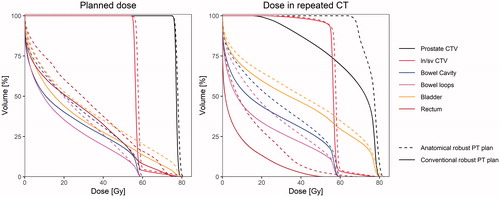
Figure 2. The V95% and D95% values in the first CT (red cross) and median and range (black dot and line) across the rCTs for the prostate CTV and ln/sv CTV across all patients and plans. The prescribed dose is 78 Gy for the prostate CTV and 56 Gy for the ln/sv CTV (horizontal line in C and D).
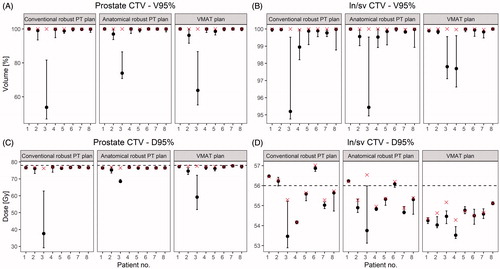
The ARPT plans slightly increased the doses to the OARs compared to the conventional robust PT plans ( and ). However, when investigating V5–75 Gy, significant differences were not found between the two proton plans (). Both proton plans had significantly lower OAR volumes receiving low-to-intermediate doses than the VMAT plans. For V25 Gy to the rectum, a mean value of 35% (95% CI: 28–43%) in the ARPT plans and a mean value of 30% (95% CI: 22–37%) in the conventional robust PT plans were observed, while the mean value was 61% (95% CI: 53–69%) for VMAT. Similar patterns were seen for the bladder, bowel cavity, and bowel loops (), as well as for the mean dose (Supplementary Material D).
Figure 3. The planned dose distributions (upper row) and the dose distributions from one of the rCTs (bottom row) for a conventional robust PT plan, ARPT plan, and VMAT plan. All images are from patient three.
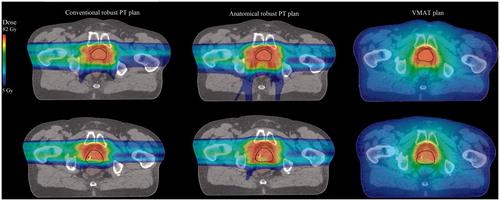
Figure 4. V5–V75 Gy for the rectum and bladder and V5–V60 Gy for the bowel loops and bowel cavity across all patients and plans.
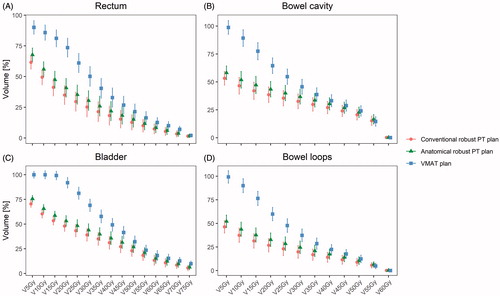
Figure 5. The V25 Gy value in the first CT (red cross) and median and range across all rCTs (black dot and line) for the OARs across all patients and plans.
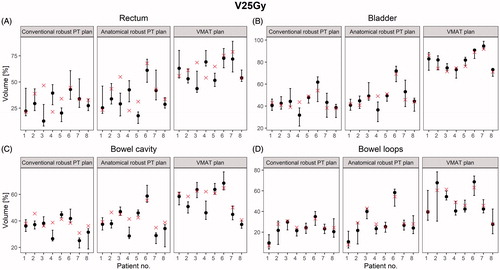
For the NTCP models explored, there was no statistically significant difference between the three plans for late rectal bleeding, grade 3+ late urinary morbidity, or grade 2+ acute diarrhoea (Supplementary Materials E and F). For the NTCP models estimating late rectal bleeding, the highest estimated mean occurred for the VMAT plans with a value of 4% (95% CI: 3–6%) for the model by Quantec. For grade 3+ late urinary morbidity the estimated mean values were between 3 and 4% across all three plans, while the estimated mean values for grade 2+ acute diarrhoea were 30–33% across all three plans. However, for the NTCP model predicting bladder urgency the estimated mean values were 15% (95% CI: 12–17%) for the ARPT plans, 12% (95% CI: 10–15%) for the conventional robust PT plans and 25% (95% CI: 22–28%) for the VMAT plans.
Discussion
In this study, we have investigated the use of patient-specific target volumes estimated from multiple pCTs to secure target coverage throughout a course of PT for locally advanced prostate cancer patients. The main finding was that including patient-specific targets in the planning process could improve the target coverage for challenging patients without significantly increasing the normal tissue doses. However, the conventional robust PT plans were already robust, at least for the relatively modest organ motion patterns seen in most of our patients.
This study was performed within the context of the planning of a Danish national randomised clinical trial for locally advanced prostate cancer that will investigate if IMPT reduces normal tissue toxicity compared to conventional photon-based RT. A major challenge of PT for pelvic tumour sites is to secure target coverage throughout the course of treatment under the influence of organ motion, including independent motion of the involved target volumes [Citation4]. To address this challenge, we have in this study investigated patient-specific targets with the use of ARPT plans. For the most challenging patient, the ARPT plan improved dose coverage to the prostate CTV compared to a conventional robust PT plan. In the first CT scan (the one used for the dose calculations) this patient had air and faeces in the rectum, which were not present at the subsequent CTs. In clinical practice, such a patient would be candidate for adaptive re-planning although the challenges with random variations would still remain. It should also be mentioned that this study has been performed on a photon-based cohort and stricter criteria may be used for the pCTs in PT. Except for this patient, we did not observe any other major differences when evaluating target coverage. Other studies have also found that incorporating multiple CTs into the optimisation process have improved plan robustness, although it slightly increased the dose to the OARs [Citation13–15]. Wang et al. [Citation13] and Cubillos-Mesias et al. [Citation15] included both the images and structures into the optimisation process for lung and head and neck cancer, respectively. Including the CT data into the optimisation process or including bony variations from the consecutive CTs in ARPT plans can potentially improve the target dose further for challenging patients. However, it will also increase the optimisation time and the number of delineated structures. Synthetic CTs can also be incorporated into the optimisation process as van de Water et al. investigated [Citation14], but complex internal pelvic organ motion is difficult to estimate for new patients by varying the densities for the OARs. As an alternative a probability distribution can be modelled based on, e.g., a principal component analysis under the assumption that general movements and deformations are similar over a population [Citation25–27]. If dose deterioration can be predicted, patients with complex organ motion could avoid unnecessary irradiation to the normal tissue.
Protons are highly sensitive towards density changes and we have therefore used bony anatomy-based rigid registrations to avoid high-density changes in the beam path direction due to changes in bony position [Citation28]. The focus of this article was on organ motion, hence we have omitted the range errors stemming from the CT calibration in the rCTs; however, it is important to investigate this before clinical implementation. We furthermore used a 5 mm setup uncertainty for the proton plans, which was found to sufficiently cover the targets, while reducing the dose to the OARs compared to VMAT. We used the same setup uncertainty for both the conventional and ARPT plans to minimise the number of variables introduced in the study. However, soft-tissue-based rigid registration and various margins could be further investigated with the aim of reducing the dose to the OARs and potentially increase plan robustness.
The ARPT plans lead to an insignificantly increase in OAR doses compared to the conventional robust PT plans, caused by enlarged target volumes, which was also seen in previous similar studies [Citation13–15]. The VMAT plans were included in this study to illustrate that the increased OAR doses in the low-to-intermediate dose range in the ARPT plans were still significantly lower than what can be achieved with state-of-the-art photon-based RT. Other studies have also found PT able to reduce the dose in the low-to-intermediate range for OARs in the pelvic region compared to photon-based RT [Citation29–31]. The same trend was observed for the bladder urgency model, since the volume effect parameter, n, correlated with the mean dose. Caution should be taken when using NTCP models to predict morbidity since large difference in morbidity predictions have been found between modalities and cohorts [Citation32]. The Quantec review of the photon-based rectum NTCP model (used in this study) showed there is a connection between the low-to-intermediate doses and morbidity [Citation21]; however, for PT there is a significant reduction in the integral dose compared to photon-based RT. The grade 3+ late urinary morbidity model (used in this study) was estimated from a prostate cancer cohort treated with passive scattering PT, where it was found that anticoagulant and age were the most prominent predictors in NTCP models [Citation23]. This, in addition to difference in patient-specific factors, biological effect, target dose prescription and treatment technique, may affect the applicability of NTCP models in studies comparing modalities with such considerable underlying differences in biology and physics as photon- vs. proton-based RT [Citation33].
Before conducting this study, four different field configurations for three patients were investigated along with overwriting the air in the bowel loops before optimisation for the proton plans (Supplementary Material G). There was no clear difference in dose to the OARs between the four configurations. However, we found that a configuration with beams at 90, 180, and 270 degrees and a configuration with beams at 90, 150, 180, 210, and 270 degrees gave a better target coverage. We decided to use the latter configuration in our study, since fields from posterior targeting the lymph nodes would be more robust than lateral opposed fields, if a patient lost or gained weight throughout a treatment course. A previous study from our group also indicated that posterior PT fields targeting the pelvic lymph nodes are robust towards organ motion [Citation34]. In addition, we found no reason to overwrite the air in the bowel loops using posterior fields. Other studies have also investigated the impact of air using PT in pelvic cancer and found it to have a minor impact on target and OAR doses [Citation35,Citation36].
To clinically implement ARPT, at least two CT scans, acquired before treatment start, are needed per patient. Furthermore, the CTs must be acquired on different days or at least with several hours in between to properly capture inter-fractional organ motion. Based on the findings of this study, some form of ARPT planning should be considered in the clinical implementation of locally advanced prostate cancer patients, or at least to certain subgroups of them experiencing large inter-fractional organ motion.
In future studies, it could be interesting to explore ARPT on patients where large inter-fractional organ motion has occurred, since relatively modest organ motion patterns were seen in our admittedly small patient cohort. In addition, increased plan robustness may also be achieved by accounting for independent target motion. One method could be to make individual treatment plans per target volume, which combined would be robust towards independent isocentre corrections. This approach could potentially secure target coverage without the need for adaptation.
In conclusion, in this study, we have explored the use of patient-specific target volumes to account for inter-fractional organ motion throughout a treatment course for locally advanced prostate cancer. It was possible to improve target coverage for one challenging patient, while the others had a similar target coverage. The potentially improved robustness to motion came without significant increased doses to any of the OARs.
Supplemental Material
Download MS Word (1.5 MB)Acknowledgments
The authors thank Bo Martin Bibby for his help in the statistical analysis.
Disclosure statement
No potential conflict of interest was reported by the author(s).
Additional information
Funding
References
- Chuong MD, Hartsell W, Larson G, et al. Minimal toxicity after proton beam therapy for prostate and pelvic nodal irradiation: results from the proton collaborative group REG001-09 trial minimal toxicity after proton beam therapy for prostate and pelvic nodal irradiation: results from the proton. Acta Oncol. 2018;57(3):368–374.
- Zhang W, Zhang X, Yang P, et al. Intensity-modulated proton therapy and osteoradionecrosis in oropharyngeal cancer. Radiother Oncol. 2017;123(3):401–405.
- Scorsetti M, Cozzi L, Navarria P, et al. Intensity modulated proton therapy compared to volumetric modulated arc therapy in the irradiation of young female patients with Hodgkin’s lymphoma. Assessment of risk of toxicity and secondary cancer induction. Radiat Oncol. 2020;15(1):12.
- Thörnqvist S, Muren LP, Bentzen L, et al. Degradation of target coverage due to inter-fraction motion during intensity-modulated proton therapy of prostate and elective targets. Acta Oncol. 2013;52(3):521–527.
- Müller BS, Duma MN, Kampfer S, et al. Impact of interfractional changes in head and neck cancer patients on the delivered dose in intensity modulated radiotherapy with protons and photons. Phys Med. 2015;31(3):266–272.
- Liu W, Zhang X, Li Y, et al. Robust optimization of intensity modulated proton therapy. Med Phys. 2012;39(2):1079–1091.
- Stuschke M, Kaiser A, Abu Jawad J, et al. Multi-scenario based robust intensity-modulated proton therapy (IMPT) plans can account for set-up errors more effectively in terms of normal tissue sparing than planning target volume (PTV) based intensity-modulated photon plans in the head and neck regi. Radiat Oncol. 2013;8(1):145.
- Albertini F, Matter M, Nenoff L, et al. Online daily adaptive proton therapy. Br J Radiol. 2020;93(1107):20190594.
- Jagt TZ, Breedveld S, van Haveren R, et al. Plan-library supported automated replanning for online-adaptive intensity-modulated proton therapy of cervical cancer. Acta Oncol. 2019;58(10):1440–1445.
- Nenoff L, Matter M, Hedlund Lindmar J, et al. Daily adaptive proton therapy–the key to innovative planning approaches for paranasal cancer treatments. Acta Oncol. 2019;58(10):1423–1428.
- Kurz C, Süss P, Arnsmeyer C, et al. Dose-guided patient positioning in proton radiotherapy using multicriteria-optimization. Z Med Phys. 2019;29(3):216–228.
- Busch K, Muren LP, Thörnqvist S, et al. On-line dose-guidance to account for inter-fractional motion during proton therapy. Phys Imaging Radiat Oncol. 2019;9:7–13.
- Wang X, Li H, Zhu XR, et al. Multiple-CT optimization of intensity-modulated proton therapy – is it possible to eliminate adaptive planning? Radiother Oncol. 2018;128(1):167–173.
- van de Water S, Albertini F, Weber DC, et al. Anatomical robust optimization to account for nasal cavity filling variation during intensity-modulated proton therapy: a comparison with conventional and adaptive planning strategies. Phys Med Biol. 2018;63(2):025020.
- Cubillos-Mesías M, Troost EGC, Lohaus F, et al. Including anatomical variations in robust optimization for head and neck proton therapy can reduce the need of adaptation. Radiother Oncol. 2019;131:127–134.
- Hysing LB, Söhn M, Muren LP, et al. A coverage probability based method to estimate patient-specific small bowel planning volumes for use in radiotherapy. Radiother Oncol. 2011;100(3):407–411.
- Muren LP, Smaaland R, Dahl O. Organ motion, set-up variation and treatment margins in radical radiotherapy of urinary bladder cancer. Radiother Oncol. 2003;69(3):291–304.
- Hysing LB, Kvinnsland Y, Lord H, et al. Planning organ at risk volume margins for organ motion of the intestine. Radiother Oncol. 2006;80(3):349–354.
- Thörnqvist S, Bentzen L, Petersen JBB, et al. Plan robustness of simultaneous integrated boost radiotherapy of prostate and lymph nodes for different image-guidance and delivery techniques. Acta Oncol. 2011;50(6):926–934.
- Burman C, Kutcher GJ, Emami B, et al. Fitting of normal tissue tolerance data to an analytic function. Int J Radiat Oncol Biol Phys. 1991;21(1):123–135.
- Michalski JM, Gay H, Jackson A, et al. Radiation dose-volume effects in radiation-induced rectal injury. Int J Radiat Oncol Biol Phys. 2010;76(3 Suppl):S123–S129.
- Mavroidis P, Pearlstein KA, Dooley J, et al. Fitting NTCP models to bladder doses and acute urinary symptoms during post-prostatectomy radiotherapy. Radiat Oncol. 2018;13(1):8.
- Pedersen J, Liang X, Casares-Magaz O, et al. Multivariate normal tissue complication probability models for rectal and bladder morbidity in prostate cancer patients treated with proton therapy. Radiother Oncol. 2020;153:279–288.
- Søndergaard J, Holmberg M, Jakobsen AR, et al. A comparison of morbidity following conformal versus intensity-modulated radiotherapy for urinary bladder cancer. Acta Oncol. 2014;53(10):1321–1328.
- Budiarto E, Keijzer M, Storchi PR, et al. A population-based model to describe geometrical uncertainties in radiotherapy: applied to prostate cases. Phys Med Biol. 2011;56(4):1045–1061.
- Furmanova K, Muren LP, Casares-Magaz O, et al. PREVIS: predictive visual analytics of anatomical variability for radiotherapy decision support. IEEE Pacific Vis Symp. 2021.
- Thörnqvist S, Hysing LB, Zolnay AG, et al. Treatment simulations with a statistical deformable motion model to evaluate margins for multiple targets in radiotherapy for high-risk prostate cancer. Radiother Oncol. 2013;109(3):344–349.
- Trofimov A, Nguyen PL, Efstathiou JA, et al. Interfractional variations in the setup of pelvic bony anatomy and soft tissue, and their implications on the delivery of proton therapy for localized prostate cancer. Int J Radiat Oncol Biol Phys. 2011;80(3):928–937.
- Widesott L, Pierelli A, Fiorino C, et al. Helical tomotherapy vs. intensity-modulated proton therapy for whole pelvis irradiation in high-risk prostate cancer patients: dosimetric, normal tissue complication probability, and generalized equivalent uniform dose analysis. Int J Radiat Oncol Biol Phys. 2011;80(5):1589–1600.
- Rana S, Cheng C, Zheng Y, et al. Proton therapy vs. VMAT for prostate cancer: a treatment planning study. Int J Part Ther. 2014;1(1):22–33.
- Busch K, G, Andersen A, Casares-Magaz O, et al. Evaluating the influence of organ motion during photon vs. proton therapy for locally advanced prostate cancer using biological models. Acta Oncol. 2017;56(6):839–845.
- Pedersen J, Flampouri S, Bryant C, et al. Cross-modality applicability of rectal normal tissue complication probability models from photon- to proton-based radiotherapy. Radiother Oncol. 2020;142:253–260.
- Pedersen J, Petersen JBB, Stokkevåg CH, et al. Biological dose and complication probabilities for the rectum and bladder based on linear energy transfer distributions in spot scanning proton therapy of prostate cancer. Acta Oncol. 2017;56(11):1413–1419.
- Andersen AG, Casares-Magaz O, Muren LP, et al. A method for evaluation of proton plan robustness towards inter-fractional motion applied to pelvic lymph node irradiation. Acta Oncol. 2015;54(9):1643–1650.
- Berger T, Petersen JBB, Lindegaard JC, et al. Impact of bowel gas and body outline variations on total accumulated dose with intensity-modulated proton therapy in locally advanced cervical cancer patients. Acta Oncol. 2017;56(11):1472–1478.
- Yao W, Schweitzer N, Biswal N, et al. Impact of bowel and rectum air on target dose with robustly optimized intensity-modulated proton therapy plans. Acta Oncol. 2020;59(10):1186–1192.