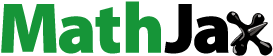
Abstract
Introduction
Hodgkin lymphoma (HL) is a highly curable hematological malignancy. Consolidation radiation therapy techniques have made significant progresses to improve organ-at-risk sparing in order to reduce late radiation-induced toxicity. Recent technical breakthroughs notably include intensity modulated proton therapy (IMPT), which has demonstrated a major dosimetric benefit at the cardiac level for mediastinal HL patients. However, its implementation in clinical practice is still challenging, notably due to the limited access to proton therapy facilities. In this context, the purpose of this study was to estimate the benefit of IMPT for HL proton therapy for diverse cardiac adverse events and to propose a general frame for mediastinal HL patient selection strategy for IMPT based on cardiotoxicity reduction, patient clinical factors, and IMPT treatment availability.
Material and methods
This retrospective dosimetric study included 30 mediastinal HL patients treated with VMAT. IMPT plans were generated on the initial simulation scans. Dose to the heart, to the left ventricle and to the valves were retrieved to calculate the relative risk (RR) of ischemic heart disease (IHD), congestive heart failure (CHF) and valvular disease (VD). Composite relative risk reduction (cRRR) of late cardiotoxicity, between VMAT and IMPT, were calculated as the weighted mean of relative risk reduction for IHD, CHF and VD, calculated across a wide range of cardiovascular risk factor combinations. The proportion of mediastinal HL patients who could benefit from IMPT was estimated in European countries, based on the country population and on the number of active gantries, to propose country-specific cRRR thresholds for patient selection.
Results
Compared with VMAT, IMPT significantly reduced average mean doses to the heart (2.36 Gy vs 0.99 Gy, p < 0.01), to the left ventricle (0.67 Gy vs 0.03, p < 0.01) and to the valves (1.29 Gy vs. 0.06, p < 0.01). For a HL patient without cardiovascular risk factor other than anthracycline-based chemotherapy, the relative risks of late cardiovascular complications were significantly lower after IMPT compared with VMAT for ischemic heart disease (1.07 vs 1.17, p < 0.01), for congestive heart failure (2.84 vs. 3.00, p < 0.01), and for valvular disease (1.01 vs. 1.06, p < 0.01). The median cRRR of cardiovascular adverse events with IMPT was 4.8%, ranging between 0.1% and 30.5%, depending on the extent of radiation fields and on the considered cardiovascular risk factors. The estimated proportion of HL patients currently treatable with IMPT in European countries with proton therapy facilities ranged between 8.0% and 100% depending on the country, corresponding to cRRR thresholds ranging from 24.0% to 0.0%.
Conclusion
While a statistically significant clinical benefit is theoretically expected for ischemic heart disease, cardiac heart failure and valvular disease for mediastinal HL patients with IMPT, the overall cardiotoxicity risk reduction is notable only for a minority of patients. In the context of limited IMPT availability, this study proposed a general model-based selection approach for mediastinal HL patient based on calculated cardiotoxicity reduction, taking into consideration patient clinical characteristics and IMPT facility availability.
Introduction
Hodgkin lymphoma is a rare hematological malignancy, which incidence is estimated around 2.7 cases per 100,000 person-year [Citation1], with a high curability rate, estimated between 80% and 90% [Citation2]. Consolidative radiation therapy (RT) improves disease control for limited and intermediate HL and is currently a standard of care in those indications [Citation3]. However, first generation techniques used extended-field radiation therapy technique (EFRT), which increased late cardiac morbidity for mediastinal HL [Citation4]; consequently, significant progresses were made to improve organ-at-risk (OAR) sparing in order to reduce late radiation-induced toxicity. Recent technical breakthroughs notably include ‘butterfly’ volumetric modulated arctherapy (VMAT) [Citation5], deep-inspiration breath hold (DIBH), and intensity modulated proton therapy (IMPT) [Citation6]. Proton beams deliver most of their energy toward the end of their ranges, limiting distant-to-target dose deposition in OARs compared with standard photon RT techniques, leading to a significant dosimetric benefit at the cardiac level for mediastinal HL patients, even compared to VMAT [Citation7,Citation8]. However, while IMPT tolerance is excellent and its short-term toxicity profile might favorably compare with VMAT, its implementation in clinical practice is still challenging [Citation9,Citation10]. The current limited access to proton therapy facilities have led multiple countries to adopt various patient selection strategies for various cancer types, for which IMPT is not yet considered as a current standard [Citation9,Citation11–13]. While model-based selection strategies have already been implemented for head and neck and breast cancers [Citation14], no such strategy has been specifically proposed for HL yet. The purpose of this study was to estimate the benefit of IMPT for HL proton therapy for diverse cardiac adverse events and to propose a general frame for mediastinal HL patient selection strategy for IMPT based on cardiotoxicity reduction, patient clinical factors, and IMPT treatment availability.
Material and methods
Patient treatment and replanning
This retrospective dosimetric study included 30 mediastinal intermediate-stage patients treated at Institut Curie (Paris, France) between January 2018 and May 2020, by random sampling of the institutional database (which approximately corresponded to half of the mediastinal HL patients treated on this period). Median age was 30 years and there were 17 female patients (56.7%). No patient had significant baseline cardiac disease. ECOG performance status ranged between 0 and 2. All patients had intermediate stage disease, according to the EORTC staging system and all of them had mediastinal involvement: 22 patients (73.3%) had lower mediastinal involvement (defined as below the origin of the left main stem coronary artery) and the eight other patients (26.7%) had an upper mediastinal disease. Eight patients (26.7%) had a mediastinal bulky presentation. In addition, fifteen patients (50.0%) presented with neck involvement and two patients (6.7%) with axillary involvement. Twenty-seven patients (90%) received upfront ABVD regimen, while BEACOPP regimen was delivered to the three other patients (10%). Patients were immobilized supine; simulation non-contrast computed tomography (CT) scans were acquired with spirometer-controlled deep-inspiration breath hold (DIBH), using 2 mm slices, and images were transferred to the Raystation v.10B treatment planning system for VMAT planning. Clinical target volumes (CTV) were defined according to the involved-site radiation therapy (ISRT) standard. Planned target volumes (PTV) were defined with a 5 mm expansion margin around clinical target volumes (CTV). Patients were treated to the total dose of 30 Gy in 15 fractions according to ESMO guidelines. A minimum of 95% of the prescribed dose was planned to be delivered to 95% of the PTV and a maximum of 107% of the prescribed dose could be delivered to 2% of the PTV. Dose constraints to organs-at-risk respected ILROG guidelines [Citation15], including mean heart dose ≤ 5 Gy, mean dose to the breasts ≤ 4 Gy, lung V5Gy ≤ 55%, lung V20Gy ≤ 30%, mean lung dose ≤ 10 Gy and thyroid V25Gy ≤ 62.5%. DIBH was controlled with spirometer.
Intensity modulated proton therapy (IMPT) with quasi-discrete active scanning was retrospectively re-planned at the National Center for Oncological Hadrontherapy (CNAO, Pavia, Italy) on the initial simulation CT scans aiming to achieve a similar CTV coverage. Robust optimization with multifield optimization was used for IMPT plan calculations on CTV; 2 mm isotropic setup error and 3% range uncertainty were applied. Cardiac motion was not specifically taken into account. Spot spacing was 3 mm with an energy step of 2 mm in water. One to four pencil beam scanning (PBS) fields were planned, depending on the target localization and on the patient anatomy. For complex target volumes, targets could be divided for multifield planning based on the anatomical localization (upper mediastinum, lower mediastinum, neck, axillary, etc.); in cases of diffuse mediastinal targets, a combination of anterior and posterior fields could be notably used to optimize cardiac and lung sparing. No systematic class solution was used for beam arrangement, which was defined on a case-by-case basis. Use of range shifter depended on the target shape and localization. Proton plans were made by physicists experienced in proton therapy planning for HL. This study was reviewed and approved by the institutional review board of the Institut Curie (Paris, France) and conducted in accordance with the STROBE guidelines [Citation16] (Supplementary Material).
Cardiotoxicity modeling
Modeled cardiac adverse events were ischemic heart disease (IHD), congestive heart failure (CHF) and valvular disease (VD). Cardiotoxicity models estimating the relative risk of each of these three radiation-induced cardiac adverse events (RRRadiotherapy) were selected, calculated based on dosimetric parameters to cardiac substructures and specifically developed on the 1965–1995 Netherland HL lymphoma survivor cohort to ensure model homogeneity [Citation17–19] (). The heart, the left ventricle and the aortic, pulmonary, mitral, and tricuspid valves were delineated according to published contouring guidelines to ensure homogeneity and all contours were independently reviewed [Citation20–22]. Mean doses to the heart, to the left ventricle and average mean doses to the cardiac valves were retrieved from dose-volume histograms and relative risks of specific late radiation-induced cardiac toxicities were calculated as follow:
Table 1. Cardiotoxicity models for ischemic heart disease, congestive heart failure and valvular disease.
The analysis of the 1965–1995 Netherlands HL lymphoma survivor cohort demonstrated that the relative risk of late cardiac complications related to patient clinical characteristics (RRClinical) was modulated by standard cardiovascular risk factors (hypertension, hypercholesterolemia, diabetes), family history of cardiovascular events, obesity, use of anthracycline-based chemotherapy and splenectomy [Citation17–19] (). Consequently, for a same RT plan, the predominantly increased cardiotoxicity risk between IHD, CHF or VD may ultimately change based on clinical considerations. Since no interaction between patient clinical characteristics and radiation-induced toxicity increase was found, the overall relative risk (RR) of a given patient for a specific cardiotoxicity type can be calculated as:
The RR of a given cardiotoxicity type after VMAT (and IMPT (
can be calculated and compared, knowing patient clinical characteristics and radiation dose delivered to cardiac substructures, and relative risk reductions (RRR) for IHD, CHF and VD with IMPT (compared to VMAT) were calculated as:
Since the amplitude of the clinical benefit of proton therapy at the cardiac level may depend on the a priori predominant cardiotoxicity type, a composite relative risk reduction (cRRR), combining the relative risk reduction for IHD, CHF and VD, was calculated, as the weighted mean of the three related RRR using the corresponding a priori baseline as the ponderation factor.
In order to evaluate the benefit of IMPT across a wide range of patient clinical characteristics, for each of the 30 VMAT and corresponding IMPT plans, cRRR were calculated for the 32 possible combination of the 5 considered clinical characteristics (cardiovascular risk factor, family history of cardiovascular events, obesity, anthracycline-based chemotherapy regimen and splenectomy [Citation17–19]). For each of the 30 plans, the minimum, median and maximum cRRR were retrieved. The complementary cumulative distribution function of the median cRRR values for the 30 treatment plans, considered representative of the general mediastinal HL population, was generated:
Treatment capacity and threshold calculation
The incidence of HL is estimated at 2.7 cases per 100,000 person-year, the proportion of stage I–II HL lymphoma represents around half of all cases [Citation23,Citation24] and approximately two thirds of all HL patients had a thoracic involvement [Citation25]. Consequently, the incidence of early-stage mediastinal HL that should benefit from consolidative RT is around 1 case per 100,000 person-years. The treatment capacity of proton therapy center for HL patients may be limited by the already active file of patients treated for cancers for which proton therapy is already a standard of care (such as choroid melanoma, pediatric tumors or chordomas). Considering that an already commissioned gantry can treat α additional HL patients per year, the theoretical proportion of mediastinal limited-stage HL who could beneficiate from IMPT in a country of β million inhabitants with γ gantries available would be
The cRRR threshold for selecting mediastinal early-stage HL for IMPT, allowing all patients above this threshold to benefit from proton therapy in this country, would be the value Δ(α,β,γ) defined by
The parameters α, β and γ might change with demographic changes and commissioning of new IMPT facilities and are thus functions of time; these parameters should continuously be reevaluated, leading to a variable proportion of treatable HL patients with IMPT at the national level and a time-variable cRRR threshold Δ(t). For the simulation purpose in this study, the current α value was set at 25 additional HL patients per year, based on the clinical experience of the single-gantry Proton Therapy Center of the Institut Curie [Citation12], the β values for European countries were extracted from 2021 national census, and the γ values for European countries with IMPT facilities were extrapolated from 2021 PTCOG data on the number of active gantries [Citation26]. For convenience, cRRR thresholds were rounded to the nearest half.
Results
Doses to the heart, to the left ventricle and to the valves were significantly lower with IMPT, compared with VMAT (). Average mean heart dose (MHD) was 2.36 Gy (range 0.13–14.38) with VMAT and 0.99 Gy (range 0.01–5.88) with IMPT (p < 0.01); average mean dose to the left ventricle was 0.67 Gy (range 0–12.43) with VMAT and 0.03 Gy (range 0–6.10) with IMPT (p < 0.01); average mean dose to the aortic valve was 1.29 Gy (range 0–22.37) with VMAT and 0.06 Gy (range 0–9.88 Gy) with IMPT (p < 0.01); average mean dose to the mitral valve was 0.93 Gy (range 0–25.66) with VMAT and 0.02 Gy (range 0–13.31 Gy) with IMPT (p < 0.01); average mean dose to the pulmonary valve was 5.68 Gy (range 0.16–24.97) with VMAT and 1.08 Gy (range 0.04–16.09 Gy) with IMPT (p < 0.01); average mean dose to the tricuspid valve was 0.67 Gy (range 0–15.94) with VMAT and 0.04 Gy (range 0–1.42 Gy) with IMPT (p < 0.01); average global mean dose to the valves was 2.35 Gy (range 0.04–22.24) with VMAT and 0.3 Gy (range 0.01–9.75 Gy) with IMPT (p < 0.01).
Figure 1. Mean dose to the heart, to the left ventricle and to the valves with photon radiotherapy (VMAT) and proton therapy (IMPT).
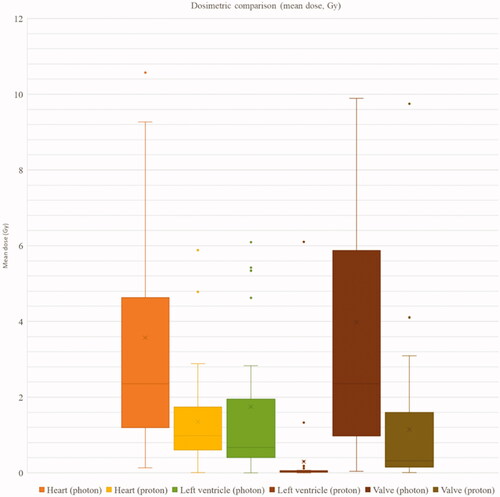
For a HL patient without cardiovascular risk factor other than anthracycline-based induction chemotherapy, the individual RR of cardiovascular complications were significantly lower with IMPT compared with VMAT for IHD (median RR: 1.07 [1.00–1.44] with IMPT vs. 1.17 [1.01–2.06] with VMAT, p < 0.01), for CHF (median RR: 2.84 [2.83–4.38] with IMPT vs. 3.00 [2.83–6.00] with VMAT, p < 0.01), and for VD (median RR: 1.01 [1.00–1.24] with IMPT vs. 1.06 [1.00–1.56] with VMAT, p < 0.01). Similarly, the RR reduction of cardiotoxicity with IMPT compared with VMAT was consistently observed in in all the possible combinations of considered clinical risk factors (cardiovascular risk factor, familial history, anthracycline use, increased BMI, splenectomy) (Supplementary Table 1). When considering patients without cardiovascular risk factor other than anthracycline-based induction chemotherapy, the median composite relative risk reduction (cRRR) of cardiac complications was 5.0%, ranging between 0.5% and 29.7%, depending on the extent of radiation fields. Considering the 32 possible combinations of the five considered cardiovascular risk factors, median cRRR was 4.8%, ranging between 0.1% and 30.5%, depending on the extent of radiation fields. The complementary cumulative distribution function of the median cRRR for each of the 30 treatment plans, calculated across all possible combinations of risk factors, is provided in .
Figure 2. Complementary cumulative distribution function of the cumulative relative risk reduction (cRRR) on 30 patients. The minimum, median and maximum cRRR for all 30 patients, obtained by evaluating all combination of risk factors included in the toxicity models (cardiovascular risk factor, familial history, anthracycline use, increased BMI, splenectomy) are provided in red, yellow and green, respectively.
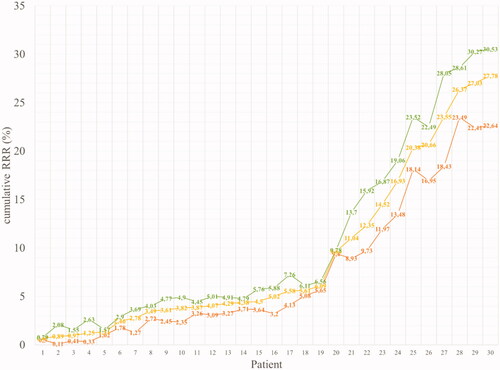
The estimation of the proportion of HL patients currently treatable with IMPT in each European country with proton therapy facilities, and the corresponding cRRR threshold, is provided in . To this date, this proportion ranged between 8.0% for Italy and 100% for Denmark, corresponding to cRRR threshold ranging from 24.0% for Italy to 0.0% for Denmark (where all mediastinal limited-stage HL patients could theoretically benefit from proton therapy) ().
Table 2. National treatment capacity of European countries with proton therapy facilities.
Discussion
In this dosimetric study, we proposed a general frame for selecting mediastinal HL patients based on cardiotoxicity modeling, taking into account patient clinical characteristics and IMPT facility availability. Due to the current IMPT resource limitation, multiple selection strategies have been proposed in order to optimally select patients for proton therapy treatment. Three main types of selection processes have been developed, all relying on a primary dosimetric comparison between an optimally-planed photon RT technique and IMPT [Citation9]. For mediastinal HL patients, ILROG guidelines should be respected and DIBH-VMAT should be theoretically preferred for such comparative dosimetric comparisons with IMPT [Citation15]. The first selection approach is limited to a direct dosimetric comparison between VMAT and IMPT, resulting in IMPT acceptance when the absolute dose difference to the OARs is above a predefined threshold. Such patient selection strategy is easy to implement in clinical practice [Citation12] but does not give any information on the magnitude of the clinical benefit. However, in the future, identification of patient populations for whom a dosimetric benefit for IMPT is constantly observed may potentially suppress the need for a systematic dosimetric comparison between VMAT and IMPT; this is for instance the case for disease located under the 7th vertebra for MHD [Citation13]. The second selection approach relies on cost-effectiveness analysis; in this case, IMPT is selected when its additional cost is lower than the economic gain related to the expected toxicity reduction between VMAT and IMPT. Based on MHD on VMAT plans, Vega et al. [Citation27] proposed age- and sex-dependent MHD thresholds for IMPT selection, which would be at least required to ensure cost-effectiveness of IMPT. The third patient selection approach relies on toxicity modeling and is currently approved in the Netherlands for IMPT reimbursement: the expected risk of late adverse events, calculated from dosimetric data, is compared between VMAT and IMPT plans. IMPT would then be selected if the expected toxicity difference between VMAT and IMPT is above a predefined threshold [Citation11]. In practice, the main difficulty of such model-based selection process is to objectively define a threshold and to decide which toxicities should be taken into account [Citation9]. Our proposed selection frame is based on cardiac toxicity modeling, additionally incorporates clinical characteristics to ponderate toxicity reduction and defines thresholds based the national treatment capacity, which is an objective data allowing for time-variable threshold variations depending on IMPT facility accessibility and demographic changes. Overall, we estimated that the relative risk reduction of cardiotoxicity with IMPT compared with VMAT was around 4.5% in our mediastinal HL population, which was randomly selected in order to ensure representativeness. This overall benefit is limited, suggesting that not all patients may objectively clinically benefit from IMPT; it should however be stressed that breast cancer proton therapy, which is being gradually implemented in clinical practice [Citation28–30], is accepted in the Netherlands for a threshold of 2% for major coronary events [Citation31] as defined by Darby et al. [Citation32].
We underlined the inequality between European countries in term of proportion of mediastinal HL patients who could be treated with IMPT. Without considering international patient transfer, selection criteria might be strongly dependent on logistical considerations; the problematic of patient selection does not technically exist in countries without proton therapy treatment centers and is limited to economic considerations in those that can theoretically treat all mediastinal HL patients with IMPT, which is only the case of Denmark in Europe to this date. In other countries with proton therapy facilities, the selection thresholds depend on the number of gantries, which is rapidly increasing, and on the population demographic [Citation31]; it is expected that the proportion of HL patients treated with IMPT will increase worldwide and that selection thresholds might become less stringent. International cooperation is possible through established networks, such as the European Particle Therapy Network [Citation33]. We proposed to identify cRRR selection thresholds for IMPT based on the proton therapy treatment capacity at the national level, which we considered to be an objective value; it should be stressed that this approach can be similarly extended at the supra-national level, in case of international collaboration. We found highly variable cRRR selection thresholds in the different European countries, which was a reflect of the inequal access to proton therapy treatment facilities around Europe. Defining different selection thresholds in the different European countries underlines the inequalities in access to care in oncology [Citation34], pleads for international cooperation but does not have directly a clinical justification. In practice, for each new mediastinal HL patient, we suggest that a cRRR score can be calculated, based on dosimetric parameters to the heart substructures on optimized VMAT and IMPT plans, and compared with the nationally-defined cRRR selection thresholds for IMPT selection. It should be noted that these thresholds do not necessarily correspond to a clinically meaningful risk reduction, since we calculated that some European countries (such as Denmark and the Netherlands) have already the capacity to treat nearly all their mediastinal HL patient population with IMPT, independently of the amplitude of the cardiac risk reduction. Since the proton therapy capacity is expected to increase in the near future, the national cRRR thresholds are expected to decrease with time and the proportion of HL patients who could be treated with IMPT will increase.
In line with previous dosimetric studies which demonstrated that IMPT reduced main cardiac substructure exposure (cardiac chambers and coronary arteries) [Citation7,Citation8], we additionally evidenced the dosimetric benefit of IMPT at the cardiac valve level. A dosimetric benefit does not systematically translates into a clinically relevant outcome but we found a significatively positive cardiotoxicity relative risk reduction, in favor of IMPT, due to the linearity between mean valve doses and radiation-related VD in the included toxicity model. However, this VD risk reduction was much more limited than CHD risk reduction. We aggregated in the combined cRRR scores different cardiac toxicities for which epidemiologic follow-up was sufficient enough to derive NTCP models from dosimetric data. As a consequence, only IHC, CHF and VD were considered in our model, given the existence of dose-response relationships. While arrythmia and conduction disorders are frequent radiation-induced cardiac adverse events after mediastinal irradiation [Citation35], no specific NTCP models have been developed for these cardiotoxicity types. Nevertheless, development of NTCP models for arrhytmias and conduction disorders after mediastinal HL radiotherapy could be considered, given the existence of conduction system delineation guidelines [Citation36,Citation37]. Lung toxicity and secondary cancers represent other radiation-induced complications of mediastinal HL irradiation and they could be additionally considered in a multi-organ model-based selection. Such multi-organ selection process would be more complete but also more complex. A combined multi-organ toxicity score, aggregating intrinsically different adverse events, may require ponderation of the different possible toxicities that could be challenging to objectively implement; in addition, what may be crucial for the treating radiation oncologist may not necessarily be the most important for a given patient.
It should be noted that the NTCP cardiotoxicity models that were aggregated in the present study relied on clinical data from patients treated with 3D radiotherapy at the end of the XXth century, which is subsequent to the late apparition of cardiac adverse events after radiotherapy. These cardiotoxicity models, developed during the 3D radiotherapy era, have not been prospectively validated with other techniques, in particular IMPT, and whether or not they are still valid for modern radiotherapy techniques has been subject to debate [Citation38]. It has been however demonstrated that toxicity models developed on photon RT techniques for head and neck cancers might be as well applied for IMPT, with acceptable performances [Citation39].
The MHD is currently the most widely used cardiac dosimetric parameter [Citation40,Citation41]; however the predicted cardiotoxicity benefit took into account cardiac substructure exposure between IMPT and VMAT. Clinical studies in lung cancers have suggested that doses to specific cardiac substructures correlated with specific cardiac toxicity [Citation42,Citation43]. While this might also be the case for HL, this is yet to be demonstrated and substructure dose constraint propositions are still limited [Citation44]. However, larger cardiotoxicity study might help precising substructure dose constraints. While manual delineation is time consuming, development of artificial intelligence tool will ease this work in the near future [Citation37,Citation45].
The issue of radiation-induced cardiotoxicity in HL management is also evolving with time, with an increasing reduction for radiotherapy place. While current ISRT or INRT paradigms consist in treating all initially involved sites, reduced radiation fields to residual sites were tested in the HD15 study of the German Hodgkin Study Group [Citation46]. Similarly, the Memorial Sloan Kettering Cancer Center suggested that treating HL patients with reduced fields (RFRT) directed to the areas of remaining masses that were PET-positive following chemotherapy might be sufficient, and this approach was evaluated in newly diagnosed HL patients with bulky disease after four cycles of brentuximab vedotin, doxorubicin, vinblastine, and dacarbazine [Citation47]. For interim and end-of-treatment PET-negative patients, 2-year PFS after 30 Gy ISRT, 20 Gy ISRT, 20 Gy RFRT or no further RT were similar (93%, 97%, 90% and 97%, respectively). In addition, the recent results of the HD17 trial [Citation48,p.17] suggested that PET negativity may identify a population of newly diagnosed HL patients who may not require consolidation RT.
In conclusion, while a statistically significant clinical benefit is theoretically expected for ischemic heart disease, cardiac heart failure and valvular disease for mediastinal HL patients with IMPT, the overall cardiotoxicity risk reduction is notable only in a minority of patients. In the context of limited IMPT availability, this study proposed a general model-based selection approach for mediastinal HL patient based on calculated cardiotoxicity reduction, taking into consideration patient clinical characteristics and IMPT facility availability.
Supplemental Material
Download MS Word (33 KB)Supplemental Material
Download MS Word (18.8 KB)Disclosure statement
No potential conflict of interest was reported by the author(s).
Data sharing statement
Research data are stored in an institutional repository and will be shared upon request to the corresponding author.
References
- Townsend W, Linch D. Hodgkin’s lymphoma in adults. Lancet. 2012;380(9844):836–847.
- Cuccaro A, Bartolomei F, Cupelli E, et al. Prognostic factors in Hodgkin lymphoma. Mediterr J Hematol Infect Dis. 2014;6(1):e2014053.
- André MPE, Girinsky T, Federico M, et al. Early positron emission tomography response-adapted treatment in stage I and II Hodgkin lymphoma: final results of the randomized EORTC/LYSA/FIL H10 trial. J Clin Oncol. 2017;35(16):1786–1794.
- Holtzman AL, Stahl JM, Zhu S, et al. Does the incidence of treatment-related toxicity plateau after radiation therapy: the long-term impact of integral dose in Hodgkin’s lymphoma survivors. Adv Radiat Oncol. 2019;4(4):699–705.
- Besson N, Pernin V, Zefkili S, et al. Evolution of radiation techniques in the treatment of mediastinal lymphoma: from 3D conformal radiotherapy (3DCRT) to intensity-modulated RT (IMRT) using helical tomotherapy (HT): a single-Centre experience and review of the literature. Br J Radiol. 2016;89(1059):20150409.
- Hoppe BS, Flampouri S, Zaiden R, et al. Involved-node proton therapy in combined modality therapy for Hodgkin lymphoma: results of a phase 2 study. Int J Radiat Oncol Biol Phys. 2014;89(5):1053–1059.
- Hoppe BS, Flampouri S, Su Z, et al. Effective dose reduction to cardiac structures using protons compared with 3DCRT and IMRT in mediastinal Hodgkin lymphoma. Int J Radiat Oncol Biol Phys. 2012;84(2):449–455.
- Scorsetti M, Cozzi L, Navarria P, et al. Intensity modulated proton therapy compared to volumetric modulated arc therapy in the irradiation of young female patients with hodgkin’s lymphoma. Assessment of risk of toxicity and secondary cancer induction. Radiat Oncol. 2020;15(1):12.
- Loap P, De Marzi L, Mirandola A, et al. Development and implementation of proton therapy for hodgkin lymphoma: challenges and perspectives. Cancers (Basel). 2021;13(15):3744.
- Loap P, Mirandola A, De Marzi L, et al. Current situation of proton therapy for Hodgkin lymphoma: from expectations to evidence. Cancers (Basel). 2021;13(15):3746.
- Langendijk JA, Lambin P, De Ruysscher D, et al. Selection of patients for radiotherapy with protons aiming at reduction of side effects: the model-based approach. Radiother Oncol. 2013;107(3):267–273.
- Loap P, Goudjil F, Dendale R, et al. Clinical and technical considerations for mediastinal Hodgkin lymphoma protontherapy based on a single-center early experience. Cancer Radiother. 2021;25:779–785.
- Ntentas G, Dedeckova K, Andrlik M, et al. Clinical intensity modulated proton therapy for Hodgkin lymphoma: which patients benefit the most? Pract Radiat Oncol. 2019;9(3):179–187.
- Tambas M, Steenbakkers RJHM, van der Laan HP, et al. First experience with model-based selection of head and neck cancer patients for proton therapy. Radiother Oncol. 2020;151:206–213.
- Specht L, Yahalom J, Illidge T, et al. Modern radiation therapy for Hodgkin lymphoma: field and dose guidelines from the international lymphoma radiation oncology group (ILROG). Int J Radiat Oncol Biol Phys. 2014;89(4):854–862.
- Cuschieri S. The STROBE guidelines. Saudi J Anaesth. 2019;13(Suppl 1):S31–S34.
- van Nimwegen FA, Schaapveld M, Cutter DJ, et al. Radiation dose-response relationship for risk of coronary heart disease in survivors of Hodgkin lymphoma. J Clin Oncol. 2016;34(3):235–243.
- Cutter DJ, Schaapveld M, Darby SC, et al. Risk of valvular heart disease after treatment for Hodgkin lymphoma. J Natl Cancer Inst. 2015;107:djv008.
- van Nimwegen FA, Ntentas G, Darby SC, et al. Risk of heart failure in survivors of Hodgkin lymphoma: effects of cardiac exposure to radiation and anthracyclines. Blood. 2017;129(16):2257–2265.
- Duane F, Aznar MC, Bartlett F, et al. A cardiac contouring atlas for radiotherapy. Radiother Oncol. 2017;122(3):416–422.
- Socha J, Rygielska A, Uziębło-Życzkowska B, et al. PO-1177 a heart valves contouring atlas on average intensity projection 4D-CT for lung cancer radiotherapy. Radiother Oncol. 2021;161:S975–S977.
- Feng M, Moran JM, Koelling T, et al. Development and validation of a heart atlas to study cardiac exposure to radiation following treatment for breast cancer. Int J Radiat Oncol Biol Phys. 2011;79(1):10–18.
- Hodgkin lymphoma | Cancer Research UK [Internet]. [cited 2021 Jun 18]. Available from: https://www.cancerresearchuk.org/about-cancer/hodgkin-lymphoma.
- Bröckelmann PJ, Sasse S, Engert A. Balancing risk and benefit in early-stage classical Hodgkin lymphoma. Blood. 2018;131(15):1666–1678.
- Filly R, Bland N, Castellino RA. Radiographic distribution of intrathoracic disease in previously untreated patients with Hodgkin’s disease and non-Hodgkin’s lymphoma. Radiology. 1976;120(2):277–281.
- PTCOG - facilities in operation [Internet]. [cited 2021 Oct 28]. Available from: https://www.ptcog.ch/index.php/facilities-in-operation.
- Vega RBM, Mohammadi H, Patel SH, et al. Establishing cost-effective allocation of proton therapy for patients with mediastinal Hodgkin lymphoma. Int J Radiat Oncol Biol Phys. 2022;112(1):158–166.
- Loap P, Beddok A, Cao KI, et al. Clinical practice of breast cancer protontherapy: a single-Centre experience from selection to treatment. Cancer Radiother. 2021;25:358–365.
- Loap P, Tkatchenko N, Goudjil F, et al. Cardiac substructure exposure in breast radiotherapy: a comparison between intensity modulated proton therapy and volumetric modulated arc therapy. Acta Oncol. 2021;60:1038–1044.
- Loap P, De Marzi L, Almeida CE, et al. Hadrontherapy techniques for breast cancer. Crit Rev Oncol Hematol. 2022;169:103574.
- Stick LB, Lorenzen EL, Yates ES, et al. Selection criteria for early breast cancer patients in the DBCG proton trial - the randomised phase III trial strategy. Clin Transl Radiat Oncol. 2021;27:126–131.
- Darby SC, Ewertz M, McGale P, et al. Risk of ischemic heart disease in women after radiotherapy for breast cancer. N Engl J Med. 2013;368(11):987–998.
- Weber DC, Langendijk JA, Grau C, et al. Proton therapy and the European Particle Therapy Network: the past, present and future. Cancer Radiother. 2020;24(6-7):687–690.
- Barcellini A, Mas FD, Paoloni P, et al. Please mind the gap—about equity and access to care in oncology. ESMO Open. 2021;6(6):100335. Internet [cited 2022 May 23]. Available from: https://www.esmoopen.com/article/S2059-7029(21)00297-0/fulltext.
- Jaworski C, Mariani JA, Wheeler G, et al. Cardiac complications of thoracic irradiation. J Am Coll Cardiol. 2013;61(23):2319–2328.
- Loap P, Servois V, Dhonneur G, et al. A radiotherapy contouring atlas for cardiac conduction node delineation. Pract Radiat Oncol. 2021;11:e434–e437.
- Loap P, Marzi LD, Kirov K, et al. Development of simplified auto-segmentable functional cardiac atlas. Practic Radiat Oncol. 2022;S1879-8500(22)00055-8. DOI:10.1016/j.prro.2022.02.004
- Schneider U, Ernst M, Hartmann M. The dose-response relationship for cardiovascular disease is not necessarily linear. Radiat Oncol. 2017;12(1):74.
- Blanchard P, Wong AJ, Gunn GB, et al. Toward a model-based patient selection strategy for proton therapy: external validation of photon-derived normal tissue complication probability models in a head and neck proton therapy cohort. Radiother Oncol. 2016;121(3):381–386.
- Hoppe BS, Bates JE, Mendenhall NP, et al. The meaningless meaning of mean heart dose in mediastinal lymphoma in the modern radiation therapy era. Pract Radiat Oncol. 2020;10:e147–e154.
- Loap P, Kirova Y. Evaluating cardiac substructure radiation exposure in breast rotational intensity modulated radiation therapy: effects of cancer laterality, fractionation and deep inspiration breath-hold. Cancer Radiother. 2021;25(1):13–20.
- Chen VE, Song AJ, Werner-Wasik M, et al. Effect of radiation dose to cardiac substructures on the acute development of new arrhythmias following conventionally fractionated radiation treatment to the lung. Int J Radiat Oncol Biol Phys. 2019;105(1):E500.
- Atkins KM, Chaunzwa TL, Lamba N, et al. Association of left anterior descending coronary artery radiation dose with major adverse cardiac events and mortality in patients with non-small cell lung cancer. JAMA Oncol. 2021;7(2):206–219.
- Dabaja BS, Hoppe BS, Plastaras JP, et al. Proton therapy for adults with mediastinal lymphomas: the International Lymphoma Radiation Oncology Group guidelines. Blood. 2018;132(16):1635–1646.
- Loap P, Tkatchenko N, Kirova Y. Evaluation of a delineation software for cardiac atlas-based autosegmentation: an example of the use of artificial intelligence in modern radiotherapy. Cancer Radiother. 2020;24(8):826–833.
- Engert A, Haverkamp H, Kobe C, et al. Reduced-intensity chemotherapy and PET-guided radiotherapy in patients with advanced stage Hodgkin’s lymphoma (HD15 trial): a randomised, open-label, phase 3 non-inferiority trial. Lancet. 2012;379(9828):1791–1799.
- Kumar A, Casulo C, Advani RH, et al. Brentuximab vedotin combined with chemotherapy in patients with newly diagnosed early-stage, unfavorable-risk Hodgkin lymphoma. J Clin Oncol. 2021;39(20):2257–2265.
- Borchmann P, Plütschow A, Kobe C, et al. PET-guided omission of radiotherapy in early-stage unfavourable Hodgkin lymphoma (GHSG HD17): a multicentre, open-label, randomised, phase 3 trial. Lancet Oncol. 2021;22(2):223–234.