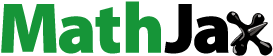
Introduction
Recurrences after radiochemotherapy for head and neck cancer (HNC) are often located within the region that received a high dose during the primary radiotherapy [Citation1]. Moreover, there is an association between areas of high standardized uptake value (SUV) within the tumor, as seen on positron emission tomography/computed tomography (PET/CT) images with the tracer 2-deoxy-2-[F-18] fluoro-D-glucose (FDG), and poor treatment response [Citation2]. This relationship seems to be stronger for HNC which is not related to human papilloma virus (HPV) [Citation3].
The use of highly conformal and tailor-made dose distributions employing intensity modulated radiotherapy (IMRT) and similar approaches facilitate dose escalation of radioresistant tumor regions without exceeding the tolerance doses to the organs at risk (OARs) [Citation4]. FDG-PET guided dose painting is one method where the putative radioresistant regions may be segmented based on relative uptake levels of FDG within the tumor. Dose painting of HNC has been investigated in several pilot studies [Citation5–10] and in two randomized trials of which one is closed [Citation11] and one is still ongoing [Citation12]. So far, the results have been promising, but an increase in late grade 3–4 side-effects has been reported. Particularly, late mucosal ulcers (LMU) have been a concern [Citation9,Citation11]. Olteanu et al. [Citation13] investigated the relationship between dose–volume parameters and toxicity (late grade 4 mucosal ulcers) and suggested a dose-volume constraint of D1.75 cc = 84 Gy, where D1.75 cc is the minimum dose to 1.75 cc of the gross tumor volume (GTV) receiving the highest dose.
The aim of this study was to develop a mucosa-sparing dose painting by contours (DPBC) protocol by including the mucosa as an OAR with a defined hard dose constraint in the treatment planning. More specifically, we wished to assess the frequency of DPBC treatment plans with acceptable coverage of the dose painting targets inside the GTV. The dose painting plans were also compared to standard plans with emphasis on both target coverage and doses to OARs. To our knowledge, this is the first study investigating mucosa-sparing dose painting for HNC.
Material and methods
Patient material
The patients were included applying the eligibility criteria of the RADPAINT study (NCT03847480) enrolling patients with HPV-unrelated cancer, having a poor prognosis with a potentially large gain from intensified treatment such as dose painting [Citation3]. Key inclusion criteria were histologically or cytologically verified squamous cell carcinomas of the head and neck, age over 18 years, curative intent and World Health Organization performance status 0–2. The exclusion criteria were T1 N0-1 TNM-classification [Citation14], T1-2 glottic cancer, HPV-related oropharyngeal cancer, cancer of the soft palate and diabetes mellitus. We chose to include 15 patients as this seemed as a reasonable number to assess the primary objective; the frequency of DPBC treatment plans with 90% (volume) coverage of the dose painting targets inside the GTV. We included patients from two cohorts: (1) The first five patients in the RADPAINT study and (2) 10 previously 1 Hospital (Supplemental Table 1). These patients were included consecutively from a list of all patients (2007–2013) with planning PET/CT (Moan et al.), starting with the most recent patients meeting the eligibility criteria of the RADPAINT study.
Delineation of target volumes and organs at risk
Our institution, Oslo University Hospital, uses a slightly modified version of the DAHANCA delineation guidelines [Citation15]. The GTVs were delineated on the PET/CT images by an experienced head and neck oncologist. The clinical target volume was an isotropic expansion of the GTV by 1 cm, adjusted for anatomical barriers. The planning target volumes (PTV) margin was 3 mm, and the same 3 mm margin was used to generate a planning organ at risk volume (PRV) for the spinal cord. Furthermore, the parotid glands and the swallowing structures were delineated [Citation16].
The mucosa OAR was defined by a 3 mm border around the lumen of the pharynx/oral cavity. Based on the findings by Olteanu et al. [Citation13], where a constraint on D1.75 cc of 84 Gy was suggested, our RADPAINT study employs an even more conservative constraint; D1.75 cc_mucosa < 80 Gy.
Dose painting target volumes
We used a modified version of a formula proposed by Vanderstraeten et al. [Citation17] giving the relationship between the prescribed dose and PET intensity:
(1)
(1)
The method was initially used for dose painting by numbers, but by defining target threshold dose levels, it can be used for DPBC. Ihigh is defined as 95% of the maximum intensity within the GTV, and Ilow is defined as 25% of Ihigh. In this study, the following fraction doses were used; Dhigh = 2.9 Gy and Dlow = 2.0 Gy.
Treatment planning
Treatment planning was done using RayStation 7.0 (Raysearch Labs AB, Sweden) with the volumetric modulated arc therapy (VMAT) technique for Varian TrueBeam (Varian Medical Systems Inc., Palo Alto, CA, USA) linear accelerators. Our standard treatment consisted of a simultaneously integrated boost plan with 1.59 Gy/1.88 Gy/2.00 Gy to the elective region, CTV and GTV, respectively for 34 fractions, with six fractions delivered per week.
The standard treatment plan was optimized with the following prioritization, in order: (1) Maximum dose (Dmax) to the spinal cord < 48 Gy. (2) Coverage of the target volumes, defined as D98 > 95% of prescribed dose to all PTVs and D99 > 95% for all CTVs. (3) Sparing of the parotid glands, aiming for an average dose less than 26 Gy. (4) Sparing of the swallowing structures reducing the average dose below 45 Gy. Two prescription volumes within the GTV were defined for dose painting: PV33 and PV66. The dose painting plan was generated by re-optimizing the standard plan, while trying to achieve 2.3 Gy (D90%) per fraction to the PV33 and 2.6 Gy (D90%) per fraction to the PV66. The maximum dose was kept below 2.9 Gy per fraction. The dose within PV33/PV66 was allowed to increase from one threshold level to the next toward the maximum allowed dose of 2.9 Gy per fraction, giving a continuous ‘mound-shaped’ dose distribution instead of discrete levels in the two dose painting volumes (PV33 and PV66). The additional priority for the dose painting plan compared with the standard plan was D1.75 cc_mucosa (dose per fraction) < 2.71 Gy.
Our proposed two-tiered treatment scheme was to use the dose painting plan for the first 17 fractions (2.0–2.9 Gy per fraction) and then switch to the standard plan (2.0 Gy per fraction). The spatiotemporal stability of FDG-PET images of HNC during radiotherapy is not known. Signs of increased inflammation in and around the tumor on the PET images after two weeks of radiotherapy has been reported [Citation8], thus obscuring the true remaining FDG uptake in the tumor cells. We therefore chose to limit dose painting to the first three weeks in line with the Ghent approach [Citation6,Citation8]. Our goal was to establish a solution which was clinically feasible. Thus, we decided not to employ imaging during treatment, but instead switch to conventional radiotherapy (without dose painting) after three weeks based on the first planning FDG- PET/CT. The resulting constraint on the D1.75 cc_mucosa was 80 Gy (17 × 2.71 Gy + 17 × 2.0 Gy).
To evaluate our proposed treatment protocol PLANDP, consisting of 17 fractions of dose painting and 17 fractions of standard treatment, it was compared to a plan with of 34 fractions of standard treatment (PLANSTD). The dose–volume data for the parallel OAR from PLANSTD and PLANDP were compared using Wilcoxon signed rank test. Data handling and statistical analyses were performed using SPSS® 26 (International Business Machines Corporation, Armonk, NY, USA).
Results
The average volume of the total tumor burden (total volume of primary tumor and affected lymph nodes; union of all GTVs) for the 15 patients was 34.9 cc. The mean PV33 and PV66 were 12.3 and 3.5 cc, respectively (Supplemental Table 2). shows an example of PLANSTD and PLANDP, in addition to the original PET/CT and PV delineation. Fourteen out of 15 patients could be dose escalated with dose painting without being restricted by D1.75 cc_mucosa and achieving adequate target coverage, D90% > 73.1 Gy and 78.2 Gy of PV33 and PV66, respectively. The coverage of PV33 was high for all patients, as the relative volume of the target inside the 73.1 Gy isodose line was 98.5% (95.5-100). For three patients, 90% of the PV66 was not covered by 78.2 Gy, and on average 94.3% (82.7-100) was covered. Apart from the single patient where the D1.75 cc_mucosa was the restricting factor, air (pharyngeal lumen) close to PV66 was restricting the dose coverage in the other two patients).
Figure 1. (A) FDG/PET CT showing the high uptake region of the primary tumor (stage T2 N0 M0, tonsillar carcinoma). (B) Delineations; Cyan = PV33, Brown = PV66, Purple = Mucosa. (C) Dose distribution of a standard plan. Green isodose = 54 Gy. Yellow isodose = 64 Gy. Red isodose = 68 Gy. (D) Dose distribution from the dose painting plan. In addition to the standard isodoses the cyan isodose = 73.1 Gy and the purple isodose = 78.2 Gy. (E) Distribution of the escalated doses, 73.1 Gy (cyan) and 78.2 Gy (purple) isodose in relation to the mucosa (pink region of interest [ROI]). (F) Zoomed in view of (E) showing the sparing of the mucosa (pink ROI).
![Figure 1. (A) FDG/PET CT showing the high uptake region of the primary tumor (stage T2 N0 M0, tonsillar carcinoma). (B) Delineations; Cyan = PV33, Brown = PV66, Purple = Mucosa. (C) Dose distribution of a standard plan. Green isodose = 54 Gy. Yellow isodose = 64 Gy. Red isodose = 68 Gy. (D) Dose distribution from the dose painting plan. In addition to the standard isodoses the cyan isodose = 73.1 Gy and the purple isodose = 78.2 Gy. (E) Distribution of the escalated doses, 73.1 Gy (cyan) and 78.2 Gy (purple) isodose in relation to the mucosa (pink region of interest [ROI]). (F) Zoomed in view of (E) showing the sparing of the mucosa (pink ROI).](/cms/asset/dfb1f5b2-aa2e-4428-886f-af9e8901c359/ionc_a_2022200_f0001_c.jpg)
All plans had an acceptable D1.75 cc_mucosa with a population average of 74.5 Gy (67.4–79.7). The overall highest D1.75 cc in the GTV was 80.4 Gy (76.6–82.1). The maximum summed dose to a point in any of the PLANDP was 83.1 Gy (80.1−84.1). The contralateral and ipsilateral parotid glands received the same average dose in both PLANSTD and PLANDP; 22.0 Gy and 27.0 Gy, respectively. For all plans, Dmax for the spinal cord and spinal cord PRV was kept below 48 Gy and 52 Gy, respectively. Considering the swallowing OARs, only the dose to the base of tongue and the oral cavity was significantly increased by dose painting (p < 0.05), receiving on average 1 Gy higher mean dose compared to the standard treatment. Eight out of 15 patients had primary disease involving the oral cavity or oropharynx. For the remaining seven patients, only the dose to the base of tongue was significantly increased by dose painting (p = 0.046; and Supplemental Table 3).
Figure 2. Dose difference (ΔDose) between mean doses from the dose painting and standard treatment for organs at risk. BOT: Base of tongue, CM: Cricopharyngeal muscle, EIM: Esophageal Inlet Muscle, SGL: Supra Glottic Larynx, GL: Glottic Larynx, S/M/IPCM: Superior/Middle/Inferior Pharyngeal Constrictor Muscle. The box-plot shows the Q1–Q3 range, median, and min and max unless outliers exists. For plots with outliers, 1.5 × inter-quartile range is used. Only ΔDose for BOT and oral cavity was statistically significant (*p < 0.05).
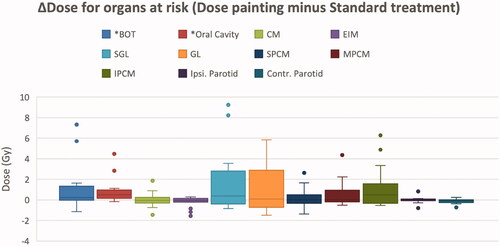
Discussion
The main aim of this study was to investigate a strategy to escalate the dose to sub-volumes of the GTV using a two-level DPBC technique while restricting the dose to the mucosa and other OARs. Late mucosal ulcers (LMU) represent the most important clinical challenge related to dose painting of HNC. High-grade LMUs have devastating consequences. In the dose painting study of Rasmussen et al., the dose to the PET-positive part of the GTV was 79.6 Gy. They observed three cases of high-grade LMU out of 15 included patients. Two out of these three patients had soft palate tumors and showed persistent ulceration five months after treatment. In one of the patients the ulceration worsened and penetrated the soft palate. This is a serious complication with a major impact on speech and eating and thereby reducing the quality of life. In the study on LMU performed by the Ghent group, nine out of 39 patients had high-grade LMU [Citation13]. Spontaneous healing was seen in only two of these patients. In one patient, bleeding from the ulceration led to death after eight months. It was also found that the ulcers had a predilection for thin mucosal layers covering bone and cartilage. Surprisingly, there was no obvious relation to the size of the GTV, but as mentioned previously, doses above 84 Gy to the high-dose D1.75 cc were associated with the risk of LMU.
In the present study, for 14 out of 15 patients the mucosa was not limiting the dose escalation. For the last patient, the D1.75 cc_mucosa exceeded 80 Gy, limiting the dose to the highest prescription volume (PV66). This patient had a large GTV of about 60 cc and a large PV33 of 20 cc. The PV66, however, was 3.9 cc, which was slightly above the population average (3.5 cc). On the other hand, the PV66 was located close to and along the pharyngeal lumen, and thus, the mucosa dose constraint led to reduced coverage. Still, the PV33 and PV66 was significantly escalated compared to the standard treatment, and even with the limitation on D1.75 cc_mucosa, an average dose of around 79 Gy in the PV66 was achieved. When comparing the D1.75 cc_mucosa with the global D1.75 cc, it is evident that actively restricting the mucosa dose is possible while still achieving a high dose within the tumor.
For the parotid glands, there was no significant differences in dose when comparing PLANSTD with PLANDP. This has also been seen in several previous in silico studies [Citation18–21] and supported by the findings in clinical trials [Citation10–12]. This may be explained by the often sufficient separation between the HNC target volumes and the parotid glands, leading to comparable doses for PLANSTD and PLANDP. However, for the swallowing OARs, there was a statistically significant increase in dose to the base of tongue and oral cavity in the order of 1 Gy. Logically, this dose increase was more pronounced when the target volume was in the oropharynx or the oral cavity. It is unlikely that this small dose increase will result in more toxicity, regardless of the statistical significance. Skorska et al. did a treatment planning study with focus on the OAR doses [Citation21]. They found an increase in the dose to the swallowing structures following dose painting. However, the maximum dose was rather high, 95 Gy, which is expected to increase the rate of dysphagia as seen in the clinical data from the Ghent group using a maximum dose of 92 Gy [Citation11]. In an interim analysis from the Tübingen group on hypoxia dose painting with a moderately increased dose of 77 Gy, no increase in dysphagia was found [Citation12]. Also, Liu et al. got similar results in a nonrandomized study on nasopharyngeal cancer with doses up to 78 Gy [Citation10]. To summarize, the maximum dose level in dose painting is most likely as an important risk factor of dysphagia as it is for late mucosal ulcers [Citation13]. It seems that a local dose escalation within the GTV to 80 Gy may be safe, while more than 90 Gy may lead to unacceptable toxicity.
Conclusion
A two-tiered FDG PET-guided DPBC is feasible without exceeding presumed safe dose-volume constraints for the mucosa. There should be no clinically significant increase in late mucosal ulcers with the approach used in our clinical trial.
Ethical approval
The study has been reviewed and approved by the Regional Committees for Medical and Health research Ethics (REK) according to Norwegian law and regulations and the Institutional Review Board. The five patients included in the RADPAINT study (NCT03847480) gave informed consent. Exemption from study-specific informed consent was granted by REK for the other 10 patients as this is a retrospective study and the patients are de-identified.
Supplemental Material
Download MS Word (16.3 KB)Supplemental Material
Download MS Word (219.9 KB)Disclosure statement
The authors report no conflicts of interest.
References
- Johansen S, Norman MH, Dale E, et al. Patterns of local-regional recurrence after conformal and intensity-modulated radiotherapy for head and neck cancer. Radiat Oncol. 2017;12(1):87.
- Machtay M, Natwa M, Andrel J, et al. Pretreatment FDG-PET standardized uptake value as a prognostic factor for outcome in head and neck cancer. Head Neck. 2009;31(2):195–201.
- Moan JM, Amdal CD, Malinen E, et al. The prognostic role of 18F-fluorodeoxyglucose PET in head and neck cancer depends on HPV status. Radiother Oncol. 2019;140:54–61.
- Gregoire V, Langendijk JA, Nuyts S. Advances in radiotherapy for head and neck Cancer. J Clin Oncol. 2015;33(29):3277–3284.
- Duprez F, De Neve W, De Gersem W, et al. Adaptive dose painting by numbers for head-and-neck cancer. Int J Radiat Oncol Biol Phys. 2011;80(4):1045–1055.
- Madani I, Duprez F, Boterberg T, et al. Maximum tolerated dose in a phase I trial on adaptive dose painting by numbers for head and neck cancer. Radiother Oncol. 2011;101(3):351–355.
- Madani I, Duthoy W, Derie C, et al. Positron emission tomography-guided, focal-dose escalation using intensity-modulated radiotherapy for head and neck cancer. Int J Radiat Oncol, Biol, Phys. 2007;68(1):126–135.
- Berwouts D, Olteanu LA, Duprez F, et al. Three-phase adaptive dose-painting-by-numbers for head-and-neck cancer: initial results of the phase I clinical trial. Radiother Oncol. 2013;107(3):310–316.
- Rasmussen JH, Hakansson K, Vogelius IR, et al. Phase I trial of 18F-Fludeoxyglucose based radiation dose painting with concomitant cisplatin in head and neck cancer. Radiother Oncol. 2016;120(1):76–80.
- Liu F, Xi XP, Wang H, et al. PET/CT-guided dose-painting versus CT-based intensity modulated radiation therapy in locoregional advanced nasopharyngeal carcinoma. Radiat Oncol. 2017;12(1):15.
- Berwouts D, Madani I, Duprez F, et al. Long-term outcome of 18 F-fluorodeoxyglucose-positron emission tomography-guided dose painting for head and neck cancer: Matched case-control study. Head Neck. 2017;39(11):2264–2275.
- Welz S, Monnich D, Pfannenberg C, et al. Prognostic value of dynamic hypoxia PET in head and neck cancer: Results from a planned interim analysis of a randomized phase II hypoxia-image guided dose escalation trial. Radiother Oncol. 2017;124(3):526–532.
- Olteanu LAM, Duprez F, De Neve W, et al. Late mucosal ulcers in dose-escalated adaptive dose-painting treatments for head-and-neck cancer. Acta Oncol. 2018;57(2):262–268.
- Lydiatt WM, Patel SG, O'Sullivan B, et al. Head and neck cancers-major changes in the american joint committee on cancer eighth edition cancer staging manual. CA Cancer J Clin. 2017;67(2):122–137.
- Jensen K, Friborg J, Hansen CR, et al. The danish head and neck cancer group (DAHANCA) 2020 radiotherapy guidelines. Radiother Oncol. 2020;151:149–151.
- Brouwer CL, Steenbakkers RJ, Bourhis J, et al. CT-based delineation of organs at risk in the head and neck region: DAHANCA, EORTC, GORTEC, HKNPCSG, NCIC CTG, NCRI, NRG oncology and TROG consensus guidelines. Radiother Oncol. 2015;117(1):83–90.
- Vanderstraeten B, Duthoy W, De Gersem W, et al. [18F]fluoro-deoxy-glucose positron emission tomography ([18F]FDG-PET) voxel intensity-based intensity-modulated radiation therapy (IMRT) for head and neck cancer. Radiother Oncol. 2006;79(3):249–258.
- Skjotskift T, Evensen ME, Furre T, et al. Dose painting for re-irradiation of head and neck cancer. Acta Oncol. 2018;57(12):1693–1699.
- Lee NY, Mechalakos JG, Nehmeh S, et al. Fluorine-18-labeled fluoromisonidazole positron emission and computed tomography-guided intensity-modulated radiotherapy for head and neck cancer: a feasibility study. Int J Radiat Oncol Biol Phys. 2008;70(1):2–13.
- Thorwarth D, Eschmann SM, Paulsen F, et al. Hypoxia dose painting by numbers: a planning study. Int J Radiat Oncol Biol Phys. 2007;68(1):291–300.
- Skorska M, Piotrowski T, Ryczkowski A. Comparison of dose distribution for head and neck cancer patients with and without dose painting escalation during radiotherapy realized with tomotherapy unit. Br J Radiol. 2017;90(1075):20170019.