Background
Many cancers result in metastasis in the spinal vertebrae, which can cause pain, neurological disability, and reduce quality of life [Citation1,Citation2]. To improve the local disease control and relieve symptoms, radiosurgery could be used for the primary treatment of spinal metastases [Citation3–5]. Compared to the standard radiotherapy, Stereotactic body radiosurgery/radiotherapy (SBRS/SBRT) delivers a much higher dose to the tumor in fewer treatment fractions while minimizing the unnecessary dose to the healthy tissues. Such a technique has been widely adopted in the management of localized spine metastases recently [Citation6–8]. However, due to the intrafraction motion and patient comfort during the treatment, exploiting new techniques to minimize the beam-on time is one of the major topics for the spine SBRS. As a result, Volumetric Modulated Arc Therapy (VMAT), which shortens the treatment delivery time through dynamic Mutileaf Collimators (MLC) movement and gantry rotation, has been implemented as a routine clinical practice for spine SBRS/SBRT.
In the past decades, proton beam therapy has been implemented clinically, taking advantage of its unique physical characteristics of dose deposition from the ‘Bragg peak’. The development of the Pencil Beam Scanning (PBS) technique allows the proton system to optimize the energies and numerous spots to deliver the radiation dose layer by layer and spot by spot in 3-dimensional, just like a 3D-printer [Citation9]. However, due to the prolonged proton PBS treatment delivery time, only a limited number of beam angles are normally used in the proton clinic [Citation10,Citation11]. Additionally, compared to the photon treatment, IMPT alone cannot provide high dose conformity and sharp dose fall-off, which is critical to SBRS/SBRT due to the large lateral penumbra from each individual proton spot [Citation12–14]. As a result, only passive-scattering proton beam therapy using a collimator system was occasionally used in spine SBRT proton treatment cases through five or more fractions instead of a newer technique, PBS [Citation15,Citation16]. Single fractionation SBRS proton beam therapy for spine metastasis is not possible in most cases, even with passive-scattering technique due to the needs in the matching line feathering [Citation17].
Most recently, there have been a lot of questions or debates in the radiation oncology society on whether proton beam therapy has been reaching its limitations in terms of plan quality. There is high doubt that whether SBRT or SBRS treatment using PBS is even clinical feasible considering the large lateral penumbra, treatment delivery efficiency, and uncertainties [Citation18]. To address these three major challenges: Spot-scanning proton arc therapy (SPArc) treatment and planning platform was introduced by Ding et al. in 2016 [Citation19] to improve the proton treatment delivery efficiency dosimetric plan quality, and plan robustness. This is the first comprehensive study to investigate the clinical feasibility and potential benefits of utilizing SPArc in single fractionated spine SBRS treatment.
Materials and methods
Treatment planning
Seven patients with spine metastases between T2-L5 and the target volume ranging from 20.65 to 66.84 cm3 (see Supplemental Table 1s), who were previously treated using VMAT SBRS in our institution were retrospectively evaluated. Two planning groups: SPArc and IMPT, were generated on the planning Computed Tomography (CT), which was the same as the one used in VMAT. This radiosurgery target volume without expansion was utilized as planning target volume(PTV) [Citation20]. The radiation dose prescription (RX) to PTV was 18 Gy in a single fraction. The planning goal is to ensure 95% of the PTV is covered by the prescription dose. All the planning groups: VMAT, SPArc, and IMPT used similar objective constraints for OARs under protocol RTOG-0631 and Task Group 101 [Citation13,Citation20]. In terms of planning goal, the planning constraint was set that 10 Gy to no more than 10% of the partial spinal cord volume or less than 0.35 cm3 [Citation7]. If this goal and constraint cannot be achieved, the target under-coverage was accepted to meet the planning objective [Citation21]. Dose was expressed as relative biological effectiveness (RBE) dose, with RBE = 1.1 for proton plans and RBE = 1.0 for photon plans [Citation22]. The VMAT plans used Elekta Versa(6 MeV Photon), while the SPArc and IMPT plans were generated using IBA’s ProteusOne PBS proton beam model (3.2 mm spot size @227.7 MeV). Details of the planning parameters were located in the supplemental document section A.
SPArc algorithm
The SPArc optimization algorithm integrated the iterative robustness optimization approaches to resample a multi-field IMPT plan with a coarse sampling frequency to a proton arc plan with a fine control point sampling frequency [Citation19] (For more details, please see supplemental document section B). This new technique has the potential to be delivered using the existing clinical proton system [Citation19,Citation23].
Dosimetric quality evaluation
The plan quality was evaluated based on the dose-volume histograms (DVHs) of target volume and OARs in the nominal plans. More specially, plans were compared for target coverage using R50 (the volume covered by 50% RX/the target volume) [Citation24], as well as conformity index (CI) [Citation25]. Normal tissue sparing including the cord (Dmax or D0.03 cm3 and D0.35 cm3), partial cord (D10% and Dmean), lung (D1000cm3), esophagus (D5cm3), and heart (Dmean) was evaluated by comparing the SPArc planning group to VMAT and IMPT group. The integral dose (ID(Gy · L)) to the whole patient body structure was analyzed as well [Citation26].
Treatment dose reconstruction on synthetic CT based CBCT
To accurately validate the radiation dose delivered to each patient on the day of treatment, the target position and density variation from pretreatment cone-beam CT (CBCT) were utilized. The plan CT and the corresponding CBCT were deformable registered to each other (See Supplemental document C). Then, the synthetic-CT was generated by warping the CT number in the initial planning CT to the paired CBCT for dose reconstruction [Citation27].
Estimation for radiation myelopathy among different treatment modalities
During radiosurgery treatments, spinal cord injury such as radiation myelopathy(RM) may result in pain, paresthesia, sensory deficits, paralysis, bowel/bladder incontinence, and Brown-Sequard syndrome [Citation28]. In the present study, the probability of RM specific to SBRT was generated via a logistic regression model for the Dmax volume [Citation29].
Treatment delivery efficiency calculation
The total treatment delivery times except couch rotation for both SPArc and IMPT were calculated based on a 360 gantry proton system with one revolution per minute (RPM) gantry rotation speed, 2 ms spot position switching time, Energy Layer Switching Time (ELST) with 1s and 4s simulating different energy layer selection system or beamline in the current market [Citation19]. For a VMAT plan, the total delivery time was estimated based on an Elekta Versa with machine parameters: dose rate of 600 MU/min and maximum leaf speed of 3.0 cm/sec, maximum gantry speed of 5.5 degrees/s [Citation30].
Statistical analysis
The data for SPArc was utilized as a reference, the comparison with two other treatment technologies (IMPT and VMAT) were assessed with a paired, 2-tailed non-parametric Wilcoxon signed-rank test via SPSS 21.0 software (International Business Machines, Armonk, New York) respectively, and p values less than 0.05 were considered statistically significant.
Results
Plan dosimetric quality comparison
All the treatment plans met the OARs sparing according to the RTOG 0631 protocol. In terms of target coverage, SPArc significantly improved target prescription dose coverage to 95.0% ± 0.0% (SPArc) compared to 92.9% ± 2.0% (VMAT) (p = 0.04) and 92.7%±2.0%(IMPT)(p = 0.04). The dose distribution of three treatment modalities and DVH of a representative spine SBRS case #1 was displayed in , (the average DVH over the entire group of patients for three techniques were shown in Supplemental document Figure 1s)). Besides, the study found that SPArc significantly reduced the R50 to 4.24 ± 0.70 compared to IMPT (R50 = 5.56 ± 0.72, p = 0.02) and VMAT (R50 = 4.82 ± 0.55, p = 0.02) planning groups, respectively. Taking advantage of the optimization freedom through arc(s) trajectory, SPArc can spare OARs better in the high dose region close to the target. More specifically, SPArc reduced the Dmax of the cord from 10.96 ± 2.29 Gy (RBE) (IMPT) (p < 0.05) and 11.07 ± 2.21 Gy (RBE) (VMAT) (p = 0.03) to 10.21 ± 2.16 cGy (RBE) (SPArc). D1000cm3 of lung was also reduced significantly from 1.17 ± 0.98 Gy (RBE) (VMAT) to 0.07 ± 0.11 Gy (RBE) (SPArc) (p = 0.04). ID was reduced from 15.11 ± 3.24 Gy·L (VMAT, p = 0.02) to 6.49 ± 1.90 Gy·L (SPArc) as well. More detailed dosimetric metrics comparison results were list in Supplemental document Table 3s.
Figure 1. (a) Isodose distributions for treatment plan using SPArc (the first column), IMPT (the second column), and VMAT (the third column) of patient #1. (b) The corresponding DVHs comparison for a spine SBRS patient #1.
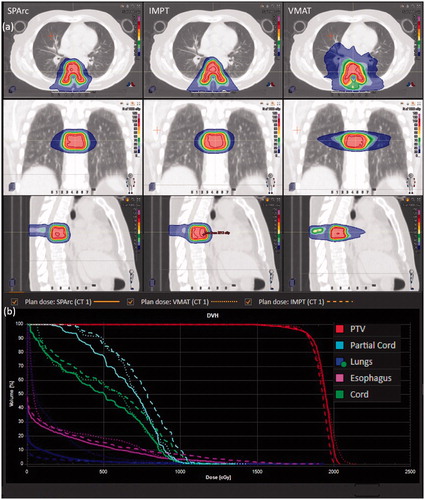
There is no statistically significant difference between SPArc and IMPT planning groups in D0.35 cm3 of cord, D10%, and Dmean of partial cord, lung D1000cm3, D5cm3 of the esophagus as well as heart Dmean. SPArc plans are comparable to VMAT plans in the following dosimetric metrics such as D0.35 cm3 of cord, D10%, and Dmean of partial cord, D5cm3 of the esophagus and Dmean of heart as well as CI.
Plan validation on synthetic CT based CBCT
The synthetic CT testing and dose reconstruction found that six out of the seven patients’ target coverage V90 was above 95.0%. The average PTV V90 coverage are 99.0 ± 0.3% (VMAT), 98.0% ± 1.8% (IMPT) and 98.0% ± 1.4% (SPArc), respectively (see an example case #1 in Supplemental document Fig 2s (a) & (b)).
The comparison of estimation for radiation myelopathy
All three technologies could meet the spinal cord constraints (RTOG 0631). The average probabilities of human SM were less than 5% among all the planning groups. However, SPArc could further reduce the probabilities of human SM from 4.3% ± 4.5% (VMAT) (p = 0.03) and 4.9% ± 4.6% (IMPT) (p = 0.03) to 2.7% ± 2.9% (SPArc) which is about 37% and 45% reduction compared to VMAT and IMPT, respectively.
Estimated total treatment delivery time
The estimated delivery time for SPArc (705.77 ± 241.24s) is less than VMAT (1088.93 ± 147.65s, p = 0.03) when ELST equal to 4 s. Once ELST reaches 1 s, SPArc treatment delivery could be comparable to a three-field IMPT (439.20 ± 87.40s (SPArc) vs 435.11 ± 89.62s (IMPT), p = 0.5), which reduced more than 50% of treatment time compared to VMAT.
Discussion
In this study, we presented a planning comparison among three approaches of delivering SBRT for spinal metastases: (a) SPArc with two partial arcs and (b) 3-field IMPT, and (c) VMAT with two or multiple partial coplanar arc trajectories. We found that SPArc plans demonstrated a superior target coverage in terms of the CI, R50, and ID compared to the IMPT planning group and improved normal tissue sparing in the lung compared to the VMAT plans.
More interestingly, the benefit of using proton therapy to reduce the integral dose is still maintained in proton arc therapy even though radiation was delivered through arc(s) trajectory. Compared to VMAT, there is still no exit dose from proton arc’s every control point. Consequently, ID was significantly reduced from 15.11 ± 3.24 Gy·L(VMAT, p = 0.02) to 6.49 ± 1.90 Gy · L(SPArc). Please note that reduction in the low dose bath depends on the patient-specific geometry and the selected arc trajectory (full vs. partial arc) or beam angles (IMPT) in the treatment plan. Lung tissue spared significantly was observed in proton plan include IMPT and SPArc compared to the photon VMAT plan. The significant advantage of dose distribution by using proton for spine SBRS may further reduce normal lung tissue toxicity and the other normal tissue complications.
This study also introduced a strategy to implement pretreatment dosimetric validation through reconstructing dose distribution on the synthetic-CT. This dose validation method was critical to the hypo-fractionated proton beam therapy in case of significant geometry changes. The study also suggested that the VMAT and SPArc plan could compensate for certain geometry uncertainties to meet target prescription dose coverage on synthetic-CT, while the target prescription dose coverage in IMPT was very sensitive to the anatomical change (patient #5 as an example in ). Such significant range changes along the beam paths caused dramatic target coverage degradation in the IMPT plan . The water equivalent thickness (WET) analysis based on the beam angles selected in IMPT (red dot) and arc trajectory in SPArc (blue line) was plotted in . The larger ΔWET indicated that more proton range changes through different gantry angles. Since numerous beamlets and angles were utilized in SPArc, it mitigates the dosimetric impact from such geometry uncertainty. The pretreatment dose validation platform introduced in this study and SPArc technique’s potential capabilities of mitigating range uncertainties could be critical to the single fraction SBRS.
Figure 2. (a) The fusion of plan CT and synthetic CT for patient #5. (b) DVH comparison between the nominal on plan CT and synthetic CT. (c) ΔWET between plan CT and synthetic CT as the gantry angle (IMPT: red dot, SPArc: blue line).
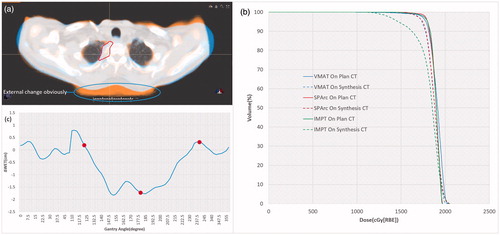
Treatment time is also one of the major clinical considerations in the spine SBRS/SBRT since some patients experience pain and have difficulty holding the treatment position during the treatment period for a long time [Citation31]. This intra-fractional motion may be caused by the long treatment delivery time, especially for patients with pain [Citation32]. In this study, we found that SPArc could offer faster treatment delivery than the VMAT plan with equivalent or better plan quality when ELST was equal to 1 s using the current PBS technique stage. Therefore, using SPArc for spine SBRS patients may significantly reduce the chance of intrafraction motion, which will help to mitigate the treatment uncertainties.
Finally, to investigate the effective spot size based the current proton system, IBA ProteusOne machine, a new plan group for SPArc with range shift was generated to compare with SPArc. The result demonstrated that SPArc performed better in terms of dosimetry (The details was list in Supplemental document Table 4s).
Conclusion
SPArc therapy is an advanced planning and treatment technique to push the dosimetric limits over the current PBS technique platform. Compared to IMPT, SPArc further improve the target coverage conformality and robustness. Compared to the VMAT technique, SPArc would be more efficient, meanwhile providing equivalent and better dosimetric plan quality for spine SBRS.
Supplemental Material
Download MS Word (1.1 MB)Disclosure statement
Xuanfeng Ding, Xiaoqiang Li, and Di Yan have a patent related to the Particle Arc Therapy (WO2017156419). The patent has been licensed to Ion Beam Application, Belgium.
Additional information
Funding
References
- Wu G, Shi Z, Chen Y, et al. A sparse representation-based radiomics for outcome prediction of higher grade gliomas. Med Phys. 2019;46(1):250–261.
- Ecker RD, Endo T, Wetjen NM, et al. Diagnosis and treatment of vertebral column metastases. Mayo Clin Proc. 2005;80(9):1177–1186.
- Joaquim AF, Ghizoni E, Tedeschi H, et al. Stereotactic radiosurgery for spinal metastases: a literature review. Einstein. 2013;11(2):247–255.
- Rief H, Chaudhri N, Tonndorf-Martini E, et al. Intensity-modulated radiotherapy versus proton radiotherapy versus carbon ion radiotherapy for spinal bone metastases: a treatment planning study. J Appl Clin Med Phys. 2015;16(6):186–194.
- Gerszten PC, Burton SA, Ozhasoglu C, et al. Radiosurgery for spinal metastases. Spine. 2007;32:193–199.
- Gerszten PC, Mendel E, Yamada Y. Radiotherapy and radiosurgery for metastatic spine disease: what are the options, indications, and outcomes? Spine. 2009;34(22 Suppl):S78–S92.
- Ryu S, James J, Gerszten P, et al. RTOG 0631 phase II/III study of image-guided stereotactic radiosurgery for localized spine metastases: phase II results. Int J Radiat Oncol. 2011;81(2):S131–S132.
- Ryu S, Yin FF, Rock J, et al. Image-guided and intensity-modulated radiosurgery for patients with spinal metastasis. Cancer. 2003;97(8):2013–2018.
- Li X, Kabolizadeh P, Yan D, et al. Improve dosimetric outcome in stage III non-small-cell lung cancer treatment using spot-scanning proton arc (SPArc) therapy. Radiat Oncol. 2018;13(1):35.
- Ding X, Li X, Qin A, et al. Have we reached proton beam therapy dosimetric limitations? – a novel robust, delivery-efficient and continuous spot-scanning proton arc (SPArc) therapy is to improve the dosimetric outcome in treating prostate cancer. Acta Oncol. 2018;57(3):435–437.
- Ding X, Li X, Qin A, et al. Redefine the role of range shifter in treating bilateral head and neck cancer in the era of intensity modulated proton therapy. J Appl Clin Med Phys. 2018;19:749–755.
- Dvorak P, Georg D, Bogner J, et al. Impact of IMRT and leaf width on stereotactic body radiotherapy of liver and lung lesions. Int J Radiat Oncol. 2005;61(5):1572–1581.
- Benedict SH, Yenice KM, Followill D, et al. Stereotactic body radiation therapy: the report of AAPM Task Group 101. Med Phys. 2010;37(8):4078–4101.
- Nill S, Tücking T, Münter MW, et al. Intensity modulated radiation therapy with multileaf collimators of different leaf widths: a comparison of achievable dose distributions. Radiother Oncol. 2005;75(1):106–111.
- MacEwan I, Chou B, Mifflin R, et al. Passively scattered proton stereotactic body radiation therapy for spine metastasis: a dosimetric analysis. Int J Radiat Oncol. 2016;96(2):E675–E676.
- Bhatt AD, Jacobson A, Lee RY, et al. High-dose proton beam-based radiation therapy in the management of extracranial chondrosarcomas. Int J Part Ther. 2016;3(3):373–381.
- Schuemann J, Dowdell S, Grassberger C, et al. Site-specific range uncertainties caused by dose calculation algorithms for proton therapy. Phys Med Biol. 2014;59(15):4007–4031.
- Paganetti H, Yu CX, Orton CG. Photon radiotherapy has reached its limit in terms of catching up dosimetrically with proton therapy: point/counterpoint. Med Phys. 2016;43(8Part1):4470–4472.
- Ding X, Li X, Zhang JM, et al. Spot-scanning proton arc (SPArc) therapy: the first robust and delivery-efficient spot-scanning proton arc therapy. Int J Radiat Oncol Biol Phys. 2016;96(5):1107–1116.
- Ryu S, Pugh SL, Gerszten PC, et al. RTOG 0631 phase 2/3 study of image guided stereotactic radiosurgery for localized (1-3) spine metastases: phase 2 results. Pract Radiat Oncol. 2014;4(2):76–81.
- Elibe E, Boyce-Fappiano D, Ryu S, et al. Stereotactic radiosurgery for primary tumors of the spine and spinal cord. J Radiosurgery SBRT. 2018;5:107–113.
- Paganetti H, Niemierko A, Ancukiewicz M, et al. Relative biological effectiveness (RBE) values for proton beam therapy. Int J Radiat Oncol. 2002;53(2):407–421.
- Liu G, Li X, Zhao L, et al. A novel energy sequence optimization algorithm for efficient spot-scanning proton arc (SPArc) treatment delivery. Acta Oncol. 2020;59:1178–1185.
- Kang KH, Okoye CC, Patel RB, et al. Complications from stereotactic body radiotherapy for lung cancer. Cancers. 2015;7(2):981–1004.
- Quan EM, Li X, Li Y, et al. A comprehensive comparison of IMRT and VMAT plan quality for prostate cancer treatment. Int J Radiat Oncol. 2012;83(4):1169–1178.
- Aoyama H, Westerly DC, Mackie TR, et al. Integral radiation dose to normal structures with conformal external beam radiation. Int J Radiat Oncol. 2006;64(3):962–967.
- Qin A, Gersten D, Liang J, et al. A clinical 3D/4D CBCT-based treatment dose monitoring system. J Appl Clin Med Phys. 2018;19(6):166–176.
- Schultheiss TE, Kun LE, Ang KK, et al. Radiation response of the central nervous system. Int J Radiat Oncol. 1995;31(5):1093–1112.
- Sahgal A, Weinberg V, Ma L, et al. Probabilities of radiation myelopathy specific to stereotactic body radiation therapy to guide safe practice. Int J Radiat Oncol. 2013;85(2):341–347.
- Bedford JL, Thomas MDR, Smyth G. Beam modeling and VMAT performance with the agility 160-leaf multileaf collimator. J Appl Clin Med Phys. 2013;14(2):172–185.
- Wu QJ, Yoo S, Kirkpatrick JP, et al. Volumetric arc intensity-modulated therapy for spine body radiotherapy: comparison with static intensity-modulated treatment. Int J Radiat Oncol. 2009;75(5):1596–1604.
- Li W, Sahgal A, Foote M, et al. Impact of immobilization on intrafraction motion for spine stereotactic body radiotherapy using cone beam computed tomography. Int J Radiat Oncol. 2012;84(2):520–526.