Background
Proton therapy has been shown to target head and neck cancer tumors more precisely than photon therapy and thus reduce the dose to the surrounding organs [Citation1,Citation2]. Nevertheless, in a previous study, it was observed that proton therapy for sinonasal cancer (SNC) tended to deliver higher doses to adjacent organs at risk (OARs) in the ipsilateral side (which are traversed by the beams) than photon therapy [Citation3]. This is likely caused by the limited number of proton beam angles compared to photon beam arc configuration which smears out the dose distribution. In addition, the lateral fall-off of proton beams is degraded [Citation4,Citation5] when range shifters are used, as is often the case for SNC treatment plans.
While proton arc therapy is not yet clinically available, there has recently been much interest in exploration of this option [Citation6–9]. Several studies have shown the feasibility and potential dosimetric benefits of proton arc therapy over conventional intensity-modulated proton therapy (IMPT) for a variety of anatomical sites, including head and neck [Citation10], brain [Citation11], lung [Citation12] and prostate [Citation13].
However, the use of proton arcs or more beam directions than conventionally used for IMPT could negatively affect the robustness of the treatment. When using a conventional beam configuration, only 3–4 beam directions are generally used, allowing some flexibility in selecting robust angles. In contrast, for proton arcs or additional beam directions increases the likelihood of anatomical variations occurring in the path of some beam directions during the treatment course. This might be particularly relevant for SNC patients, who often experience large anatomical changes, such as tumor shrinkage or variations in the filling of the nasal cavities during a treatment course [Citation14,Citation15]. As such, it is important to consider the potential risks and benefits of using proton arcs for SNC patients.
In this study, we aimed to investigate the effects of adding additional beam angles in a configuration mimicking arc therapy, compared to a conventional 3–4 field configuration with respect to both dose to organs at risk and robustness to anatomical variations over the full treatment course. This was evaluated in the nominal plans, daily doses and in the doses accumulated over the entire treatment.
Material and methods
Retrospective data from 24 SNC patients treated at a single institution were used. The patient data has been described in previous studies [Citation3,Citation14] and will therefore only be presented briefly. The image data for each patient comprised a planning computed tomography scan (pCT) and daily cone-beam computed tomography scans (CBCT). All patients were previously treated with photon intensity-modulated radiation therapy (IMRT), for primary radiotherapy with a prescribed dose of 66–68 Gy in 33–34 fractions (n = 8) and for post-operative radiotherapy with 60–66 Gy in 30–33 fractions (n = 16). The study was approved by the Danish Data Protection Agency (1-16-02-676-18).
For the purpose of this study, proton plans were generated in the treatment planning system Eclipse v. 15.6 (Varian Medical Systems). The first planning strategy, S1, consisted of the conventional intensity modulated proton therapy with 3–4 beam angles. For one-sided targets, ipsilateral beam angles were chosen, although for a few cases an extra beam from the contralateral side was added. Couch rotations were used for some beam directions when needed to avoid critical structures. For central targets, beam angles were chosen depending on the critical structures. The second planning strategy, S2, consisted of 11 beam angles through the frontal part of the patient (avoiding the nose) and spaced 20 degrees between beams. We did not have the technical possibility to generate full continuous arcs for proton therapy in our treatment planning system, so these plans served as pseudo-arc substitutes with a reasonable number of beams for practical feasibility with respect to both optimization and delivery in any standard system. A range shifter of 5 cm was used for S1 for beams where the water equivalent distance between skin surface and target in the beams-eye-view was less than 4 cm. The range shifter was avoided for S2 if good target coverage and robustness could be achieved without it, but if needed was used for all 11 fields, as it would be impracticable to insert/remove it between beams. As it is general practice to use range shifter for superficial targets, the effect of using/not using range shifter was investigated by optimizing a third plan (S3) for the patients without range shifter in S2, with range shifter applied for all beams.
An example of the beam configurations is shown in . All plans were optimized with robust and multifield optimization. The robustness optimization parameters were ± 2 mm in all cardinal directions for setup uncertainty and ± 3.5% range uncertainty (14 scenarios). To evaluate the robustness, uncertainty scenarios with the same parameters were generated for all plans, and all plans had to fulfill the same criterion for target coverage as the nominal plan for initial plan approval.
Figure 1. Beam configuration for the planning strategies: (A) S1, (B) S2. S1: Conventional field configuration, S2: Pseudo-arc configuration. The gantry angles for the S1 configuration shown in this figure are 10, 65, 120 and 40 degrees. For the 40 degrees gantry angle the couch is rotated to 285 degrees, for the other gantry angles the couch is in zero degree.
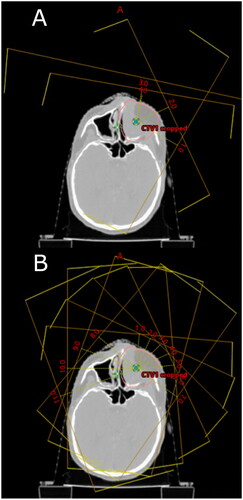
Doses to the OARs were evaluated and compared for all plans, and the near-maximum doses (D0.027 cc) and mean doses were reported.
The deliverability of the 11-field configuration plans was investigated by delivering one of the plans according to our clinical patient-specific QA program, and by estimating the delivery time. The dosimetric analysis was conducted by delivering each of the 11 fields on the 2D-detector array MatriXX (IBA Dosimetry GmbH, Germany). The measured vs expected doses were compared in two planes (1,7 cm and 4,7 cm water-equivalent depth) using the γ pass rate evaluation (3%/2 mm global, threshold >5%). All the measurements and analyses were done using myQA (IBA Dosimetry GmbH, Germany). The delivery time was estimated as the total beam-on time for the 11 fields with the gantry angle at zero, plus 30 s preparation between fields.
We subsequently evaluated the impact of anatomical variations occurring during the full treatment course by calculating the daily and accumulated doses over the entire treatment, using synthetic CTs (SynCTs) created by deforming the pCT to the daily CBCTs in the commercial software MIM Maestro v.7.0.2 [Citation16] following the procedure and validation scheme described in [Citation3].
Target coverage was evaluated for both the nominal, daily and accumulated doses. The volume of the clinical target volume receiving 95% of the prescribed dose (V95%) was quantified, which according to the Danish Head and Neck Cancer group guidelines [Citation17] must be more than 99%.
Results
All plans passed the target coverage criterion V95% > 99% for initial acceptance for both nominal plans and subsequent robustness evaluation with the uncertainty scenarios.
The pseudo-arc S2 plans gave lower doses to the OARs in the ipsilateral side than the conventional S1 plans, but at the expense of extra dose to the OARs in the contralateral side for both maximum and mean doses, . For example, the mean dose to the ipsilateral eye anterior was 6.8 Gy lower for S2 than for S1 (median over patient population), and for the ipsilateral eye posterior the mean dose was 9.8 Gy lower for S2 than for S1 (median over patient population). Similarly, the near-maximum dose to the ipsilateral optic nerve was 6.0 Gy lower for S2 than for S1 (median over patient population).
Figure 2. Maximum (A) and mean (B) doses to OARs for plans S1, S2 and S3. S1: Conventional field configuration, S2: Pseudo-arc configuration without range shifter, S3: Pseudo-arc configuration with range shifter, Ipsi: ipsilateral, Contra: contralateral.
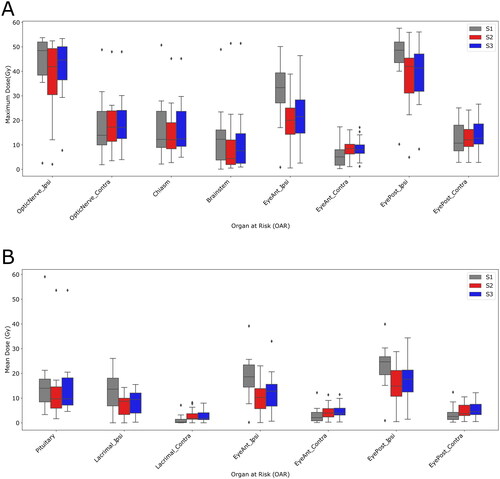
With respect to deliverability, the delivery time was 23:10 min for the whole S2 plan, including 30 s preparation between beams. All 11 fields passed the γ evaluation with γ > 97%.
The reduced dose to the ipsilateral OARs came at the expense of loss of robustness of target coverage, as shown in , especially for patients that were planned without range shifter. For those patients, the daily dose coverage was impacted negatively when calculated on the anatomy of the day. Nevertheless, the accumulated doses exhibited acceptable coverage (V95%>99%) with the S2 plan for most of the patients except three. As an example, the dose distribution for patient 11 in the nominal plan and a later fraction is shown in Supplementary Figure S1a.
Figure 3. Upper panel (A): CTV coverage (V95%) for S1 and S2. The patients with the label in red were planned with range shifter for S2. Lower panel (B): CTV coverage (V95%) for the three plans (S1, S2, S3) for the patients that did not require range shifter for S2. The stars represent the accumulated CTV coverage over the whole treatment. S1: Conventional field configuration, S2: Pseudo-arc configuration without range shifter, S3: Pseudo-arc configuration with range shifter.
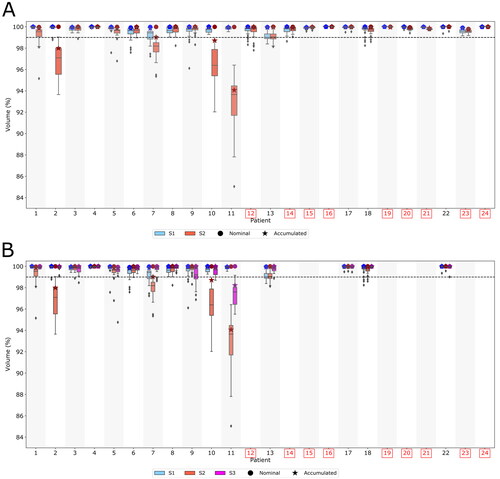
Adding range shifter to the S2 plans for which it was not initially required, resulted in an improved robustness as compared to S2 plans without range shifter, . However, for three of those patients the daily dose coverage (V95%) was still worse than for S1. In Supplementary Figure S1b, the dose distribution for patient 11 in the nominal S3 plan and a later fraction is shown. The doses to OARs in the ipsilateral side were slightly higher for the S3 plan compared to the S2 plan, but still lower than for S1 plan ().
Discussion
We compared two different proton treatment plans, one with conventional IMPT with 3–4 beams and the other a pseudo-arc configuration with 11 beams. The pseudo-arc configuration delivered lower dose to OARs in the contralateral side than the conventional IMPT plan, however, at the expense of an increased low-dose bath. The pseudo-arc configuration was less robust toward daily anatomical variations, especially when no range shifter was used.
Although the number of studies concerning proton arc therapy are increasing, no studies have yet evaluated the robustness of proton arc therapy toward daily anatomical variations. A feasibility study of proton arc therapy for head and neck cancer by Liu et al. [Citation10] showed dosimetric benefit of using proton arc therapy over 3-field IMPT plans. They observed a considerable reduction of the mean dose by 25,8% and 20,8% to the ipsilateral parotid and contralateral parotid with the proton arc plan compared to the IMPT plan. In their study, the robustness was evaluated in 21 uncertainty scenarios including isocenter shifts and stopping power ratio variations. However, no evaluation of the effect of anatomical variations during the treatment course was done.
In this study, we observed a visible negative effect on the robustness when using the 11-field configuration. A cause may be that with the 11-field configuration, larger regions susceptible to anatomical variations (such as contralateral nasal cavities) are traversed by beams. With the conventional field configuration, the changes happening on the contralateral side of the patient will have small or no effect on the delivered dose. This effect will be patient specific depending on the actual changes happening, as demonstrated for one patient with large changes (patient 11).
For patients that did not show good robustness with the S2 plan, the robustness improved considerably when adding the range shifter (plan S3). The reason for the increased robustness may be that with the range shifter, all fields can contribute to cover the target with Bragg peaks. The advantages of avoiding use of a range shifter are to be able to reduce even more the dose to the OARs surrounding the target, and to reduce the delivery time which may be an issue for arc delivery. However, our results show that while OARs benefit dosimetrically from avoiding the range shifter, the plans become less robust.
A limitation of this study is the lack of capability for optimizing full continuous arcs. While the 11-field pseudo-arc plans will be different from actual arc plans, they may serve as an indication of the advantages and limitations of using such technique regarding dose to OARs and CTV coverage robustness.
The increased delivery time with the 11-field configuration may be a downside, but this time is comparable to very modulated Intensity Modulated Radiotherapy plans [Citation18], and methods have been proposed to reduce the calculation and delivery time for proton arc therapy [Citation19,Citation20]. Optimizing delivery time was outside the scope of this study, but energy layer optimization methods as described by Gu et al. [Citation19] could be applied to our 11-field configuration to improve delivery efficiency. With lower delivery time, additional beam directions could be a viable option to further reduce dose to OARs until arc capabilities are technically possible.
In conclusion, the 11-field pseudo-arc configuration gave a noticeable dosimetric benefit for ipsilateral OARs in comparison to the conventional IMPT plan, but the robustness for target coverage was deteriorated significantly when evaluated in the daily doses. Target coverage was better in the accumulated doses than in the daily doses, but still worse than for conventional proton configuration. In future works the impact of using a smaller range shifter should be investigated, preferably with continuous full arcs.
Supplemental Material
Download MS Word (1.1 MB)Acknowledgments
The authors thank Anne Ivalu Sander Holm and Ditte Sloth Møller (Department of Oncology, Aarhus University Hospital, Aarhus, Denmark) for their help in setting up the MIM workflow for generation of synthetic CTs.
Disclosure statement
No potential conflict of interest was reported by the author(s).
Data availability statement
The data that support the findings of this study are available on request from the corresponding author, [RAP].
Additional information
Funding
References
- Levin WP, Kooy H, Loeffler JS, et al. Proton beam therapy. Br J Cancer. 2005;93(8):849–854.
- Water TA, Bijl HP, Schilstra C, et al. The potential benefit of radiotherapy with protons in head and neck cancer with respect to normal tissue sparing: a systematic review of literature. Oncologist. 2011;16(3):366–377.
- Argota-Perez R, Sharma MB, Elstrom UV, et al. Dose and robustness comparison of nominal, daily and accumulated doses for photon and proton treatment of sinonasal cancer. Radiother Oncol. 2022;173:102–108.
- Safai S, Bortfeld T, Engelsman M. Comparison between the lateral penumbra of a collimated double-scattered beam and uncollimated scanning beam in proton radiotherapy. Phys Med Biol. 2008; Mar 2153(6):1729–1750.
- Winterhalter C, Lomax A, Oxley D, et al. A study of lateral fall-off (penumbra) optimisation for pencil beam scanning (PBS) proton therapy. Phys Med Biol. 2018;63(2):025022.
- Ding X, Li X, Zhang JM, et al. Spot-Scanning proton arc (SPArc) therapy: the first robust and Delivery-Efficient Spot-Scanning proton arc therapy. Int J Radiat Oncol Biol Phys. 2016;96(5):1107–1116.
- Rah JE, Kim GY, Oh DH, et al. A treatment planning study of proton arc therapy for Para-aortic lymph node tumors: dosimetric evaluation of conventional proton therapy, proton arc therapy, and intensity modulated radiotherapy. Radiat Oncol. 2016;11(1):140.
- Ding X, Zhou J, Li X, et al. Improving dosimetric outcome for hippocampus and cochlea sparing whole brain radiotherapy using spot-scanning proton arc therapy. Acta Oncol. 2019;58(4):483–490.
- Sanchez-Parcerisa D, Kirk M, Fager M, et al. Range optimization for Mono- and bi-energetic proton modulated arc therapy with pencil beam scanning. Phys Med Biol. 2016;61(21):N565–N574.
- Liu G, Li X, Qin A, et al. Improve the dosimetric outcome in bilateral head and neck cancer (HNC) treatment using spot-scanning proton arc (SPArc) therapy: a feasibility study. Radiat Oncol. 2020;15(1):21.
- Toussaint L, Indelicato DJ, Holgersen KS, et al. Towards proton arc therapy: physical and biologically equivalent doses with increasing number of beams in pediatric brain irradiation. Acta Oncol. 2019;58(10):1451–1456.
- Liu G, Zhao L, Qin A, et al. Lung stereotactic body radiotherapy (SBRT) using Spot-Scanning proton arc (SPArc) therapy: a feasibility study. Front Oncol. 2021;11:664455.
- Ding X, Li X, Qin A, et al. Have we reached proton beam therapy dosimetric limitations? – a novel robust, delivery-efficient and continuous spot-scanning proton arc (SPArc) therapy is to improve the dosimetric outcome in treating prostate cancer. Acta Oncol. 2018; 2018/03/0457(3):435–437.
- Sharma MB, Argota Perez R, Holm AIS, et al. Air variability in maxillary sinus during radiotherapy for sinonasal carcinoma. Clin Transl Radiat Oncol. 2021;27:36–43.
- Figen M, Colpan Oksuz D, Duman E, et al. Radiotherapy for head and neck cancer: evaluation of triggered adaptive replanning in routine practice. Front Oncol. 2020;10:579917.
- Piper J, Aaron Nelson M, Harper J. Deformable image registration in MIM Maestro®. Evaluation and Description [White paper]: MIM Software Inc. 2018. https://s3.amazonaws.com/downloads.mimsoftware.com/Deformable_Image_Registration_in_MIM_Maestro_Evaluation_and_Description.pdf
- Jensen K, Friborg J, Hansen CR, et al. The danish head and neck cancer group (DAHANCA) 2020 radiotherapy guidelines. Radiother Oncol. 2020;151:149–151.
- Thomas SJ, Vinall A, Poynter A, et al. A multicentre timing study of intensity-modulated radiotherapy planning and delivery. Clin Oncol. 2010;22(8):658–665.
- Gu W, Ruan D, Lyu Q, et al. A novel energy layer optimization framework for spot-scanning proton arc therapy. Med Phys. 2020;47(5):2072–2084.
- Engwall E, Battinelli C, Wase V, et al. Fast robust optimization of proton PBS arc therapy plans using early energy layer selection and spot assignment. Phys Med Biol. 2022;67(6):065010.