Introduction
When treating breast cancer patients with external beam radiotherapy, deep inspiration breath hold (DIBH) has proven to be a valuable method to decrease dose to the heart and lungs [Citation1–4]. Several commercial methods to monitor and regulate respiration during DIBH exist [Citation5], and the most common methods are: external surrogate with an optical or infrared camera [Citation1,Citation3,Citation4,Citation6–10], spirometry [Citation11], or surface monitoring with optical cameras and structured light [Citation12–15]. When using a reflective surrogate, the closer the surrogate is placed to the treated breast in the cranio-caudal and lateral direction the better it represents the motion of the breast [Citation8,Citation16,Citation17]. However, when positioning the surrogate close to the target, the surrogate will often be positioned in the path of the treatment beam. This may lead to an unwanted bolus effect, increasing the skin dose, thereby increasing the risk of erythema and skin irritation [Citation18,Citation19].
The commercially available two-marker real-time position management (RPM) box (TMB) was not suitable for placement within the treatment beams due the amount of material it consists of, as this may result in an unwanted skin reaction. Furthermore, due to the relatively wide lateral extent of the TMB, it may be positioned with a tilt toward the treated breast on some patients [Citation16]. Therefore, a novel respiratory surrogate (NRS) was designed consisting of less material and with a smaller footprint to (1) allow placement within the treatment beam and (2) enable a stable placement of the reflective surrogate on the sternum approximately at the level of the papilla.
In this study, we compared surface dose and water-equivalent thickness between the NRS and the TMB by radiosensitive film measurements. Tracking performance of the two types of surrogates was investigated in both a phantom and in clinical setting.
Material and methods
Novel respiratory surrogate (NRS)
The NRS () was made from a non-reflective, matte light gray polyester sheet. To emulate the TMB in the infrared view of the RPM system, two circular reflective markers were affixed on the stalk of the NRS. The markers on the NRS were raised above the base, to improve their visibility on the monitoring system. To ease the positioning of the NRS with the lasers used for patient setup, a vertical line was printed through the centers of the reflective marker positions. The NRS is disposable and meant for single patient use, for hygienic reasons. The NRS was bought from manufacturer Langhoff Teknik, Denmark (contact e-mail: [email protected]) at a price of €1.2 per surrogate (compared to the price of the TMB of €206). The NRS design is not copyright or patent protected and can therefore be reproduced freely. The design details are presented in the Supplementary material.
Figure 1. The dimensions of the two surrogates: Two-marker RPM box (TMB) to the left and novel respiratory surrogate (NRS) to the right. The NRS has a base with a diameter of 38 mm and reflective markers affixed on a stalk with 30 mm spacing between them, i.e., the same distance as on the TMB. The stalk of the surrogate was 60 mm with the reflective markers elevated 21 mm above the base.
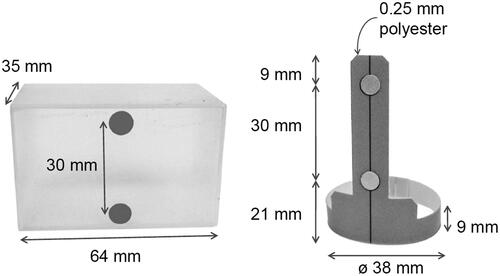
Film measurements
To assess surface dose to the patient, Gafchromic EBT3™ film was placed horizontally at 100 cm SSD on a 30 × 30 × 5 cm3 thick Solid Water™ block for backscatter. The film was irradiated at an incident angle of 0° with a 10 × 10 cm2 6 MV flat photon beam delivering 5 Gy at the peak of the depth-dose curve. Irradiations were performed with the TMB, the NRS, and without any surrogates. Water-equivalent thickness was measured by acquiring percentage depth-dose curves with and without any surrogates. This irradiation was performed with the Gafchromic EBT3™ film placed vertically on a 10 cm thick water equivalent backscatter block and sandwiched between two 30 × 30 × 5 cm3 Solid Water™ blocks and aligned to the top horizontal surface. In this way, the depth-dose curve was obtained from depth 0 cm to maximum of 4.5 cm. Each film was scanned three times following the procedure suggested by Ferreira et al. [Citation20]
Tracking performance
We used the RPM™ system version 1.7 (Varian Medical Systems Inc, Palo Alto, CA, USA) for tracking and monitoring as described previously [Citation7].
The tracking of the two surrogates was tested with both a wall-mounted installation in a treatment bunker and a couch-mounted installation at a CT-scanner. To control the movement of the surrogates, a Scandidos Hexamotion™ motion platform (Scandidos, Uppsala, Sweden) was programed to perform a vertical motion with 15 mm peak to peak amplitude with a 4 s period. The surrogates were placed on the platform one at a time at the reference location (i.e., isocenter for a wall-mounted installation). Inter- and intra-system performance was investigated by repeating the measurement 5 times with each surrogate. The influence of surrogate angle was measured by tilting the surrogates on the platform by 3°, 6°, 9°, 15°, 20°, and 30°. The tilts were performed as rotation around the lateral and longitudinal axes using a lockable hinged platform.
Clinical performance during treatment
Twenty consecutive female breast cancer patients, treated with external beam radiotherapy between November 2016 and February 2017 at the Department of Oncology at Rigshospitalet (Copenhagen, Denmark) were retrospectively selected for this study. Ten patients were monitored with the TMB and 10 with the NRS. All patients were coached, CT-scanned for radiotherapy planning, and treated in DIBH with visual feedback. During their course of treatment (3DCRT to 50 Gy in 25 fractions), all patients were daily image-guided with orthogonal 2D kV images, in addition weekly portal MV verification images of the medial treatment field were acquired. Patient data was collected as part of routine clinical quality control and was therefore not subject to ethical approval.
The NRS was positioned on a stable part at the caudal end of the sternum with the reflective markers at the midline of the patient. The TMB was positioned similarly. Both surrogates were held in place with a piece of tape and the surrogate’s midline position was marked with a tattoo.
Two portal verification images from different fractions acquired during the last half of the treatment for each patient were registered (translations only) to the digitally reconstructed radiograph (DRR). The residual shift was compared between patients monitored using NRS and TMB.
Non-parametric statistics (Mann–Whitney) were used to compare the data and p < .05 was considered significant.
Results
Surface dose
shows the dose under the centerline of the surrogates and surface dose films. The TMB resulted in a higher surface dose over a larger area compared to the NRS. Surface dose was increased by up to 235% for the TMB and 45% for the NRS compared to no surrogate. The area of at least 25% surface dose increase was 71.7 cm2 and 9.7 cm2 for the TMB and NRS, respectively.
Figure 2. Surface dose measured directly under the two-marker RPM box (TMB) and the novel respiratory surrogate (NRS) when exposed to 500 MU in a 10 × 10 cm2 field of 6 MV photons. Graph (A) shows the profiles below the centerline of the TMB (red), NRS (blue), and without any surrogates (magenta). The 2D profiles inserted below the graph show the surface dose directly below the TMB (B) and NRS (C). The surrogates were positioned with the reflective markers facing right in the image.
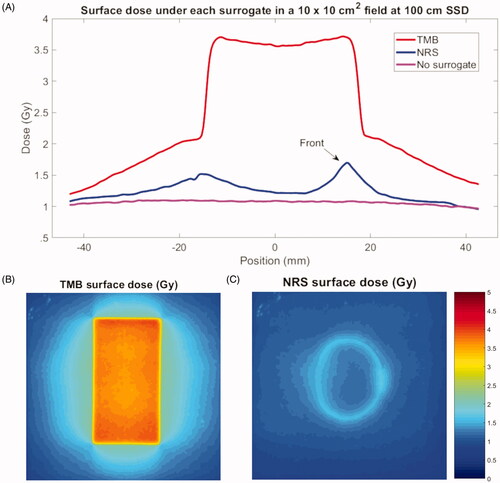
Water-equivalent thickness
The percentage depth-dose curves obtained for the two surrogates and without any surrogate were used to determine the water-equivalent thickness of the surrogates. The TMB was found to be equivalent to 3.5 mm water under the center and 5.0 mm under the edges, while the NRS was found to be equivalent to 0.9 mm of water under the stalk and less everywhere else.
Tracking of the respiratory signal
The inter- and intra-system differences were < 0.1 mm for both surrogates for repeated measurements. The measured vertical motion amplitudes were 14.75 ± 0.05 and 15.05 ± 0.05 mm (mean ± SD) for the NRS and TMB, respectively, when measured with the untilted surrogate. When tilting the surrogates, a larger tilt resulted in a larger measured amplitude, but similar for both surrogates; this has been previously reported for the TMB [Citation16]. No significant differences between the surrogates were found in tracking performance, regardless of system mounting (wall or couch) or tilt (p > .05).
Clinical performance during treatment
The patients’ median age (range) was 55 years (31–81 years) and 58 years (37–76 years) for the group with the TMB and the NRS, respectively. A total of 20 fractions were analyzed for each patient group. Mean values and standard deviations of the residual shift of the portal verification images for patients treated with the TMB and NRS were 3.6 ± 0.7 mm and 3.7 ± 1.1 mm, respectively, with no significant difference between the two surrogates when in the clinical use (p > .05).
Discussion
We have compared two respiratory surrogates, the NRS and the TMB, with respect to skin dose and motion tracking capabilities. The use of the NRS resulted in less skin dose. No significant differences were observed regarding tracking capabilities either in a clinical or in a phantom setting.
Several studies have shown that a surrogate/marker positioned on the xiphoid process or the sternum improved correlation to breast/thoracic wall compared to a position on the abdomen [Citation11,Citation17,Citation21], in general the closer the surrogate is placed to the breast in cranio-caudal and lateral directions the better [Citation8,Citation16]. NRS’ narrow base enabled positioning of the surrogate on the sternum at the level of the papilla.
The measurements of the skin dose increase from the TMB in a direct beam showed that it corresponded to a 3.5–5.0 mm bolus, which agreed well with a previously reported result of 3 mm [Citation16]. This bolus effect could lead to an increased risk of erythema in breast cancer patients [Citation16]. The NRS corresponded to less than 1.0 mm bolus which still increased the risk of erythema but to a much lesser extent than the TMB [Citation18]. We have not observed any skin reaction related to the NRS in the more than 1000 breast cancer patients treated using this surrogate.
Limitations in this work include that only one marker of each type was investigated, and the relatively small number of patients included. Due to relatively large differences between the two surrogates in surface dose, investigating several markers of each type is unlikely to alter our conclusion. The reflective markers on the NRS are elevated above the base to allow the surrogate to be tracked even on patients where the abdomen may occlude the visibility of the marker closest to the surface of the patient. We have not tested it systematically, but our clinical experience is that the NRS is trackable for most patients without compromising surrogate position. The commercial six-marker RPM box was not included in the analysis as it is not used in our clinics and is physically very similar to the TMB. Using 2D MV images to determine 3D shift has some uncertainties but this limitation does not affect the total displacement.
Conclusions
The novel respiratory motion surrogate improved skin sparing and had equivalent tracking performance compared to Varian’s commercially available two-marker RPM box, when positioned on the sternum of the patient. The NRS has been put into clinical use at Rigshospitalet and Naestved Hospital for all breast cancer and other patients, treated in DIBH, and monitored with the RPM system.
Supplemental Material
Download MS Word (82.4 KB)Acknowledgments
The authors wish to acknowledge Dennis Langhoff of Langhoff Teknik for his assistance in developing the novel surrogate and providing working prototypes.
Disclosure statement
The authors have no conflicts of interest to disclose.
References
- Bruzzaniti V, Abate A, Pinnarò P, et al. Dosimetric and clinical advantages of deep inspiration breath-hold (DIBH) during radiotherapy of breast cancer. J Exp Clin Cancer Res. 2013;32:88– 88.
- Borst GR, Sonke JJ, den Hollander S, et al. Clinical results of image-guided deep inspiration breath hold breast irradiation. Int J Radiat Oncol Biol Phys. 2010;78(5):1345–1351.
- Pedersen AN, Korreman S, Nyström H, et al. Breathing adapted radiotherapy of breast cancer: reduction of cardiac and pulmonary doses using voluntary inspiration breath-hold. Radiother Oncol. 2004;72(1):53–60.
- Hjelstuen MHB, Mjaaland I, Vikström J, et al. Radiation during deep inspiration allows loco-regional treatment of left breast and axillary-, supraclavicular- and internal mammary lymph nodes without compromising target coverage or dose restrictions to organs at risk. Acta Oncol. 2012;51(3):333–344.
- Boda-Heggemann J, Knopf AC, Simeonova-Chergou A, et al. Deep inspiration breath hold-based radiation therapy: a clinical review. Int J Radiat Oncol Biol Phys. 2016;94(3):478–492.
- Latty D, Stuart KE, Wang W, et al. Review of deep inspiration breath-hold techniques for the treatment of breast cancer. J Med Radiat Sci. 2015;62(1):74–81.
- Damkjaer SMS, Aznar MC, Pedersen AN, et al. Reduced lung dose and improved inspiration level reproducibility in visually guided DIBH compared to audio coached EIG radiotherapy for breast cancer patients. Acta Oncol. 2013;52(7):1458–1463.
- Lutz CM, Poulsen PR, Fledelius W, et al. Setup error and motion during deep inspiration breath-hold breast radiotherapy measured with continuous portal imaging. Acta Oncol. 2016;55(2):193–200.
- Stranzl H, Zurl B, Langsenlehner T, et al. Wide tangential fields including the internal mammary lymph nodes in patients with left-sided breast cancer. Influence of respiratory-controlled radiotherapy (4D-CT) on cardiac exposure. Strahlenther Onkol. 2009;185(3):155–160.
- McIntosh A, Shoushtari AN, Benedict SH, et al. Quantifying the reproducibility of heart position during treatment and corresponding delivered heart dose in voluntary deep inhalation breath hold for left breast cancer patients treated with external beam radiotherapy. Int J Radiat Oncol Biol Phys. 2011;81(4):e569–e576.
- Mittauer K, Deraniyagala R, Li JG, et al. Monitoring ABC-assisted deep inspiration breath hold for left-sided breast radiotherapy with an optical tracking system. Med Phys. 2015;42(1):134–143.
- Cerviño LI, Gupta S, Rose MA, et al. Using surface imaging and visual coaching to improve the reproducibility and stability of deep-inspiration breath hold for left-breast-cancer radiotherapy. Phys Med Biol. 2009;54(22):6853–6865.
- Tang X, Zagar TM, Bair E, et al. Clinical experience with 3-dimensional surface matching-based deep inspiration breath hold for left-sided breast cancer radiation therapy. Pract Radiat Oncol. 2014;4(3):e151–e158.
- Kalet AM, Cao N, Smith WP, et al. Accuracy and stability of deep inspiration breath hold in gated breast radiotherapy – a comparison of two tracking and guidance systems. Phys Med. 2019;60:174–181.
- Wikström K, Isacsson U, Nilsson K, et al. Reproducibility of heart and thoracic wall position in repeated deep inspiration breath holds for radiotherapy of left-sided breast cancer patients. Acta Oncol. 2018;57(10):1318–1324.
- Conroy L, Guebert A, Smith WL. Technical note: issues related to external marker block placement for deep inspiration breath hold breast radiotherapy. Med Phys. 2017;44(1):37–42.
- Skyttä T, Kapanen M, Laaksomaa M, et al. Improving the reproducibility of voluntary deep inspiration breath hold technique during adjuvant left-sided breast cancer radiotherapy. Acta Oncol. 2016;55(8):970–975.
- Bray FN, Simmons BJ, Wolfson AH, et al. Acute and chronic cutaneous reactions to ionizing radiation therapy. Dermatol Ther. 2016;6(2):185–206.
- Ryan JL. Ionizing radiation: the good, the bad, and the ugly. J Invest Dermatol. 2012;132(3 Pt 2):985–993.
- Ferreira BC, Lopes MC, Capela M. Evaluation of an Epson flatbed scanner to read Gafchromic EBT films for radiation dosimetry. Phys Med Biol. 2009;54(4):1073–1085.
- Stock M, Kontrisova K, Dieckmann K, et al. Development and application of a real-time monitoring and feedback system for deep inspiration breath hold based on external marker tracking. Med Phys. 2006;33(8):2868–2877.