Abstract
Introduction
Right-lateralized cardiac substructures can be substantially exposed during right breast cancer (R-BC) radiotherapy. The cardiac benefit of deep inspiration breath hold (DIBH) is established in combination with volumetric modulated arctherapy (VMAT) for left breast cancer with regional node irradiation but is unknown for R-BC. This study evaluated the dosimetric benefit of DIBH for locoregional irradiation of R-BC with VMAT.
Material and Methods
All patients treated for R-BC with adjuvant locoregional DIBH-VMAT in the Department of Radiation Oncology of the Institut Curie (Paris, France) until December 2022 were included, corresponding to 15 patients. FB- and DIBH-VMAT plans were compared both for a normofractionated regimen (50 Gy/25fx) used for treatment and a replanned hypofractionated regimen (40 Gy/15fx). Dose to the heart, cardiac substructures (sinoatrial node (SAN), atrio-ventricular node (AVN), right coronary artery, left anterior descending coronary artery, left ventricle), ipsilateral lung and liver were retrieved and compared.
Results
Mean heart dose (MHD) was 3.33 Gy with FB vs. 3.10 Gy with DIBH on normofractionated plans (p = 0.489), and 2.58 Gy with FB vs. 2.41 Gy with DIBH on hypofractionated plan (p = 0.489). The benefit of DIBH was not significant for any cardiac substructure. The most exposed cardiac substructure were the SAN (mean dose of 6.62 Gy for FB- and 5.64 Gy for DIBH-VMAT on normofractionated plans) and the RCA (mean dose of 4.21 Gy for FB- and 4.06 Gy for DIBH-VMAT on normofractionated plans). The maximum benefit was observed for the RCA with a median individual dose reduction of 0.84 Gy on normofractionated plans (p = 0.599). No significant dosimetric difference were observed for right lung. Liver mean dose was significantly lower with DIBH with median values decreasing from 2.54 Gy to 0.87 Gy (p = 0.01).
Conclusion
Adding DIBH to efficient cardiac-sparing radiotherapy techniques, such as VMAT, is not justified in the general case for locoregional R-BC irradiation. Specific R-BC patient subpopulations who could benefit from additional DIBH combination with locoregional VMAT are yet to be identified.
Keywords:
Introduction
Right-sided breast cancer (R-BC) radiotherapy is usually associated with lower mean heart dose (MHD) compared with left-sided breast cancer (R-BC) irradiation [Citation1], since the heart is left-lateralized in the mediastinum. Additionally, most epidemiological studies analyzing the dosimetric determinants of radiation-induced cardiotoxicity in breast cancer management focused on left-lateralized cardiac substructures [Citation2], such as the left ventricle (LV) [Citation3–7] or the left anterior descending coronary artery (LADCA) [Citation8,Citation9]; right-lateralized cardiac substructures, such as the sinoatrial node (SAN) or the right coronary artery (RCA) were usually disregarded until recently [Citation10,Citation11]. However, dosimetric parameters to the SAN or the RCA correlate with arrhythmia and mortality after lung cancer irradiation [Citation12]. Furthermore, when the internal mammary chain (IMC) is irradiated, R-BC radiotherapy is associated with high doses to right-lateralized cardiac substructures with mean doses to the proximal RCA segment sometimes reaching 10 Gy [Citation11], which is greater than what is usually recommended for the LADCA mean dose [Citation2,Citation8]. Consequently, the cardiotoxicity risk of locoregional R-BC irradiation should not be minimized a priori.
Technical progresses have been made to improve cardiac sparing for breast locoregional irradiation. Such advances include highly conformal techniques such as volumetric modulated arctherapy (VMAT) or helical tomotherapy [Citation11], deep-inspiration breath-hold (DIBH) [Citation13], and intensity modulated proton therapy (IMPT) [Citation14–16]. The cardiac dosimetric benefit of DIBH for left breast cancer (L-BC) locoregional irradiation with VMAT has been demonstrated [Citation11,Citation17,Citation18]. More recently, a dosimetric study focusing on R-BC locoregional radiotherapy with a classic 3D technique suggested that DIBH could possibly provide an additional cardiac dosimetric benefit, in addition to lung and liver dose reduction [Citation19]. However, when using modern highly-conformal cardiac-sparing techniques for R-BC such as VMAT, the additional benefit of DIBH is uncertain. In the department of Radiation Oncology (Institut Curie, Paris, France), R-BC locoregional irradiation with DIBH-VMAT was proposed in some specific situations; this study aimed to evaluate the dosimetric benefit of DIBH for locoregional irradiation of R-BC with VMAT in those patients.
Material and methods
Population
This study was conducted in the Department of Radiation Oncology of the Institut Curie (Paris, France) and approved by the local institutional review board. All consecutive patients treated for locally-advanced R-BC with adjuvant locoregional DIBH-VMAT until December 2022 were included. For all these patients, DIBH-VMAT was recommended by a multidisciplinary staff based on anatomical, clinical and dosimetric considerations (such as pectus excavatum, cardiac history or disease, high exposure to cardiotoxic antineoplastic drugs, unacceptable doses to organs-at-risk (OAR) with 3D techniques) [Citation20].
VMAT planning and treatment
All patients were immobilized supine, both arms above the head. Simulation non-contrast free-breathing (FB) and DIBH- computed tomography (CT) scans were acquired with 3 mm-thick slices. DIBH was controlled with an in-house camera system evaluating the inspiration amplitude based on skin marker displacement. FB-CT scan were routinely acquired when planning DIBH treatments to serve as back-up simulation CT scans in cases where the patients had to switch from DIBH to FB during irradiation, due to tolerance issues. DIBH-CT scan images were transferred to the Eclipse 15.6 treatment planning system (Varian Medical Systems) for VMAT planning. Clinical target volumes (CTV) were the whole right breast (after breast conserving surgery) or to the right chest wall (after total mastectomy) and the right regional lymph nodes (axillary level II-III nodes, supraclavicular nodes, inter-pectoral nodes, and IMC). Axillary level I nodes could be included in cases of positive sentinel node without of surgical dissection or of massive involvement (more than 50% of pathologically involved nodes). Inverse optimization was used to plan DIBH-VMAT treatment with four 230° arcs (beam angle ranging between 40° and 180°), using 6 MV photons, a maximum dose rate of 600 MU/min and 2° control point spacing. Patients were treated with a normofractionated regimen delivering 50 Gy to the CTV in 25 fractions. Planned target volumes (PTV) were defined with a 5-mm margin around CTV. A minimum of 95% of the PTV should receive at least 95% of the prescribed dose, and no more than 2% of the PTV could receive more than 107% of the prescribed dose. The heart, the left breast, the lungs and the spinal cord were considered as OAR. Generic institutional planning dose constraints were used for treatment planning optimization, with MHD < 5 Gy, lung V20Gy < 25% and V30Gy < 18%, left breast mean dose < 4 Gy, and spinal cord maximum dose < 34 Gy; no optimization was done on specific cardiac substructures. Rapidplan knowledge-based planning model (Varian Medical Systems) was used to optimize the treatment plan in order to minimize OAR exposure based on the patient’s anatomy [Citation21]. Treatments were delivered on Varian Truebeam linear accelerators with 120‐leaf multileaf collimators, with weekly offline control cone-beam CT.
As part of this study, in addition to the treatment normofractionated DIBH-VMAT plan, we additionally simulated for all patients a normofractionated FB-VMAT plan, a hypofractionated DIBH-VMAT plan, and a hypofractionated FB-VMAT plan. The PTV coverage objective and OAR dose constraints were the same and the simulated hypofractionated regimen was 40.05 Gy in 15 fractions.
Cardiac substructure delineation
The left ventricle (LV), the left anterior descending coronary artery (LADCA), the right coronary artery (RCA), the sinoatrial node (SAN) and the atrio-ventricular node (AVN) were retrospectively delineated on the simulation FB- and DIBH-CT scans according to published guidelines to ensure reproducibility and homogeneity [Citation22,Citation23]; an illustrative case is provided in the Supplementary Figure 1. These cardiac substructures were selected based on the existence of established dose-toxicity relationships for breast or thoracic irradiation (LADCA, LV, SAN) [Citation2,Citation8,Citation12] or on their right-laterality (RCA, SAN, AVN). Substructure contours were evaluated by two radiation oncologists and one radiologist to ensure delineation exactness.
Statistics
Mean dose to the heart, to the LV, to the LADCA, to the RCA, to the SAN and to the AVN, V10Gy to the LADCA and the RCA, as well as dosimetric parameters to the liver and to the right lung (mean dose, V10Gy, V20Gy and V30Gy, volumes), were retrieved from the dose-volume histograms (DVH) of the FB- and DIBH-VMAT plans and compared with Wilcoxon signed-rank tests, both for the normofractionated and the hypofractionated regimens. In addition, comparison of the entire DVHs for the PTV and the organs-at-risk was performed with a p-curve obtained by applying Wilcoxon signed-rank tests for each dose level (see further description in Engstrom et al. [Citation24] and Bertelsen et al. [Citation25]).
Statistical significance was defined by a p-value < 0.05; for p-curve interpretation, large regions below 0.05 indicate dose distribution differences between DVHs. Pearson correlation coefficient matrixes were calculated between mean doses to the heart and to the other considered cardiac substructures. Statistical analyses were conducted using the R 4.0.1 software.
Results
Between January 2022 and October 2022, 15 R-BC patients were treated with locoregional normofractionated DIBH-VMAT. The median age was 51 years [range: 30–68]; 9 patients (60%) were treated after total mastectomy, and 6 patients (40%) after breast conserving surgery. Patients characteristics are provided in . All patients received full course treatment and well-tolerated the DIBH procedure. Average right lung volume increased from 1425 cc [range: 1110–2022] in FB to 2324 cc [1588–3046] with DIBH (p < 0.001), corresponding to a + 63.0% [range: 20.0–84.3%] relative volume change; average left lung volume increased from 1242 cc [range: 895–1650] in FB to 2017 cc [range 1337–2460] with DIBH (p < 0.001), corresponding to a + 74.0% [range: 32.3–113.8%] relative volume change.
Table 1. Clinical features of the right breast cancer patients treated with locoregional deep inspiration breath hold (DIBH) volumetric modulated arctherapy (VMAT).
DIBH slightly reduced mean dose to the heart and to all cardiac substructures, but this reduction was not significant in any case ( and , ). Median MHD was 3.33 Gy with FB and 3.10 Gy with DIBH on normofractionated plans (p = 0.489), and 2.58 Gy with FB and 2.41 Gy with DIBH on hypofractionated plans (p = 0.489). The most exposed cardiac substructure were the SAN (average mean dose of 6.62 Gy for normofractionated FB-VMAT, 5.64 Gy for normofractionated DIBH-VMAT, 5.24 Gy for hypofractionated FB-VMAT, 5.11 Gy for hypofractionated DIBH-VMAT) and the RCA (average mean dose of 4.21 Gy for normofractionated FB-VMAT, 4.06 Gy for normofractionated DIBH-VMAT, 3.14 Gy for hypofractionated FB-VMAT, 3.25 Gy for hypofractionated DIBH-VMAT). The maximum absolute benefit was observed for the RCA with a median individual dose reduction of 0.84 Gy on normofractionated plans (p = 0.599) and 0.62 Gy on hypofractionated plans (p = 0.762). Pearson correlation coefficients between mean dose to the heart and mean doses to cardiac substructures were usually weak and non-significant ().
Figure 1. (A) Mean doses to the heart, to cardiac substructures, to the lung and to the liver with volumetric modulated arctherapy (VMAT) with or without deep inspiration breath hold (DIBH). (B) Individual paired dosimetric parameters on free-breathing (FB) and DIBH VMAT plans. LV: left ventricle; LADCA: left anterior descending coronary artery; RCA: right coronary artery; SAN: sinoatrial node; AVN: atrio-ventricular node.
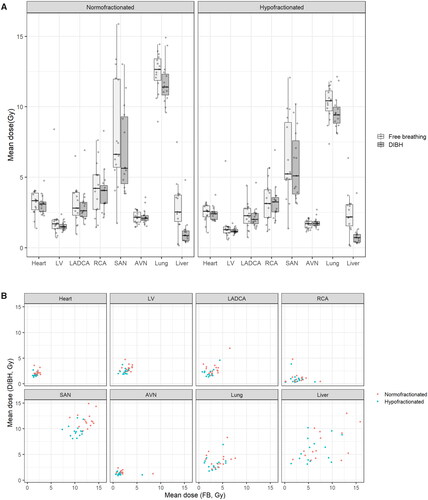
Figure 2. Mean dose differences between deep inspiration breath hold (DIBH) and free breathing (FB) volumetric modulated arctherapy plan for different organs-at-risk (heart, cardiac substructures, lung and liver).
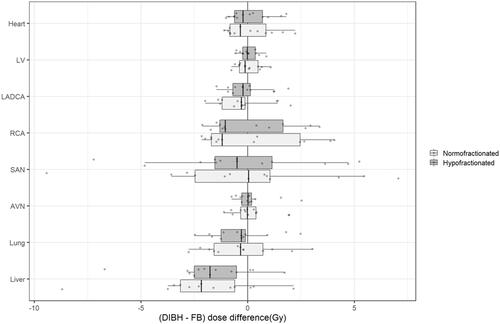
Figure 3. Correlation matrixes between mean doses to the heart and to cardiac substructures. Correlation strength (r value) is indicated by the color of the box (ranging between –1 and 1). A crossed box refers to a non-significant correlation. LV: left ventricle; LADCA: left anterior descending coronary artery; RCA: right coronary artery; SAN: sinoatrial node; AVN: atrio-ventricular node.
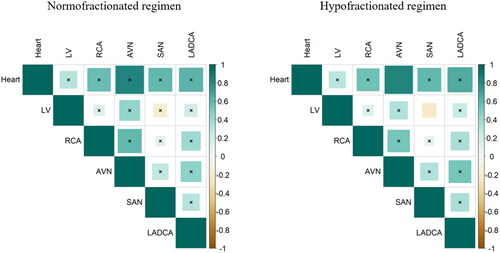
Table 2. Doses to the heart, to cardiac substructures, to the lung and to the liver with volumetric modulated arctherapy with or without deep inspiration breath hold (DIBH), for normofractionated and hypofractionated regimens.
No significant differences were observed in mean doses, V10Gy, V20 Gy and V30 Gy to the right lung between DIBH- and FB-VMAT plans. Liver mean dose was significantly lower with DIBH with median values decreasing from 2.54 Gy to 0.87 Gy for normofractionated regimens (p = 0.01) and from 2.18 Gy to 0.72 Gy for hypofractionated regimens (p = 0.01) ( and Citation2, ).
provides the mean DVHs for the PTV and for the OAR (cardiac substructures, lungs, contralateral breast, and liver) for the FB and DIBH hypofractionated plans. The dose distribution to the PTVs (right breast/chest wall, regional lymph nodes) were not statistically significant; for the OAR, only the liver DVH showed a statistical difference in the low dose region between DIBH and FB plans, while the dose distribution profiles to the other OAR were not statistically different.
Discussion
This study focusing on R-BC locoregional adjuvant irradiation with VMAT only found a dosimetric benefit of DIBH for the liver. DIBH did not provide any significant benefit for the ipsilateral lung, for the heart, or for any studied cardiac substructure. At the cardiac substructure level, the dosimetric gain was the greatest for the RCA, but the average individual observed benefit was less than 1 Gy and non-significant. The average benefit for left-sided substructures (the LV and the LADCA) were less than 0.4 Gy.
While the cardiac sub-entities supplied by the LADCA and RCA notably differ in terms of anatomy and of function, the histological and anatomopathological consequences of RCA overexposure might be somewhat similar to the ones of LADCA radiation-induced damages. It should be stressed that a LADCA mean dose threshold of 2.8 Gy (equivalent dose in 2 Gy fractions (EQD2)) was predictive of increased clinical cardiac adverse events [Citation8]. While this threshold was usually respected for the LADCA in our patient cohort, it was usually exceeded for the RCA with R-BC FB- and DIBH-VMAT. Potential complications related to RCA radiation exposure might include right ventricle congestive failure or dysfunction [Citation26], as well as right or inferior ischemic heart disease and conduction disorders due to blood supply considerations [Citation27]. Radiation overexposure of SAN was associated with atrial fibrillation development in lung cancer [Citation12]; however, for R-BC locoregional VMAT irradiation, the median individual dosimetric benefit of DIBH was lower than 0.4 Gy. Whether or not dose constraints for right-lateralized cardiac substructure will be similarly defined for R-BC radiotherapy is still an open debate. In any case, dose constraints to cardiac substructures have to take into account the fractionation regimen: to this date, cardiac substructure dose constraints have been proposed by analyzing the cardiac adverse events occurring after normofractionated breast radiotherapy [Citation2]. If ultra-hypofractionation became a standard of care for locoregional breast irradiation, cardiac substructure dose constraints will be necessarily substantially lower; correspondence tables have already been proposed [Citation28].
We found that the dosimetric benefit of DIBH at the cardiac substructure level was statistically non-significant for locoregional R-BC irradiation with VMAT; since normal tissue complication probability (NTCP) models rely on dosimetric data, the added clinical benefit of DIBH would likely be minimal in the general case for R-BC population with an indication for regional node irradiation. While small dosimetric benefits could be overcome by time-consuming procedures and by a waste of resources, there is no identified threshold for cardiac toxicity related to MHD [Citation29]: since the cardiotoxicity risk increases linearly with MHD [Citation29], even small dosimetric differences may translate into disparate clinical outcomes. Whether or not a R-BC subpopulation could clinically benefit from DIBH addition to VMAT for cardiotoxicity reduction is yet to be determined; such patients would typically have a substantially high baseline cardiac substructure exposure with FB-VMAT, resulting from anatomical particularities or from specific clinical situations (such as reirradiation, exclusive radiotherapy, or pathological IMC lymph node boost). In these challenging situations, however, breast proton therapy could be a more efficient cardiac-sparing technique than DIBH-VMAT [Citation14]. Follow-up of breast cancer patient cohorts treated with rotational intensity modulated radiation therapy (IMRT) (such as VMAT or helical tomotherapy) is still limited [Citation30]; establishing proper normal tissue complication probability (NTCP) models for VMAT will take time. Mathematical modeling suggests that NTCP cardiotoxicity models developed based on 3D breast radiotherapy may not valid when IMRT techniques are used [Citation31].
We found that DIBH had no significant cardiac or lung dosimetric benefit when combined with VMAT for R-BC locoregional irradiation, even when looking at the right-lateralized cardiac substructures. These results differ from those of a study evaluating DIBH for 3D radiotherapy [Citation19], where DIBH was associated with improved heart and lung sparing, and notably deviate from what was observed for L-BC irradiation where large scale dosimetric studies have demonstrated a major reduction in heart radiation exposure [Citation18,Citation32]. Plausible explanations could be that VMAT is already an efficient cardiac- and lung-sparing technique [Citation20] and that the heart is additionally less exposed in R-BC than in L-BC irradiation; consequently, any cardiac dosimetric benefit of DIBH for R-BC VMAT is necessarily minimal. Similarly, it has been shown that DIBH did not provide any substantial cardiac dosimetric benefit for breast proton therapy [Citation14]. In concordance with the previous R-BC 3D-radiotherapy study [Citation19], we similarly found a dosimetric benefit for the liver. However, whether or not this dosimetric benefit is clinically significant is highly debatable since radiation-induced liver toxicity with FB-VMAT in breast cancer patients seems extremely uncommon [Citation33]. Additionally, whether or not 3D radiotherapy with DIBH could be dosimetrically superior to FB VMAT was not investigated in this study, since locoregional irradiation are increasingly commonly conducted with rotational IMRT techniques [Citation20]. It should be underlined that the relative lung volume increase with DIBH in our study was comparable with results from other publications, similarly evidencing a 60–70% volume increase between FB and DIBH [Citation34], which suggests that the lack of dosimetric difference at the cardiac substructure level observed in our study may not be related to an inadequate breath-hold technique. On the other side, the PTV coverage between DIBH and FB plans were similar, ruling out the hypothesis that the benefits of increased heart-target distance could have gone into better target coverage. Possibly, VMAT treatment plans may not have been optimized with enough penalty on heart dose, which maximum mean dose was set at 5 Gy. It should be underlined that studies using ‘butterfly’ two-arc configuration techniques have achieved lower MHD for left-sided breast radiotherapy with regional node irradiation, ranging around 2 Gy in cases of internal mammary chain irradiation [Citation24] or even lower when the internal mammary chain was excluded from the PTV [Citation35]. Using four larger 230° arcs, with gantry angles between 40° and 180°, as it was planned in the present study, may have exposed a greater cardiac volume to low doses. Nevertheless, given our findings, it is unlikely that a significant DIBH dosimetric benefit for R-BC would have been observed by using a more cardiac-sparing VMAT planning technique.
MHD is widely considered as the main cardiac parameter, implicitly considering the heart as an homogeneous organ-at-risk. However, the heart is constituted of diverse tissues (such as myocardium, pericardium, nerves, vessels, amongst others) with a complex functional and histological organization; this complexity explains the diversity of radiation-induced cardiac adverse events. In addition, we found that MHD was poorly predictive of cardiac substructures exposure, in particular for the RCA and the SAN. This finding corroborates another dosimetric study that evidenced that MHD was not representative of cardiac substructure exposure for L-BC VMAT [Citation14]. These considerations plead for a more systematic consideration of cardiac substructures in breast radiotherapy with VMAT [Citation36], independently of the breast cancer laterality. However, systematic delineation of cardiac substructure in clinical practice is challenging, notably due to its limited reproducibility and its fastidiousness. Fortunately, multiple atlas-based and artificial-intelligence-based cardiac autosegmentation atlases have shown promising results and they may be routinely used in the near future [Citation37,Citation38].
We only included patients with locoregional irradiation indication, including the IMC. This population represents a small proportion of all breast cancer patients and this study is thus limited by the limited number of patients, which reduces its power. However, given the dose difference distributions for cardiac substructures, which systematically overlapped 0 Gy without any clear tendency for clinically-relevant dosimetric gain, it is unlikely that increasing the number of included patients may ultimately reveal a significant cardiac benefit for DIBH combined with VMAT.
Conclusion
The analysis of this cohort of right breast cancer patients treated with adjuvant locoregional VMAT demonstrated that additional DIBH did not improve ipsilateral lung or cardiac exposure, even for right-lateralized cardiac substructures. Liver exposure is significantly reduced when combining DIBH with VMAT but the clinical relevance of this dosimetric gain may be debatable.
Adding DIBH to efficient cardiac-sparing radiotherapy techniques such as VMAT, by fear of radiation-induced cardiotoxicity, is not justified in the general case for locoregional R-BC irradiation. However, specific R-BC patient subpopulations who could clinically benefit from additional DIBH combination with locoregional VMAT, due to anatomical or target volume considerations, might be better identified in the future.
Supplemental Material
Download MS Word (576.8 KB)Disclosure statement
No potential conflict of interest was reported by the author(s).
Data availability statement
Research data are stored in an institutional repository and will be shared upon request to the corresponding author
References
- Jacob S, Camilleri J, Derreumaux S, et al. Is mean heart dose a relevant surrogate parameter of left ventricle and coronary arteries exposure during breast cancer radiotherapy: a dosimetric evaluation based on individually-determined radiation dose (BACCARAT study). Radiat Oncol. 2019;14(1):29.
- Piroth MD, Baumann R, Budach W, et al. Heart toxicity from breast cancer radiotherapy : current findings, assessment, and prevention. Strahlenther Onkol. 2019;195(1):1–12.
- Erven K, Florian A, Slagmolen P, et al. Subclinical cardiotoxicity detected by strain rate imaging up to 14 months after breast radiation therapy. Int J Radiat Oncol Biol Phys. 2013;85(5):1172–1178.
- Marks LB, Yu X, Prosnitz RG, et al. The incidence and functional consequences of RT-associated cardiac perfusion defects. Int J Radiat Oncol Biol Phys. 2005;63(1):214–223.
- Skyttä T, Tuohinen S, Boman E, et al. Troponin T-release associates with cardiac radiation doses during adjuvant left-sided breast cancer radiotherapy. Radiat Oncol. 2015;10:141.
- D'Errico MP, Grimaldi L, Petruzzelli MF, et al. N-terminal pro-B-type natriuretic peptide plasma levels as a potential biomarker for cardiac damage after radiotherapy in patients with left-sided breast cancer. Int J Radiat Oncol Biol Phys. 2012;82(2):e239-246–e246.
- van den Bogaard VAB, Ta BDP, van der Schaaf A, et al. Validation and modification of a prediction model for acute cardiac events in patients with breast cancer treated with radiotherapy based on Three-Dimensional dose distributions to cardiac substructures. J Clin Oncol. 2017;35(11):1171–1178.
- Zureick AH, Grzywacz VP, Almahariq MF, et al. Dose to the left anterior descending artery correlates with cardiac events after irradiation for breast cancer. Int J Radiat Oncol Biol Phys. 2022;114(1):130–139.
- Nilsson G, Holmberg L, Garmo H, et al. Distribution of coronary artery stenosis after radiation for breast cancer. J Clin Oncol. 2012;30(4):380–386.
- Errahmani MY, Locquet M, Spoor D, et al. Association between cardiac radiation exposure and the risk of arrhythmia in breast cancer patients treated with radiotherapy: a Case-Control study. Front Oncol. 2022;12:892882.
- Loap P, Kirova Y. Evaluating cardiac substructure exposure in breast rotational intensity modulated radiation therapy: effects of cancer laterality, fractionation and deep inspiration breath-hold. Cancer Radiother. 2021;25(1):13–20.
- Kim KH, Oh J, Yang G, et al. Association of sinoatrial node radiation dose with atrial fibrillation and mortality in patients with lung cancer. JAMA Oncol. 2022;8(11):1624–1634.
- Gaál S, Kahán Z, Paczona V, et al. Deep-inspirational breath-hold (DIBH) technique in left-sided breast cancer: various aspects of clinical utility. Radiat Oncol. 2021;16(1):89.
- Loap P, Tkatchenko N, Goudjil F, et al. Cardiac substructure exposure in breast radiotherapy: a comparison between intensity modulated proton therapy and volumetric modulated arc therapy. Acta Oncol. 2021;60(8):1038–1044.
- Loap P, Beddok A, Cao KI, et al. Clinical practice of breast cancer protontherapy: a single-Centre experience from selection to treatment. Cancer Radiother. 2021;25(4):358–365.
- Loap P, De Marzi L, Almeida CE, et al. Hadrontherapy techniques for breast cancer. Crit Rev Oncol Hematol. 2022;169:103574.
- Wolf J, Stoller S, Lübke J, et al. Deep inspiration breath-hold radiation therapy in left-sided breast cancer patients: a single-institution retrospective dosimetric analysis of organs at risk doses. Strahlenther Onkol. 2022. DOI:10.1007/s00066-022-01998-z
- Nissen HD, Appelt AL. Improved heart, lung and target dose with deep inspiration breath hold in a large clinical series of breast cancer patients. Radiother Oncol. 2013;106(1):28–32.
- Peters GW, Gao SJ, Knowlton C, et al. Benefit of deep inspiratory breath hold for right breast cancer when regional lymph nodes are irradiated. Pract Radiat Oncol. 2022;12(1):e7–12–e12.
- Lauche O, Kirova YM, Fenoglietto P, et al. Helical tomotherapy and volumetric modulated arc therapy: new therapeutic arms in the breast cancer radiotherapy. World J Radiol. 2016;8(8):735–742.
- Costa E, Richir T, Robilliard M, et al. Assessment of a conventional volumetric-modulated arc therapy knowledge-based planning model applied to the new halcyon© O-ring linac in locoregional breast cancer radiotherapy. Phys Med. 2021;86:32–43.
- Loap P, Servois V, Dhonneur G, et al. A radiotherapy contouring atlas for cardiac conduction node delineation. Pract Radiat Oncol. 2021;11(4):e434–e437.
- Duane F, Aznar MC, Bartlett F, et al. A cardiac contouring atlas for radiotherapy. Radiother Oncol. 2017;122(3):416–422.
- Engstrøm KH, Brink C, Nielsen MH, et al. Automatic treatment planning of VMAT for left-sided breast cancer with lymph nodes. Acta Oncol. 2021;60(11):1425–1431.
- Bertelsen A, Hansen CR, Johansen J, et al. Single arc volumetric modulated arc therapy of head and neck cancer. Radiother Oncol. 2010 May;95(2):142–148.
- Tadic M, Cuspidi C, Hering D, et al. Radiotherapy-induced right ventricular remodelling: the missing piece of the puzzle. Arch Cardiovasc Dis. 2017;110(2):116–123.
- Futami C, Tanuma K, Tanuma Y, et al. The arterial blood supply of the conducting system in normal human hearts. Surg Radiol Anat. 2003;25(1):42–49.
- Loap P, Kirova Y. The challenge of cardiac dose constraint adaptation to hypofractionated breast radiotherapy in clinical practice. Strahlenther Onkol. 2021;197(6):555–557.
- Darby SC, Ewertz M, McGale P, et al. Risk of ischemic heart disease in women after radiotherapy for breast cancer. N Engl J Med. 2013;368(11):987–998.
- Zolcsak Z, Loap P, Fourquet A, et al. Long-term follow-up results of intensity-modulated radiotherapy with helicoïdal tomotherapy for non-metastatic breast cancers: single Centre experience. Cancer Radiother. 2022;26(5):654–662.
- Schneider U, Ernst M, Hartmann M. The dose-response relationship for cardiovascular disease is not necessarily linear. Radiat Oncol. 2017;12(1):74.
- Berg M, Lorenzen EL, Jensen I, et al. The potential benefits from respiratory gating for breast cancer patients regarding target coverage and dose to organs at risk when applying strict dose limits to the heart: results from the DBCG HYPO trial. Acta Oncol. 2018;57(1):113–119.
- Park HJ, Cheong KH, Koo T, et al. Effects of radiation dose on liver after free-breathing volumetric modulated arc therapy for breast cancer. In Vivo. 2022;36(4):1937–1943.
- Browne P, Beaton NR, Sharma H, et al. Identifying breast cancer patients who gain the most dosimetric benefit from deep inspiration breath hold radiotherapy. J Med Radiat Sci. 2020;67(4):294–301.
- Rice L, Goldsmith C, Green MM, et al. An effective deep-inspiration breath-hold radiotherapy technique for left-breast cancer: impact of post-mastectomy treatment, nodal coverage, and dose schedule on organs at risk. Breast Cancer. 2017;9:437–446.
- Loap P, Fourquet A, Kirova Y. Should We move beyond mean heart dose? Int J Radiat Oncol Biol Phys. 2020;107(2):386–387.
- Loap P, Tkatchenko N, Kirova Y. Evaluation of a delineation software for cardiac atlas-based autosegmentation: an example of the use of artificial intelligence in modern radiotherapy. Cancer Radiother. 2020;24(8):826–833.
- Loap P, Marzi LD, Kirov K, et al. Development of simplified Auto-Segmentable functional cardiac atlas. Pract Radiat Oncol. 2022;12(6):533–538.