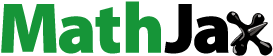
Abstract
Purpose
The purpose of this study was to evaluate three techniques of irradiation of left-sided breast cancer patients, three-dimensional conformal radiotherapy (3D-CRT), hybrid Intensity-Modulated Radiotherapy (h-IMRT), and hybrid Volumetric-Modulated Arc Therapy (h-VMAT, h-ARC), in terms of dose distribution in the planning target volume (PTV) and organs at risk (OARs). The second aim was to estimate the projected relative risk of radiation-induced secondary cancers for hybrid techniques.
Materials and methods
Three treatment plans were prepared in 3D-CRT, h-IMRT, and h-VMAT techniques for each of the 40 patients, who underwent CT simulation in deep inspiration breath-hold (DIBH). For hybrid techniques, plans were created by combining 3D-CRT and dynamic fields with an 80%/20% dose ratio for 3D-CRT and IMRT or VMAT. Cumulative dose–volume histograms were used to compare dose distributions within the PTV and OARs (heart, left anterior descending coronary artery [LAD], left and right lung [LL, RL], right breast [RB]). Projected risk ratios for secondary cancers were estimated relative to 3D-CRT using the organ equivalent dose (OED) concept for the Schneider’s linear exponential, plateau, and full mechanistic dose–response model.
Results
All plans fulfilled the PTV criterium: V95%≥95%. Compared to 3D-CRT, both hybrid techniques showed significantly better target coverage (PTV: V95%>98%, p < 0.001), and the best conformality was achieved by h-ARC plans (CI: 1.18 ± 0.09, p < 0.001). Compared to 3D-CRT and h-ARC, h-IMRT increased the average sum of monitor units (MU) over 129.9% (p < 0.001). H-ARC increased the mean dose of contralateral organs and the LL V5Gy parameter (p < 0.001). Both hybrid techniques significantly reduced the Dmax of the heart by 5 Gy. Compared to h-IMRT, h-ARC increased secondary cancer projected relative risk ratios for LL, RL, and RB by 18, 152, and 81%, respectively.
Conclusions
The results confirmed that both hybrid techniques provide better target quality and OARs sparing than 3D-CRT. Hybrid VMAT delivers less MU compared to hybrid IMRT but may increase the risk of radiation-induced secondary malignancies.
Introduction
In 2020, breast cancer was the most commonly diagnosed in women (11.7% of total cases), and the fifth leading cause of cancer mortality worldwide (6.9% of all cancer deaths) [Citation1]. Demographic forecasts and past global incidence and mortality trends show that by 2040, the number of newly diagnosed breast cancers is projected to grow by over 40%, to about three million cases every year [Citation2]. Breast cancer treatment can be highly effective, especially when the disease is diagnosed at an early stage – the 5-year relative survival rates exceed 90% in most high-income countries [Citation3]. The improvement in overall survival rates necessitates the need of providing patients with a good quality of life after treatment, hence minimizing radiotherapy-related complications.
Radiotherapy as an adjuvant therapy to surgery and chemotherapy is crucial as it improves local control and long-term survival as well as reduces the risk of disease recurrence by half [Citation4]. However, apart from advantageous effects, irradiation may cause detrimental side effects on healthy tissues. Therefore, to reduce the dose in organs at risk (OAR) and prevent long-term complications, various irradiation techniques have been developed. These include deep inspiration breath hold (DIBH) which has become the gold standard in treating left-sided breast cancer because besides the significant heart and left anterior descending coronary artery (LAD) dose reduction [Citation5–8], this method also minimizes positioning uncertainties and thus discrepancies between planned and delivered dose distributions [Citation9–11]. In recent years, conventional three-dimensional conformal radiotherapy (3D-CRT) has been superseded by intensity-modulated radiotherapy (IMRT) and volumetric-modulated arc therapy (VMAT) to provide more homogeneous and conformal dose distribution in breast cancer treatment. Nevertheless, numerous studies [Citation12–14] found that, in contrast to 3D-CRT, those dynamic techniques are likewise characterized by significantly larger low-dose volumes in surrounding normal tissues. This is a major concern when using IMRT or VMAT, since larger volumes of normal tissue exposed to lower doses may increase a radiation-induced risk of secondary malignancies [Citation15–17].
To provide excellent target coverage and overcome the low-dose bath to normal tissue, an innovative hybrid method has been developed. The hybrid radiation technique is a combination of 3D-CRT and IMRT/VMAT fields with different dose weightings. Many studies have reported that hybrids accumulate the benefits of static and dynamic techniques, thus providing balanced results for planning target volume (PTV) coverage and OAR sparing [Citation18–21].
Most of the published data have discussed the benefits of hybrids for whole breast irradiation in terms of target coverage and OARs sparing, whereas studies assessing their clinical impact and radiation toxicity are sparse [Citation22,Citation23]. Due to the improvement in the efficacy and overall survival of breast cancer patients, it is crucial to research the long-term side effects of radiotherapy. Therefore, the purpose of the present study is to estimate a projected relative risk of radiation-induced secondary cancers for hybrid techniques and perform a dosimetric comparison of 3D-CRT, hybrid IMRT, and hybrid VMAT plans for left-sided breast cancer patients.
Materials and methods
Patient selection, CT simulation, and delineation
Forty women diagnosed with early-stage left-sided breast cancer (T1–2N0M0) were selected for this retrospective planning study. All patients underwent breast-conserving surgery and were irradiated in our facility during the years 2017–2018. Radiotherapy was delivered in 3D-CRT technique using the deep inspiration breath hold (DIBH) method performed by the Real-Time Position Management system (RPM, Varian Medical Systems, Palo Alto, CA, USA). To differentiate the study group and prevent selection bias, the patients were selected randomly with no selection criteria. The median age of patients’ was 60 (range 31–70).
The CT for radiotherapy planning was performed in the supine position with arms positioned above the head with a commercial immobilization system (Posiboard, CIVCO Medical Solutions, Kalona, IA, USA). The thickness of the scan slice was 3 mm. A radiation oncologist defined the target volume and the organs at risk including the heart, left anterior descending coronary artery (LAD), left lung (LL), right lung (RL), and right breast (RB). The clinical target volume (CTV) included the whole breast and was delineated according to the European Society for Radiotherapy and Oncology (ESTRO) delineation guideline [Citation24]. The planning target volume (PTV) was obtained by adding a 5 mm geometrical margin to the CTV. All target structures were cropped at 5 mm from the skin surface.
Treatment planning
Three treatment plans were created for each patient: 3D-CRT, hybrid IMRT, and hybrid VMAT. All plans were prepared for the DIBH technique in the Eclipse External Beam planning system Version 13.0.33 (Varian Medical System, Palo Alto, CA, USA) using the Anisotropic Analytical Algorithm (AAA) Version 13.0.26. The total prescribed dose to the PTV was 42.5 Gy in 17 fractions (fraction dose 2.5 Gy). Each plan was normalized to ensure that the mean dose (Dmean) received by the PTV was equal to 100% of the prescribed dose. To minimize planning bias, two independent medical physicists were responsible for preparing and optimizing treatment plans.
Due to different normalizations, clinical 3D-CRT plans were not included in this study. Replanned 3D-CRT plans consisted of 2–7 tangential beams with 6 MV and/or 15 MV photons. Gantry angles were selected to provide the best target coverage while limiting dose exposure to the heart, ipsilateral lung, and contralateral breast. Field-in-field technique was also used, if necessary.
The hybrid IMRT (h-IMRT) plans consisted of two tangential static fields (6 MV photons) delivering 80% of the prescribed dose to PTV and six dynamic IMRT fields (6 MV photons) providing the remaining 20% of the prescribed dose. IMRT fields were positioned around the left breast with gantry angles: 300°, 315°, 330°, 95°, 110°, and 125°. Collimator angles were settled as follows: 10°, 10°, 10°, 350°, 350°, and 350°.
The hybrid VMAT (h-ARC) plans included two tangential static fields (6 MV photons) that delivered 80% of the prescribed dose and the dynamic partial arcs (6 MV photons) providing the final 20% of the prescribed dose to PTV. Partial arcs were irradiating between gantry angles 305°–140°, 140°–305°, and 305°–140° with collimator angles 20°, 357°, and 100°. The beam arrangements for h-IMRT and h-ARC plans are illustrated in Figure S1 in the Supplementary Materials.
H-IMRT and h-VMAT plans were optimized using Progressive Resolution Optimizer Version 13.0.26. lists the dose–volume constraints for PTV and OARs that were set as a starting point for each hybrid plan optimization. The highest priority was to cover at least 95% of each PTV by 95% of the prescribed dose. A further focus for the planning optimization was dose constraints for the heart and LAD. Dose constraints for the LL, RL, and RB were set with lesser priority. Since the present study focused on the heart and LAD sparing, their dose–volume constraints were continuously pushed to lower doses whenever the optimizer achieved the planning goals without compromising PTV coverage and conformality. To adequately protect lower-priority OARs, more radical dose–volume constraints than those recommended by the Radiation Therapy Oncology Group (RTOG) were applied. Considering a potential change in the shape of the breast following radiation and to improve the robustness of dynamic plans, PTV was covered with a 10 mm virtual bolus during the optimization process. The bolus was removed for the final dose calculation.
Table 1. Planning objectives for h-IMRT and h-ARC optimization.
Calculation of secondary cancer risk estimates
Excess absolute risk (EAR), which describes the difference in the absolute risk of disease between persons exposed to radiation and those who were not (per 10,000 person-years), can be used to describe the projected risk of a solid secondary malignancy developing after radiotherapy. The EAR can be calculated using Schneider’s concept of organ equivalent dose (OED) [Citation25,Citation26]:
(1)
(1)
(2)
(2)
where EARorg stands for an excess absolute risk for a whole organ, VT is the total volume of the organ to be analyzed, V(Di) is the volume of an organ that receives irradiation dose Di, β is the initial slope of the dose–response curve at a low dose and RED(Di) is a function describing dose–response relationship for radiation-induced cancer in units of dose Di. Formula μ(agex, agea) includes population-related factors, such as age at exposure (agex), attained age (agea) and age-modifying parameters.
EquationEquation (3)(3)
(3) describes the risk ratio, which was used to compare treatment modalities in this study:
(3)
(3)
Risk ratio, is equivalent to OED ratios; therefore, corresponding uncertainties are kept at a minimum, because OED values are independent of the initial slope β and population-dependent factors.
The OEDs for LL, RL, and RB were calculated based on the differential DVHs for three models for carcinoma induction developed by Schneider et al. [Citation25,Citation26]:
Full mechanistic model which includes the impact of fractionation, cell destruction, cell repair, and cell regeneration effects
(4)
(4)
Linear-exponential model which neglects any cell repopulation/repair effect (R = 0)
(5)
(5)
Plateau model for full repopulation/repair effect (R = 1)
(6)
(6)
where VT is the total volume of the organ, V(Di) is the volume of an organ that receives dose Di, R is a repopulation parameter and α’ represents cell destruction effect and is defined as follows:
(7)
(7)
where d stands for dose per fraction, D for total dose, and N is a number of fractions, α and β are the parameters of the linear-quadratic model.
OEDs were calculated using the following parameters reported by Schneider et al. [Citation26]: α = 0.044, R = 0.15 for a right breast and =0.042, R = 0.83 for lungs, assuming an α/β value of 3 Gy.
Conformity and homogeneity indices
The conformity index and homogeneity index were calculated to evaluate the dose distribution.
The conformity index (CI) was calculated using the following formula:
(8)
where V95% is the volume of isodose of 95% of prescribed dose and VPTV is the planning target volume.
The homogeneity index (HI) was calculated using the ICRU report 83 definition [Citation27]:
(9)
where DX% is a dose received by the X% of PTV.
Statistical analysis
SPSS 29.0.0.0 software (IBM, Armonk, NY, USA) was used for the statistical analysis. To evaluate the normality of the data, Shapiro–Wilk’s test was used. Quantitative data were expressed as mean ± standard deviation when being normally distributed and as median ± quartile deviation when they were not. Multiple groups of means were compared by one-way ANOVA after testing for equality of variance, which was assessed with Levene’s test. Multiple groups of medians were compared using Kruskal–Wallis one-way ANOVA. The post hoc least significant difference (LSD) test was used for multiple testing. In all cases, differences were considered statistically significant at p < 0.05. The correlation coefficients (r) were calculated using the Pearson’s correlation.
Results
Dosimetric results
summarizes the dosimetric comparison for hybrid and 3D-CRT techniques. All plans fulfilled the planning criterion for PTV coverage: D95% ≥95% of prescribed dose (40.375 Gy). Coverage of PTV and CTV was significantly better in hybrid plans compared to 3D-CRT (p < 0.001). Both hybrid techniques achieved comparable results for the PTV V95% parameter (h-IMRT:98.81 ± 0.60; h-ARC:98.34 ± 0.67). Compared to 3D-CRT, hybrid plans showed significantly different homogeneity and conformity indices. Better conformity was obtained by h-ARC (1.18 ± 0.09, p < 0.001), and h-IMRT technique improved the homogeneity of a dose distribution (0.07 ± 0.01, p < 0.001). The average sum of monitor units in the treatment plans was visibly higher in h-IMRT technique (800.0 ± 378.6, p < 0.001). 3D-CRT and h-ARC plans achieved similar results and reduced MU number by more than half compared to h-IMRT (p < 0.001).
Table 2. Dosimetric parameters comparison between 3D-CRT and hybrid (h-IMRT, h-ARC) techniques.
shows comparison of average DVHs between 3D-CRT, h-IMRT and h-ARC plans. Although the heart mean dose was comparable among all plans (ca. 2 Gy), the hybrid plans resulted in Dmax reduction by 5 Gy compared to 3D-CRT (h-IMRT: p = 0.039, h-ARC: p = 0.014). The volumes of heart that received a dose of 5 Gy and 10 Gy were smaller in h-ARC plans but results didn’t reach statistical significance. The LAD Dmean, Dmax, and D10% were also lower in h-ARC plans but obtained differences were not statistically significant. Hybrid VMAT-combined plans significantly increased the left lung volume receiving 5 Gy (p < 0.001), whereas Dmean, V10 and V20Gy were similar in all plans. The RL Dmean and Dmax were comparable in 3D-CRT and h-IMRT plans. H-ARC plans increased Dmax of RL and Dmean of RB (RL: p < 0.023, RB: p = 0.130) but also resulted in RB Dmax reduction. The maximum dose to right breast was reduced by more than 30% using partial arcs, but no statistically significance was found. compares the isodose distribution in the axial plane between analyzed modalities: 3D-CRT, h-IMRT and h-ARC. The color-wash thresholds were: 3, 5, and 40.375 Gy which is 95% of the prescribed dose.
Organ equivalent dose and projected relative risk
summarizes the calculated mean OED values for each of the three treatment modalities for 40 patients. In all analyzed organs, OEDs were equal to or less than the mean dose, for left lung the difference was up to 50%. Figure S2 in the Supplementary Materials presents comparison of OEDs for all analyzed techniques and Schneider’s models. The OEDs were higher for h-ARC plans (p < 0.001), 3D-CRT plans resulted in similar or slightly lower values in comparison to h-IMRT, but with no statistically significant difference. Figure S3 in the Supplementary Materials shows correlations between total MU in a plan and OED values for all analyzed OARs and treatment modalities. Only h-IMRT plans had a strong correlation between MUs and OEDs (LL: r = 0.6244, p < 0.001; RL: r = 0.7605, p < 0.001, RB: r = 0.7204, p < 0.001).
Table 3. Comparison of OED for left lung, right lung and right breast.
presents the projected relative risk ratios of radiation-induced secondary cancers for all hybrid techniques relative to 3D-CRT. depicts the estimates of the projected relative risk ratios of secondary lung and contralateral breast cancer induction for each patient according to full mechanistic dose-response model. According to all three Schneider’s models, h-ARC increased the projected probability of radiation-induced carcinomas when compared to 3D-CRT plans, however certain cases showed a slightly lower risk estimates for left lung (patient 30, ) and right breast (patient 39, ).
Figure 3. Relative risks ratios of secondary cancer based on the full mechanistic dose-response model for left lung, right lung and right breast, for each patient.
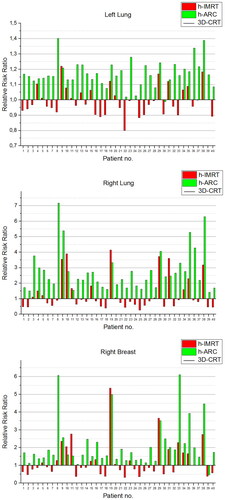
Table 4. Relative risk ratios for secondary carcinomas (left lung, right lung, right breast) assessed using the Schneider’s models.
Discussion
In this study, the two different hybrid techniques, h-IMRT and h-ARC, were investigated for early-stage left-sided breast cancer. There are studies [Citation18,Citation19,Citation28–30] which have indicated that comparing to fully dynamic plans, the hybrid method achieves balance between excellent homogenous and conformal dose distribution in PTV and small low-dose volumes in surrounding normal tissues. Bi et al. [Citation29] evaluated three treatment modalities: full IMRT, hybrid IMRT and hybrid VMAT for early-stage breast cancer. Authors found that compared to full IMRT, hybrid IMRT decreased the Dmean of contralateral lung by 10 Gy and the V10Gy of ipsilateral lung by 4%. Lin et al. [Citation19] demonstrated benefits of H-VMAT technique over pure-VMAT and a fixed-field IMRT technique. They demonstrated that hybrid-VMAT not only generated the best results in dose coverage of the target but also significantly decreased volumes of the ipsilateral lung exposed to doses of 20 and 5 Gy and reduced Dmean of the heart and the contralateral breast over 50%. Comparing to our previous work [Citation31], in which we analyzed two VMAT geometries for left-sided breast irradiation, hybrid VMAT technique not only reduced V5 and V10Gy of left lung by 17 and 7%, respectively, but also drastically decreased Dmean and Dmax in contralateral OARs by >60 and >55%, respectively. The V5Gy parameter for contralateral organs was kept at 1% using hybrids, while in full VMAT plans it was 8% for contralateral lung and 17% for contralateral breast. However, maximum heart and LAD doses were lower, on average, by 15 Gy with full VMAT plans. In this study, h-IMRT and h-ARC achieved similar results in PTV coverage and heart and LAD sparing. H-IMRT reduced V5Gy of left lung by 7% as well as Dmean and Dmax of right lung by 62% and 30%, respectively, compared to h-ARC plans. Nevertheless, h-IMRT plans generated twice as high MU values compared to h-ARC and 3D-CRT technique. More monitor units not only transport MLC leakage and scattered radiation to out-of-field regions which increase the total body dose [Citation32,Citation33], but also may greatly extend beam-on time [Citation21,Citation34,Citation35]. The treatment time plays an important role in DIBH, because with an increased beam-on time, more breathing cycles have to be completed per fraction what can makes whole treatment less feasible especially for elderly patients who may not be able to hold their breath for such a long time. Since the dynamic and hybrid plans are characterized by steep dose gradient outside the target volume, DIBH method should be definitely taken into consideration, because besides the significant heart and LAD dose reduction [Citation5–8], this method also minimizes positioning uncertainties and thus discrepancies between planned and delivered dose distribution [Citation9–11].
In this study, hybrid plans were created by combining 3DCRT and IMRT/VMAT techniques with a PTV-dose proportion: 80% of 3D-CRT and 20% of IMRT/VMAT. Balaji et al. [Citation36] compared different dose weighting for left-sided chest wall radiation. The authors found that 90% 3DCRT/10% VMAT ratio gives unacceptable conformity and inadequate modulation during VMAT optimization; therefore, to achieve balanced results for target coverage and OARs sparing, the optimal combination for hybrids is 70–80% for 3DCRT and 20–30% for VMAT. There are some studies where hybrid plans were generated by combining IMRT and VMAT techniques. Lin et al. [Citation19], Sathiyaraj et al. [Citation37], Aly et al. [Citation38], and Jöst et al. [Citation20] have indicated that hybrids (IMRT + VMAT) were also superior to static and/or fully dynamic plans. Nevertheless, in contrast to 3D-CRT, IMRT fields as a base dose plan in combination with VMAT increase MU, delivery time as well as uncertainties related to patient’s setup and breathing effects. Venjakob et al. [Citation39] analyzed different H-VMAT configurations varying beam energy and the application or omission of hard wedge filters (HWF) in the 3D-CRT base-dose plans. Their results showed that the choice of higher beam energy and using HWF is significantly disadvantageous for quality indices as well as PTV coverage and OARs sparing. For this reason, in our study, both the 3D-CRT base-dose plans and dynamic plans consisted of 6 MV photon beams and no wedge filters were used. Alternatively, there are studies which have reported on the benefits of using flattening filter-free (FFF) beams in hybrid plans for whole-breast radiotherapy. For instance, Spruijt et al. [Citation40] and Bahrainy et al. [Citation41] demonstrated that target volume metrics for flattened and FFF plans were comparable, but FFF-based plans resulted in the average treatment time reduction. According to Balaji et al. [Citation34], in contrast to flat beams, FFF has a minimal hot-dose region over the breast surface, which contributes to a reduced skin dose and potentially improved cosmetic results. On the other hand, authors noted that the most common restriction of FFF beams is their usage with large fields, therefore further research is required to determine whether FFF beams are feasible for the breast/chest wall with supraclavicular nodal areas irradiation.
The OED values and hence projected radiation-induced secondary cancer risk were comparable for linear-exponential, plateau, and full mechanistic dose–response models. It results from similarities in dose–effect relationship up to dose levels attained in this study [Citation26,Citation42]. Studies evaluating hybrid techniques in terms of estimating the projected risk of secondary radiation-induced malignancies are rare. Bi et al. [Citation22] compared three modalities for whole breast radiotherapy with simultaneous integrated boost: full IMRT, hybrid IMRT, and hybrid VMAT. According to their research, the lowest EAR values for the contralateral breast, contralateral lung and esophagus were achieved with hybrid IMRT plans, which reduced EARs by 33, 70, and 58%, respectively, in comparison to hybrid VMAT and by 9, 42, and 56%, respectively, in comparison to full IMRT plans. Similarly, in this study, comparison of the two different hybrid techniques indicated a relevant increase in projected relative risk ratios for h-ARC plans. Obtained relative risk ratios estimates for ipsilateral lung, contralateral lung and contralateral breast were 18, 152, and 81% higher, respectively, compared to those calculated for h-IMRT. Only a few publications have attempted to find a correlation between the risk of second malignancies and a number of monitor units. For instance, Hall EJ. suggests that the probability of radiation-induced second malignancies rises with the number of monitor units, which is related, among others, to the higher total-body dose due to leaking and scattered radiation [Citation32]. On the other hand, according to Sakthivel et al. [Citation42], there is no correlation between the calculated EAR and the MU for dynamic techniques. In the present study, only h-IMRT plans demonstrated a strong correlation between MUs and OEDs, which are proportional to the EARs. However, it should be mentioned that h-IMRT technique significantly increased the average sum of monitor units in the treatment plans over 129.89% compared to 3D-CRT and h-ARC technique.
There are certain limitations to this study. One is a relatively small number of cases. Individual anatomical features can have a significant influence on organ doses and thus on estimation of the projected relative risk of radiation-induced secondary malignancies. The second limitation is the fact that all the plans were calculated using the Anisotropic Analytical Algorithm (AAA) which may bring additional uncertainties in terms of dose calculation in inhomogeneous regions. To overcome the limitations of AAA and improve the accuracy of dose calculations in heterogeneous media, Varian (Varian Medical Systems, Palo Alto, USA) developed the Acuros External Beam (AXB) algorithm in 2010. Although Kumar et al. [Citation43] showed that, comparing these two algorithms, AXB may be able to better estimate lung toxicities and treatment outcome in DIBH, Guebert et al. [Citation44] stated that there are minimal insignificant differences in both magnitude and spatial dose distribution for breast cancer radiotherapy planning. Joosten et al. [Citation45] observed that comparing to Monte Carlo (MC) simulations, the convolution/superposition algorithm can bring additional uncertainties in out-of-field dosimetry. Authors found that large fraction of the out-of-field dose comes from the out-of-field head scatter fluence which is not adequately modeled by the convolution/superposition algorithm. Furthermore, all hybrid plans were optimized using the default smoothing parameters. Saroj et al. [Citation46] found that increasing the smoothing levels reduces the MUs and smoothes the fluence, with an insignificant variation of the dosimetric parameters on PTV and OARs. Another limitation is that in all cases we used the same h-IMRT and h-ARC geometry. The gantry angle range was set to 185° for h-IMRT and 195° for h-VMAT in order to improve field regulation ability and achieve better homogeneity in the target area. There are studies [Citation47–51] confirming that limiting the IMRT fields and VMAT start/stop degrees to tangential fields, applying the ‘butterfly-VMAT‘ technique, or using fewer long arcs can further reduce doses in contralateral organs and beam-on time as well as decrease V5% parameter of total body. It should be noted that, as we did not have access to the medical histories of the analyzed patients, the predicted relative risk of secondary cancer did not include the baseline risk; therefore, the relative risk we estimated comes exclusively from the irradiation. Furthermore, the calculated OEDs and thus projected relative risks are highly dependent on the specific dose plans and geometry used; therefore, the obtained results should be interpreted with caution and used as an additional useful metric while assessing treatment modalities.
Conclusion
The hybrid method achieves balance between excellent homogenous and conformal dose distribution in target structure and the volume of normal tissues receiving a low radiation bath, which still remains a major concern in purely dynamic plans. The present dosimetric study shows that both h-IMRT and h-VMAT provided comparable results in terms of PTV coverage. The comparison of hybrid techniques indicated that h-VMAT plans significantly reduced MU, which may give logistic benefits during DIBH therapy and h-IMRT considerably decreased the projected secondary cancer risk ratio for the contralateral breast and lungs. Nevertheless, projected relative risk ratio estimates should be evaluated cautiously due to potential uncertainties, hence additional theoretical research and prospective clinical trials with a long-term follow-up are required to determine the correlation between treatment techniques and risk of radiation-induced secondary malignancies.
Supplemental Material
Download MS Word (941.4 KB)Disclosure statement
No potential conflict of interest was reported by the author(s).
References
- Sung H, Ferlay J, Siegel RL, et al. Global cancer statistics 2020: GLOBOCAN estimates of incidence and mortality worldwide for 36 cancers in 185 countries. CA Cancer J Clin. 2021;71(3):209–249. doi: 10.3322/caac.21660.
- Arnold M, Morgan E, Rumgay H, et al. Current and future burden of breast cancer: Global statistics for 2020 and 2040. Breast. 2022;66:15–23. doi: 10.1016/j.breast.2022.08.010.
- Allemani C, Matsuda T, Di Carlo V, et al. Global surveillance of trends in cancer survival 2000-14 (CONCORD-3): analysis of individual records for 37,513,025 patients diagnosed with one of 18 cancers from 322 population-based registries in 71 countries. Lancet. 2018;391(10125):1023–1075. doi: 10.1016/S0140-6736(17)33326-3.
- Darby S, McGale P, Correa C, Early Breast Cancer Trialists’ Collaborative Group (EBCTCG), et al. Effect of radiotherapy after breast-conserving surgery on 10-year recurrence and 15-year breast cancer death: meta-analysis of individual patient data for 10,801 women in 17 randomised trials. Lancet. 2011;378(9804):1707–1716. doi: 10.1016/S0140-6736(11)61629-2.
- Vikström J, Hjelstuen MH, Mjaaland I, et al. Cardiac and pulmonary dose reduction for tangentially irradiated breast cancer, utilizing deep inspiration breath-hold with audio-visual guidance, without compromising target coverage. Acta Oncol. 2011;50(1):42–50. doi: 10.3109/0284186X.2010.512923.
- Hjelstuen MH, Mjaaland I, Vikström J, et al. Radiation during deep inspiration allows loco-regional treatment of left breast and axillary-, supraclavicular- and internal mammary lymph nodes without compromising target coverage or dose restrictions to organs at risk. Acta Oncol. 2012;51(3):333–344. doi: 10.3109/0284186X.2011.618510.
- Berg M, Lorenzen EL, Jensen I, et al. The potential benefits from respiratory gating for breast cancer patients regarding target coverage and dose to organs at risk when applying strict dose limits to the heart: results from the DBCG HYPO trial. Acta Oncol. 2018;57(1):113–119. doi: 10.1080/0284186X.2017.1406139.
- Vikström J, Hjelstuen MH, Wasbø E, et al. A comparison of conventional and dynamic radiotherapy planning techniques for early-stage breast cancer utilizing deep inspiration breath-hold. Acta Oncol. 2018;57(10):1325–1330. doi: 10.1080/0284186X.2018.1497294.
- Zhou S, Zhu X, Zhang M, et al. Estimation of internal organ motion-induced variance in radiation dose in non-gated radiotherapy. Phys Med Biol. 2016;61(23):8157–8179. doi: 10.1088/0031-9155/61/23/8157.
- Ramasubramanian V, Balaji K, Balaji Subramanian S, et al. Hybrid volumetric modulated arc therapy for whole breast irradiation: a dosimetric comparison of different arc designs. Radiol Med. 2019;124(6):546–554. doi: 10.1007/s11547-019-00994-1.
- Michalski A, Atyeo J, Cox J, et al. Inter- and intra-fraction motion during radiation therapy to the whole breast in the supine position: a systematic review. J Med Imaging Radiat Oncol. 2012;56(5):499–509. doi: 10.1111/j.1754-9485.2012.02434.x.
- Moon SH, Shin KH, Kim TH, et al. Dosimetric comparison of four different external beam partial breast irradiation techniques: three-dimensional conformal radiotherapy, intensity-modulated radiotherapy, helical tomotherapy, and proton beam therapy. Radiother Oncol. 2009;90(1):66–73. doi: 10.1016/j.radonc.2008.09.027.
- Beckham WA, Popescu CC, Patenaude VV, et al. Is multibeam IMRT better than standard treatment for patients with left-sided breast cancer? Int J Radiat Oncol Biol Phys. 2007;69(3):918–924. doi: 10.1016/j.ijrobp.2007.06.060.
- Haciislamoglu E, Cinar Y, Gurcan F, et al. Secondary cancer risk after whole-breast radiation therapy: field-in-field versus intensity modulated radiation therapy versus volumetric modulated arc therapy. Br J Radiol. 2019;92(1102):20190317. doi: 10.1259/bjr.20190317.
- Lee B, Lee S, Sung J, et al. Radiotherapy-induced secondary cancer risk for breast cancer: 3D conformal therapy versus IMRT versus VMAT. J Radiol Prot. 2014;34(2):325–331. doi: 10.1088/0952-4746/34/2/325.
- Paganetti H. Assessment of the risk for developing a second malignancy from scattered and secondary radiation in radiation therapy. Health Phys. 2012;103(5):652–661. doi: 10.1097/HP.0b013e318261113d.
- National Research Council. Health risks from exposure to low levels of ionizing radiation: BEIR VII phase 2. Washington DC: The National Academies Press; 2006.
- Mayo CS, Urie MM, Fitzgerald TJ. Hybrid IMRT plans–concurrently treating conventional and IMRT beams for improved breast irradiation and reduced planning time. Int J Radiat Oncol Biol Phys. 2005;61(3):922–932. doi: 10.1016/j.ijrobp.2004.10.033.
- Lin JF, Yeh DC, Yeh HL, et al. Dosimetric comparison of hybrid volumetric-modulated arc therapy, volumetric-modulated arc therapy, and intensity-modulated radiation therapy for left-sided early breast cancer. Med Dosim. 2015;40(3):262–267. doi: 10.1016/j.meddos.2015.05.003.
- Jöst V, Kretschmer M, Sabatino M, et al. Heart dose reduction in breast cancer treatment with simultaneous integrated boost. Strahlenther Onkol. 2015;191(9):734–741. doi: 10.1007/s00066-015-0874-7.
- Chen YG, Li AC, Li WY, et al. The feasibility study of a hybrid coplanar arc technique Versus hybrid intensity-modulated radiotherapy in treatment of early-stage left-sided breast cancer with simultaneous-integrated boost. J Med Phys. 2017;42(1):1–8. doi: 10.4103/jmp.JMP_105_16.
- Bi S, Zhu R, Dai Z. Dosimetric and radiobiological comparison of simultaneous integrated boost radiotherapy for early stage right side breast cancer between three techniques: IMRT, hybrid IMRT and hybrid VMAT. Radiat Oncol. 2022;17(1):60. doi: 10.1186/s13014-022-02009-2.
- Zhang Q, Zeng Y, Peng Y, et al. Critical evaluation of secondary cancer risk after breast radiation therapy with hybrid radiotherapy techniques. Breast Cancer. 2023;15:25–38. doi: 10.2147/BCTT.S383369.
- Offersen BV, Boersma LJ, Kirkove C, et al. ESTRO consensus guideline on target volume delineation for elective radiation therapy of early stage breast cancer. Radiother Oncol. 2015;114(1):3–10. doi: 10.1016/j.radonc.2014.11.030.
- Schneider U. Mechanistic model of radiation-induced cancer after fractionated radiotherapy using the linear-quadratic formula. Med Phys. 2009;36(4):1138–1143. doi: 10.1118/1.3089792.
- Schneider U, Sumila M, Robotka J. Site-specific dose-response relationships for cancer induction from the combined Japanese A-bomb and Hodgkin cohorts for doses relevant to radiotherapy. Theor Biol Med Model. 2011;8:27. doi: 10.1186/1742-4682-8-27.
- ICRU. Report 83. Prescribing, recording, and reporting photon-beam intensity-modulated radiation therapy (IMRT). J ICRP. 2010;10(1):34–36. doi: 10.1093/jicru/ndq002.
- Lang K, Loritz B, Schwartz A, et al. Dosimetric comparison between volumetric-modulated arc therapy and a hybrid volumetric-modulated arc therapy and segmented field-in-field technique for postmastectomy chest wall and regional lymph node irradiation. Med Dosim. 2020;45(2):121–127. doi: 10.1016/j.meddos.2019.08.001.
- Bi S, Zhu R, Sun X, et al. Dosimetric comparison of IMRT, hybrid IMRT and hybrid VMAT for early stage right-sided breast cancer. Clin Oncol. 2021;6:1808.
- Jeulink M, Dahele M, Meijnen P, et al. Is there a preferred IMRT technique for left-breast irradiation? J Appl Clin Med Phys. 2015;16(3):5266.
- Racka I, Majewska K, Winiecki J. Three-dimensional conformal radiotherapy (3D-CRT) vs. volumetric modulated arc therapy (VMAT) in deep inspiration breath-hold (DIBH) technique in left-sided breast cancer patients-comparative analysis of dose distribution and estimation of projected secondary cancer risk. Strahlenther Onkol. 2023;199(1):90–101. doi: 10.1007/s00066-022-01979-2.
- Hall EJ. Intensity-modulated radiation therapy, protons, and the risk of second cancers. Int J Radiat Oncol Biol Phys. 2006;65(1):1–7. doi: 10.1016/j.ijrobp.2006.01.027.
- Clemente S, Cozzolino M, Chiumento C, et al. Monitor unit optimization in RapidArc plans for prostate cancer. J Appl Clin Med Phys. 2013;14(3):4114.
- Balaji K, Balaji Subramanian S, Sathiya K, et al. Hybrid planning techniques for hypofractionated whole-breast irradiation using flattening filter-free beams. Strahlenther Onkol. 2020;196(4):376–385. doi: 10.1007/s00066-019-01555-1.
- Balaji K, Ramasubramanian V. Hypofractionated hybrid radiotherapy techniques for synchronous bilateral breast cancer. Asian Pac J Cancer Prev. 2021;22(12):3933–3939. doi: 10.31557/APJCP.2021.22.12.3933.
- Balaji K, Yadav P, BalajiSubramanian S, et al. Hybrid volumetric modulated arc therapy for chest wall irradiation: for a good plan, get the right mixture. Phys Med. 2018;52:86–92. doi: 10.1016/j.ejmp.2018.06.641.
- Sathiyaraj P, Manikandan PS, Varatharaj C, et al. Dosimetric evaluation of hybrid and volumetric-modulated arc therapy plan for left-sided chest wall irradiation in Monaco treatment planning system. J Cancer Res Ther. 2022;18(6):1728–1732. doi: 10.4103/jcrt.JCRT_707_20.
- Aly MM, Glatting G, Jahnke L, et al. Comparison of breast simultaneous integrated boost (SIB) radiotherapy techniques. Radiat Oncol. 2015;10:139. doi: 10.1186/s13014-015-0452-2.
- Venjakob A, Oertel M, Hering DA, et al. Hybrid volumetric modulated arc therapy for hypofractionated radiotherapy of breast cancer: a treatment planning study. Strahlenther Onkol. 2021;197(4):296–307. doi: 10.1007/s00066-020-01696-8.
- Spruijt KH, Dahele M, Cuijpers JP, et al. Flattening filter free vs flattened beams for breast irradiation. Int J Radiat Oncol Biol Phys. 2013;85(2):506–513. doi: 10.1016/j.ijrobp.2012.03.040.
- Bahrainy M, Kretschmer M, Jöst V, et al. Treatment of breast cancer with simultaneous integrated boost in hybrid plan technique: influence of flattening filter-free beams. Strahlenther Onkol. 2016;192(5):333–341. doi: 10.1007/s00066-016-0960-5.
- Sakthivel V, Kadirampatti Mani G, Mani S, et al. Estimating second malignancy risk in intensity-modulated radiotherapy and volumetric-modulated arc therapy using a mechanistic radiobiological model in radiotherapy for carcinoma of left breast. J Med Phys. 2017;42(4):234–240. doi: 10.4103/jmp.JMP_89_17.
- Kumar L, Kishore V, Bhushan M, et al. Impact of acuros XB algorithm in deep-inspiration breath-hold (DIBH) respiratory techniques used for the treatment of left breast cancer. Rep Pract Oncol Radiother. 2020;25(4):507–514. doi: 10.1016/j.rpor.2020.04.011.
- Guebert A, Conroy L, Weppler S, et al. Clinical implementation of AXB from AAA for breast: plan quality and subvolume analysis. J Appl Clin Med Phys. 2018;19(3):243–250. doi: 10.1002/acm2.12329.
- Joosten A, Matzinger O, Jeanneret-Sozzi W, et al. Evaluation of organ-specific peripheral doses after 2-dimensional, 3-dimensional and hybrid intensity modulated radiation therapy for breast cancer based on Monte Carlo and convolution/superposition algorithms: implications for secondary cancer risk assessment. Radiother Oncol. 2013;106(1):33–41. doi: 10.1016/j.radonc.2012.11.012.
- Saroj DK, Yadav S, Paliwal N. Does fluence smoothing reduce the complexity of the Intensity-Modulated radiation therapy treatment plan? A dosimetric analysis. J Med Phys. 2022;47(4):336–343. doi: 10.4103/jmp.jmp_81_22.
- Fogliata A, De Rose F, Franceschini D, et al. Critical appraisal of the risk of secondary cancer induction from breast radiation therapy with volumetric modulated arc therapy relative to 3D conformal therapy. Int J Radiat Oncol Biol Phys. 2018;100(3):785–793. doi: 10.1016/j.ijrobp.2017.10.040.
- Virén T, Heikkilä J, Myllyoja K, et al. Tangential volumetric modulated arc therapy technique for left-sided breast cancer radiotherapy. Radiat Oncol. 2015;10:79. doi: 10.1186/s13014-015-0392-x.
- Yu PC, Wu CJ, Nien HH, et al. Tangent-based volumetric modulated arc therapy for advanced left breast cancer. Radiat Oncol. 2018;13(1):236. doi: 10.1186/s13014-018-1167-y.
- Karpf D, Sakka M, Metzger M, et al. Left breast irradiation with tangential intensity modulated radiotherapy (t-IMRT) versus tangential volumetric modulated arc therapy (t-VMAT): trade-offs between secondary cancer induction risk and optimal target coverage. Radiat Oncol. 2019;14(1):156. doi: 10.1186/s13014-019-1363-4.
- Engstrøm KH, Brink C, Nielsen MH, et al. Automatic treatment planning of VMAT for left-sided breast cancer with lymph nodes. Acta Oncol. 2021;60(11):1425–1431. doi: 10.1080/0284186X.2021.1983209.