Introduction
Intracranial germ cell tumours are rare brain tumours occurring mainly in children and young adults. The tumours can be histologically divided into pure germinoma, representing the majority of intracranial germ cell tumours, and nongerminomatous germ cell tumours (NGGCTs). The latter group includes embryonal carcinoma, choriocarcinoma, yolk sac tumours as well as teratomas that consist of mature and immature teratoma and malignant transformed teratoma [Citation1–4]. NGGCTs can present with mixed nongerminomatous histologies and can also include pure germinoma components [Citation4–6]. A diagnosis of NGGCT can be made without histological confirmation if the serum or cerebrospinal fluid alpha fetoprotein (AFP) and/or human chorionic gonadotropin (HCG) is elevated [Citation2–4,Citation7,Citation8]. While pure germinomas are very sensitive to both chemotherapy and radiotherapy (RT) and have an excellent prognosis, NGGCTs are less responsive to treatment [Citation9].
RT is essential for the treatment of all intracranial germ cell tumours [Citation4], regardless of histology or degree of dissemination. Modern RT treatment techniques include photon treatments like intensity modulation RT (IMRT) and volumetric modulated arc therapy (VMAT), or proton therapy [Citation4,Citation10–14]. While craniospinal irradiation (CSI) has been used previously, RT to the whole ventricular system (WVS) has recently emerged as the standard RT volume for localised pure germinoma [Citation4,Citation9,Citation15]. Relatively low doses in the range of 18–24 Gy seem to be sufficient to treat microscopic pure germinoma [Citation16–20].
For localised NGGCTs, there is no international consensus on the optimal target volume for RT target volumes, i.e. whether the CSI or WVS should be included vs. targeting the primary site only [Citation4]. In the American and Japanese treatment protocols, the WVS is often included either through CSI, whole brain irradiation or in a WVS target [Citation9]. In the European studies, such as SIOP-CNS-GCT-96 [Citation21] and SIOP CNS GCT II [Citation22], localised non-high risk NGGCTs were treated with chemotherapy followed by focal RT to the tumour bed, but without including intentionally the WVS. As NGGCTs often harbour pure germinoma components, the WVS might be important to treat in the context of having been recognised as target volume in the RT treatment of pure germinoma. However, due to the close proximity of the WVS to typical NGGCT sites, the WVS often receives some incidental radiation dose. This low dose may be sufficient for eradication of microscopic tumours, especially for radiosensitive pure germinoma components.
The Children’s Oncology Group (COG) phase II study ACNS1123 for localised NGGCT, in which the WVS and the tumour bed were part of the target volume, suggested the spine as the site of early recurrence, it is not clear whether the excess recurrence is a reflection of inadequate staging before RT or aggressive biology of the tumour [Citation23]. While studies of patterns of failure in NGGCTs correlating in detail the RT dose to relapses can provide insights on the appropriate RT volumes, the matter remains still unresolved, as studies reporting on tumour bed irradiation only, often are lacking dosimetric data and clear definitions of what represents a locoregional or distant failure [Citation21,Citation24]. In this context, we performed a dose planning study to evaluate the extent of incidental irradiation to the WVS when using different RT techniques for the focal treatment of NGGCTs.
Materials and methods
Anonymized data from five patients with NGGCTs previously treated with a combination of chemotherapy and passive scattering proton RT were included in this study. Two patients were originally treated with CSI followed by a boost to the tumour bed, and three patients had received focal RT to the tumour bed.
For the purpose of this planning study, the two CSI patients were replanned for focal RT by applying the original boost plans to the tumour bed but by modifying the total dose to 54 Gy (RBE) in 30 fractions. For the three patients treated with focal RT only, the original proton plans were used in the analysis. Treatments were delivered using passive scattering proton therapy and consisted of three fields, of which at least one was non-coplanar. In the original plans, the clinical target volume (CTV) of the tumour bed had been delineated and a 3 mm margin (three patients) or a 5 mm margin (two patients) was used to generate the planning target volume (PTV). The prescription dose was 54 Gy (RBE) to the PTV in 30 fractions.
For all five patients, four additional photon plans were generated: a five coplanar field intensity modulated RT plan (5 F-IMRT), a non-coplanar seven field intensity modulated RT plan (7 F-IMRT), a two coplanar arc volumetric modulated arc therapy (VMAT) plan and a TomoTherapy® plan (TOMO). 5 F-IMRT, 7 F-IMRT and VMAT were optimised using Eclipse treatment planning system (version 11.0.31, Varian Medical System, Palo Alto, CA, USA), while the TOMO plans were created using the Hi-ART System v4.2.2 (Accuray Incorporated Sunnyvale, CA). Thus, five focal RT plans with equivalent PTV coverage were available for analysis: a 5 F-IMRT, a 7 F-IMRT, a VMAT, a TOMO and a proton plan. The WVS was delineated in the structure sets according to an online available atlas developed by the COG [Citation25].
The dose delivered to 98% of the WVS (D98%), the WVS volume receiving 20 Gy (V20Gy), the WVS mean dose (Dmean) as well as Dmean to the whole brain when the PTV and WVS were subtracted (Brain-PTV-WVS) were analysed. Because the study only included five cases, no statistical significance analysis of the differences between the techniques was performed.
Results
The mean PTV volume was 65.9 cm3 [range 25.8–106.4 cm3]. Two tumours were in the pineal region, one in the suprasellar region and two were bifocal disease. The tumour size and location of the different cases are summarised in Table 1 in the Supplementary Material. An illustrative example of the dose distribution at the 95% (51.3 Gy) and 20 Gy isodose generated by the different techniques is shown in , highlighting the similar target coverage and the reduced low/intermediate-dose bath of proton vs. photon techniques.
Figure 1. Dose distribution for one example patient (Case 3), illustrating the five different techniques. Black line: planning target volume (PTV); light orange line: whole ventricular system. (1a) dose colour wash at 95% target coverage isodose (51.3 Gy); (1b) dose colour wash at 20 Gy.
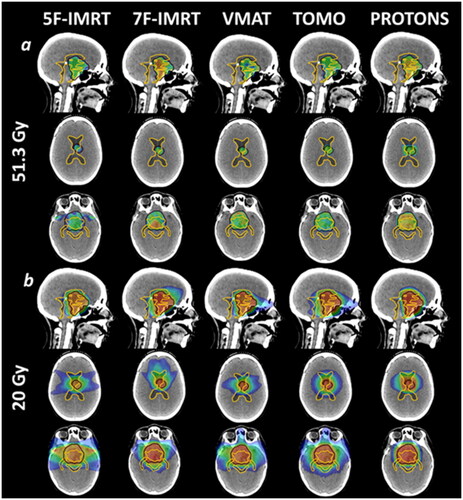
The mean D98% to the WVS was 2.9 Gy [1.0−6.5 Gy] for 5 F-IMRT, 6.8 Gy [1.6−12.8 Gy] for 7 F-IMRT, 2.9 Gy [1.1−6.8 Gy] for VMAT, 9.3 Gy [1.4–24 Gy] for TOMO and 0.1 Gy [0−0.2 Gy] for protons ().
Figure 2. Dose data for the whole ventricular system (WVS) per patient and per technique. D98%: dose covering 98% of the WVS volume; V20Gy: WVS volume receiving 20 Gy; Dmean: mean dose received by the WVS.
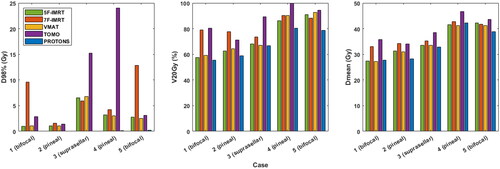
The mean WVS V20Gy was 73.1% [range 57.5−91.1%] for 5 F-IMRT, 81.7% [73.4−90.3%] for 7 F-IMRT, 74.7% [59.2−92.5%] for VMAT, 87% [71.1−99.8%] for TOMO and 68% [55.4−80.3%] for protons (). The mean WVS Dmean was 35.2 Gy [27.4−42.2 Gy] for 5 F-IMRT, 37.4 Gy [33−42.8 Gy] for 7 F-IMRT, 34.8 Gy [27.3−41.2 Gy] for VMAT, 39.7 Gy [34.1–46.7 Gy] for TOMO and 34.5 Gy [27.8−42.3 Gy] for protons (). The mean Brain-PTV-WVS Dmean was 12.9 Gy [10.2−15.6 Gy] for 5 F-IMRT, 13.6 Gy [11.8−16.1 Gy] for 7 F-IMRT, 12.4 Gy [9.7−15.2 Gy] for VMAT, 16.5 Gy [13.0−21.4 Gy] for TOMO and 8.3 Gy [6.5−11.7 Gy] for protons (Supplementary Figure 1). The full dose volume histograms (DVHs) of the WVS for the five individual cases planned with the five different techniques can be seen in Supplementary Figure 2.
Discussion
In this study, we evaluated the dose delivered to the WVS during tumour bed irradiation with different radiotherapy techniques in NGGCT irradiation. Our data indicate that the modern photon-RT techniques often irradiated a larger volume of the WVS than the proton plans with the same target volumes.
Historically, irradiation of the WVS as the standard RT volume for localised pure germinoma was established following pattern of failure studies showing recurrences occurring predominantly in the WVS when treated with focal RT to the primary site [Citation20,Citation26–28]. Studies also showed that doses as low as 18 Gy might be sufficient for eradication of microscopic pure germinoma [Citation18,Citation19]. However, NGGCTs are a heterogeneous group of tumours and it is not clear to what extent the different subtypes disseminate to the WVS. In the ACNS1123 trial, WVS RT for pure germinoma was delivered with 18 Gy, but WVS RT for NGGCT was planned to 30.6 Gy [Citation19]. Often the specific NGGCT histology of the individual patient is even not known as many cases are only diagnosed on the basis of elevated tumour markers. The mixed NGGCTs harbouring pure germinoma components are usually not separately identified and may therefore contribute further to the difficulties to associate patterns of failure to different subtypes that would allow optimisation of RT doses and volumes.
Our study demonstrates that some modern photon-RT techniques cover the WVS to a large extent, while targeting only the tumour bed, with doses that can potentially eradicate microscopic disease of pure germinoma. Indeed, the TOMO plans showed good WVS coverage even at the 20 Gy isodose level for three of the cases. Also the 7 F-IMRT covered with 20 Gy at least 75% of the WVS in all patients. The WVS coverage was less with the coplanar 5 F-IMRT and VMAT, where the difference compared to the proton plans was less pronounced. The WVS was consistently better covered by the photon plans compared to the proton plans.
The European Proton Therapy Network (EPTN) has recently published an updated atlas of brain structures that also includes the ventricular system [Citation29]. The COG atlas [Citation25] used in our study was the only atlas available at the time of delineation and differs from the EPTN atlas by including into the WVS volume the pineal-, suprasellar-, and, in case of third ventriculostomy, the prepontine cisterns.
Several studies have analysed the pattern of relapse after treatment for localised NGGCT with chemotherapy and RT only to the tumour bed: In the SIOP-96 study, 27 of the 116 treated patients presented with a recurrence. Fourteen patients had a locoregional relapse (two involving the ventricles), six had a locoregional and distant relapse (four in the ventricles), and seven were reported as distant relapse (three in the ventricles) [Citation21]. A study at the Mayo-Clinic included focal RT for 25 patients with localised NGGCT, where twelve patients developed recurrences, four were local, three in the ventricles, four in the spine and one in an unknown location [Citation30].
In contrast, the COG ACSNS1123 study aimed to investigate the outcome of localised NGGCT treated with chemotherapy followed by RT targeting the tumour bed and the WVS. The study had to be closed prematurely because more recurrences than expected were observed [Citation31]. A pattern of failure analysis showed tumour recurrence in eight out of 66 patients. All eight had recurrences in the spine. Two of these eight patients had additional areas of recurrence: one had leptomeningeal dissemination involving the brain, and another presented with locoregional recurrence extending into the ventricles [Citation23].
A recent pooled analysis of five international cooperative protocols about the pattern of failure of NGGCTs could not find an association between the development of recurrences outside the radiation field and whether a focal/WVS or CSI irradiation had been used. However, detailed RT dose data of the recurrences and the dose threshold to define the limits of the radiation fields were not presented in this analysis [Citation24].
Although our study was only performed for five cases, we can assume the target location of the primary tumours (including suprasellar, pineal gland and bifocal locations) to be representative for the most common locations of NGGCTs in Europe and North America [Citation2,Citation9], even though rarer locations such as the basal ganglia and the thalamic region have not been investigated. Interestingly, the smallest and largest PTV were bifocal tumours.
In all of our cases the dose received by the Brain-PTV-WVS volume was lower using proton therapy than modern photon radiotherapy techniques, thus demonstrating the conformality and the brain sparing potential of proton treatment in comparison with other modern RT. However, while adopting high precision techniques such as proton therapy to spare normal brain tissue, it is significantly more important to accurately define the CTV to prevent marginal recurrences, which would compromise the chance of cure.
This virtual dose planning study has the drawback, that the generated treatment plans were representing plans as we would use in our clinics. In other institutions, different conformal photon or proton techniques could be used.
The appropriate RT target for treatment of NGGCTs is yet to be defined. We demonstrated that some modern photon techniques can incidentally irradiate the WVS to a potentially therapeutic dose. Pattern of failure studies for NGGCTs should therefore detail the dose received by the WVS and by the tumour bed as well as by the recurrences to clarify the role of focal or WVS irradiation for local control of NGGCT tumours. In addition, it would be interesting to study whether patients treated with tumour bed irradiation using proton therapy have a higher risk of ventricular relapses compared to those treated with photon therapy.
Supplemental Material
Download MS Word (341.5 KB)Disclosure statement
No potential conflict of interest was reported by the author(s).
Data availability statement
The data presented in this study are available upon request.
Additional information
Funding
References
- Louis DN, Perry A, Reifenberger G, et al. The 2016 world health organization classification of tumors of the Central nervous system: a summary. Acta Neuropathol. 2016;131(6):803–820.
- Fujimaki T. Central nervous system germ cell tumors: classification, clinical features, and treatment with a historical overview. J Child Neurol. 2009;24(11):1439–1445.
- Murray M, Bartels U, Nishikawa R, et al. Consensus on the management of intracranial germ-cell tumours. Lancet Oncol. 2015;16:470–477.
- Frappaz D, Dhall G, Murray MJ, et al. EANO, SNO and Euracan consensus review on the current management and future development of intracranial germ cell tumors in adolescents and young adults. Neuro Oncol. 2022;24(4):516–527.
- Matsutani M, Sano K, Takakura K, et al. Primary intracranial germ cell tumors: a clinical analysis of 153 histologically verified cases. J Neurosurg. 1997;86(3):446–455.
- Phi JH, Wang KC, Kim SK. Intracranial germ cell tumor in the molecular era. J Korean Neurosurg Soc. 2018;61(3):333–342.
- Kanamori M, Kumabe T, Tominaga T. Is histological diagnosis necessary to start treatment for germ cell tumours in the pineal region? J Clin Neurosci. 2008;15(9):978–987.
- Venkatasai J, Balakrishnan R, Rajkrishna B, et al. A pragmatic diagnostic approach to primary intracranial germ cell tumors and their treatment outcomes. CNS Oncol. 2021;10:CNS79.
- Bowzyk Al-Naeeb A, Murray M, Horan G, et al. Current management of intracranial germ cell tumours. Clin Oncol. 2018;30(4):204–214.
- Park J, Park Y, Lee SU, et al. Differential dosimetric benefit of proton beam therapy over intensity modulated radiotherapy for a variety of targets in patients with intracranial germ cell tumors. Radiat Oncol. 2015;10:135.
- MacDonald SM, Trofimov A, Safai S, et al. Proton radiotherapy for pediatric central nervous system germ cell tumors: early clinical outcomes. Int J Radiat Oncol Biol Phys. 2011;79(1):121–129.
- Mokhtech M, Rotondo RL, Bradley JA, et al. Early outcomes and patterns of failure following proton therapy for nonmetastatic intracranial nongerminomatous germ cell tumors. Pediatr Blood Cancer. 2018;65(6):e26997.
- Greenfield BJ, Jaramillo S, Abboud M, et al. Outcomes for pediatric patients with Central nervous system germ cell tumors treated with proton therapy. Clin Transl Radiat Oncol. 2016;1:9–14.
- Correia D, Terribilini D, Zepter S, et al. Whole-ventricular irradiation for intracranial germ cell tumors: dosimetric comparison of pencil beam scanned protons, intensity-modulated radiotherapy and volumetric-modulated arc therapy. Clin Transl Radiat Oncol. 2019;15:53–61.
- Rogers S, Mosleh-Shirazi M, Saran F. Radiotherapy of localised intracranial germinoma: time to sever historical ties? Lancet Oncol. 2005;6(7):509–519.
- Lee JW, Lim DH, Sung KW, et al. Induction chemotherapy reduces radiation therapy dose and volume in the treatment of intracranial germinoma: results of the SMC-G13 tri-al. Int J Radiat Oncol Biol Phys. 2020;108(3):649–656.
- Yen SH, Chen YW, Huang PI, et al. Optimal treatment for intracranial germinoma: can we lower radiation dose without chemotherapy? Int J Radiat Oncol Biol Phys. 2010;77(4):980–987.
- Cho J, Choi JU, Kim DS, et al. Low-dose craniospinal irradiation as a definitive treatment for intracranial germinoma. Radiother Oncol. 2009;91(1):75–79.
- Foote M, Millar BA, Sahgal A, et al. Clinical outcomes of adult patients with primary intracranial germinomas treated with low-dose craniospinal radiotherapy and local boost. J Neurooncol. 2010;100(3):459–463.
- Aoyama H, Shirato H, Ikeda J, et al. Induction chemotherapy followed by low-dose involved-field radiotherapy for intracranial germ cell tumors. J Clin Oncol. 2002;20(3):857–865.
- Calaminus G, Frappaz D, Kortmann RD, et al. Outcome of patients with intracranial non-germinomatous germ cell tumors – lessons from the SIOP-CNS-GCT-96 trial. Neuro Oncol. 2017;19(12):1661–1672.
- Clinical trials.gov. Available online: https://clinicaltrials.gov/ct2/show/NCT01424839. (accessed on 22.06.2022)
- Murphy ES, Dhall G, Fangusaro J, et al. A phase 2 trial of response-based radiation therapy for localized central nervous system germ cell tumors: patterns of failure and radiation dosimetry for nongerminomatous germ cell tumors. Int J Radiat Oncol Biol Phys. 2022;113(1):143–151.
- Fonseca A, Faure-Conter C, Murray MJ, et al. Pattern of treatment failures in central nervous system non-germinomatous germ cell tumors (CNS-NGGCT): a pooled analysis of clinical trials. Neuro Oncol. 2022;24(11):1950–1961.
- ACNS1123_Atlas. Available online: https://www.qarc.org/cog/ACNS1123_Atlas.pdf. (accessed on 22.06.2022)
- Alapetite C, Brisse H, Patte C, et al. Pattern of relapse and outcome of nonmetastatic germinoma patients treated with chemotherapy and limited field radiation: the SFOP experience. Neuro Oncol. 2010;12:1318–1325.
- Calaminus G, Kortmann R, Worch J, et al. SIOP CNS GCT 96: final report of outcome of a prospective, multinational nonrandomized trial for children and adults with intracranial germinoma, comparing craniospinal irradiation alone with chemotherapy followed by focal primary site irradiation for patients with localized disease. Neuro Oncol. 2013;15(6):788–796.
- Eom KY, Kim IH, Park C, et al. Upfront chemotherapy and involved-field radiotherapy results in more relapses than extended radiotherapy for intracranial germinomas: modification in radiotherapy volume might be needed. Int J Radiat Oncol Biol Phys. 2008;71(3):667–671.
- Eekers D, Di Perri D, Roelofs E, et al. Update of the EPTN atlas for CT- and MRI-based contouring in neuro-oncology. Radiother Oncol. 2021;160:259–265.
- Breen WG, Blanchard MJ, Rao AN, et al. Optimal radiotherapy target volumes in intracranial nongerminomatous germ cell tumors: long-term institutional experience with chemotherapy, surgery, and dose- and field-adapted radiotherapy. Pediatr Blood Cancer. 2017;64(11):e26637.
- Fangusaro J, Wu S, MacDonald S, et al. Phase II trial of response-based radiation therapy for patients with localized CNS nongerminomatous germ cell tumors: a children’s oncology group study. J Clin Oncol. 2019;37(34):3283–3290.