Abstract
Background and purpose
Radiotherapy for vulvar carcinoma is challenging due to relatively high risk of locoregional disease recurrence, a technically challenging target, and postoperative lymphocele, and a high risk radiation sequelae. We aim to explore, if it is possible to reduce dose to normal tissue, while maintaining CTV coverage for this patient group with online adaptive radiotherapy.
Materials and methods
20 patients with vulvar carcinoma (527 fractions) were treated with online adaptation on a Varian Ethos accelerator. Setup CBCTs were acquired daily for adaptive planning. Verification CBCTs were acquired immediately prior to dose delivery. CTV dose coverage and dose to bladder and rectum were extracted from the scheduled and adapted plans as well as from adapted plans recalculated based on verification CBCTs. In addition, analysis of the decision of the adaptive procedure was performed for 17 patients (465 fractions).
Results
Mean CTV D95% and standard deviation was 98% ± 5% for the scheduled plan compared to 100.0 ± 0.3% and 100.0 ± 0.8% for the adapted plan on the setup and verification CBCT respectively. Dose to OARs varied substantially and did not show any benefit from adaption itself, however a margin reduction was implemented after the first patients treated. The adapted plan was chosen for 63.5% of the fractions and dominant reasons for not adapting were ‘no significant dosimetric gain’ (75 fractions, 14%) and ‘Medical doctor (MD) not available for treatment’ (50 fractions, 9.5%). The median adaption time was 15 min and the 25th and 75th percentile was 12 and 17 min, respectively.
Conclusion
CTVs and PTVs dose coverage were significantly improved with adaptation compared to image-guided RT. This gain was robust during the treatment time.
Introduction
Vulvar carcinoma is a rare malignancy that accounts for 0.3% of all cancers globally, with an incidence of approximately 45,000 new cases and 17,000 deaths per year (2020) [Citation1]. From 1978 to 2006, an increase of 1.6% per year has been observed for women under 60 years in Denmark, while the incidence has remained unchanged for women over 60 [Citation2].
Current treatment involves surgical resection of the primary vulvar lesion. Inguinal lymph node dissection, or sentinel lymph node staging, is performed if the primary tissue invasion exceeds 1 mm or clinically and/or radiographically suspected nodes. Patients without macrometastases, but with histological findings of micrometastases in one or more sentinel nodes are offered the same adjuvant treatment as surgical resected macrometastases, either concomitant external beam radiation therapy (EBRT) and chemotherapy or ERBT without chemotherapy [Citation3,Citation4].
Radiotherapy has a major role in curative treatment of vulvar carcinoma patients [Citation4]. National and international guidelines dictate that T1b-T2 patients should undergo radiotherapy based on the size of the surgical margin and patients with FIGO stage III disease should receive postoperative radiotherapy into the groin with the lymph node metastases [Citation5]. Postoperative radiotherapy for vulvar carcinoma is challenging due to a high burden of radiation sequelae, relatively high risk of locoregional disease recurrence and technically challenging targets (large volume concave contiguous targets) combined with a high frequency of postoperative lymphocele causing daily anatomical variations [Citation6]. The aforementioned factors are traditionally accounted for by a margin around the clinical target volume (CTV) to yield a planning target volume (PTV) to be irradiated encompassing the predicted deviations and ensure target coverage [Citation7]. This, however, causes the treatment field to include healthy tissue in the high dose area which increases the risk of side effects such as skin irritation, lymphoedema, cystitis, proctitis, urge incontinence for urine and faeces, bladder and bowel problems and sexual dysfunction. Adaptive radiation therapy (ART) can be used to account for both systematic and random complex anatomical changes, potentially reducing the required margin [Citation8,Citation9]. In ART with daily online (real-time) replanning, the dose plan is reoptimized based on the anatomy of the day in every treatment fraction [Citation10]. No other studies have been found published on this subject and comparable data is therefore limited.
It is of importance that the additional time for an online ART implementation is as short as possible, as prolonged treatment times increased the risk of intrafractional variations and could be difficult for the patient to endure [Citation11]. In addition, the monetary cost of providing care would increase if ART required substantially longer timeslots.
The risk and severity of side effects, presence of highly variable target volume and high probability of locoregional recurrence make postoperative vulvar carcinoma patients a preferred group for early experiences with online adaptive radiotherapy on a dedicated linear accelerator (Ethos, Varian Medical Systems, Palo Alto, CA) [Citation12].
Here we report on our clinical experience with the first 20 patients treated in order to explore whether online ART is relevant for these patients and if it improves CTV coverage and/or reduces dose to organs at risk (OARs). We also aim to analyze the adaptive workflow with respect to treatment time and the workload of manual editing.
Materials and methods
Patients
Daily adaptive radiotherapy became available in our department on January 22, 2021 and vulvar carcinoma patients were prioritized for the adaptive treatment slots as part of routine clinical treatment decision. After a short run-in period, the daily adaptive workflow became the standard treatment offering for vulvar carcinoma patients at our institution. Here, we report on the first 20 vulvar carcinoma patients referred for radiotherapy with daily adaptation on the Ethos accelerator. Patients were referred to postoperative radiotherapy at the primary tumor site in vulva if the surgical margins were < 3 mm, with a dose of 1.85 Gy x 27 fractions (total 49.95 Gy). If surgery was not possible on the primary site or if residual macroscopic tumor persisted after surgery the dose was 2 Gy x 32 fractions (total 64 Gy). In case of one or more groin lymphnodes with metastases patients received 1.85 Gy x 27 fractions (total 49.95 Gy). In the cases where the metastatic lymphnodes were not surgically removed the dose was 2 Gy x 32 fractions (total 64 Gy).
Patient 1 was undergoing traditional IGRT in the clinic, but transferred to adaptive radiotherapy, hence she only received the last 8 fractions of the treatment on the adaptive workflow. Another patient only received 18/32 fractions due to distant metastases discovered during treatment. Collection and reporting of the data were performed with authorization from departmental review and regulatory approval as quality assurance of the clinical procedures.
Patients 1–3 were planned with an isotropic PTV margin of 7 mm from CTV, which is standard for non-adaptive treatments in our clinic. CTVs were delineated by the oncologist based on CT images. As we observed robust adaptive plans, it was decided, starting with patient 4, to reduce PTV margins to 5 mm. This was to still account for intrafractional uncertainties, delineation uncertainties and mechanical uncertainties but let the adaptive system account for interfractional uncertainties (change in anatomy and lymphocele volume), estimated to account for 2 mm of the PTV.
shows how the dosimetric data was collected. Data includes all fractions where the physician approved the adapted structures.
Starting with patient 4, we implemented a daily questionnaire including details on the quality of the automatic contouring of OARs and CTVs, how many CBCT slices that needed to be contoured/edited as well as the reason for the choice of plan on the specific day. Number of slices that needed editing were counted by RTT’s and physician. Edits were stratified into none, mild, intermediate and major using the definition from Sibolt et al. [Citation13]. The reasons for not choosing the daily adaptive plan were categorized as 1) No physician available, 2) No dosimetric gain (in CTV coverage and OARs sparring between scheduled and adaptive plan), 3) Automatic contouring of either CTV or OARs were too poor, 4) Other and 5) Unknown. Adaption time was recorded from the first CBCT scan to the second verification CBCT scan. This interval includes evaluation and re-contour of CTVs and OAR structures, dose-calculation of scheduled and adaptive plan, plan choice and approval and QA process and could be extracted from the Ethos system, see .
Ethos planning system
Planning CT-scans were acquired with and without intra-venous contrast (Optiray 300). This ensured optimal conditions for the oncologist to contour relevant structures while having non-contrast planning scans for dose calculation and adaption to the non-contrast CBCT scans at each treatment fraction.
Treatment plans were generated semi-automatically by choosing several dosimetric goals for the CTVs, PTVs and OARs on the baseline CT scan. Plans were created with 7, 9, and 12 equidistant IMRT fields plans for adaption. The best IMRT plan, based on 12 preset dose constraints and CTV and PTV coverage for planning, was chosen as the reference plan for adaptation. The specific beam geometry (number of beams, collimator, and gantry angle) from the reference plan was used for every subsequent adapted fraction.
Adaptive online planning
The adaptive workflow is depicted schematically in . The daily adaptive procedure started with conventional patient positioning and a CBCT scan. CTVs and normal structures were transferred from the planning CT simulation to the CBCT scan of the day using a proprietary deformable registration algorithm (DIR) using hybrid matching of intensity and predefined ‘influencer structures’ [Citation14]. ‘Influencers’ is an Ethos term defining contours of organs that have a high impact on target structures in regard to position, shape and dosimetric influence. Influencer structures were reviewed and when necessary corrected manually before the remaining structures were propagated.
In this study, influencer structures were chosen to be bowel, rectum, and bladder for the first two patients but was changed to rectum and bladder only for the subsequent patients. This was due to excessive time needed to correct the automatic delineation of the bowel. CTVs and the remaining OAR structures were then deformed by the same hybrid AI algorithm as the influencer structures, accounting for the corrected and approved influencer structures. CTV and OAR structures were reviewed and approved by a specialist gynecological radiation oncologist, after editing as required.
An adapted plan was created by reoptimizing the reference plan to the anatomy of the day. The dose distribution of the reference plan was calculated on the anatomy of the day for comparison. This plan was referred to as ‘scheduled plan’. In this process the beforementioned DIR of the baseline CT Hounsfield unit map to the CBCT was used as the electron density of the day [Citation14]. The physician and physicist decided which plan to use for treatment (‘scheduled’ or ‘adaptive’) and approved it. In the absence of a specialist oncologist on the day, only the scheduled plan was allowed for treatment as this plan had been accepted by an oncologist and been through QA prior to treatment start.
A verification CBCT was acquired just prior to treatment delivery to ensure no clinically relevant changes in positioning or anatomy of the patient had occurred during the adaption. The couch position could be adjusted to account for displacements if needed. The chosen plan was verified through a QA process using Mobius3D (Varian Medical Systems, Palo Alto, CA), which include an independent MU dose calculation prior to treatment.
Offline simulation of robustness of the adapted plans
An offline validation of the quality/robustness of the adaptive plans was performed with regards to the uncertainties arising from motion during the adaptation workflow by recalculating the dose distribution from the adapted plan on the verification CBCT (293 fractions). This procedure was implemented to ensure the treatment was as secure as a traditional image-guided radiotherapy (IGRT). A post-treatment CBCT was not acquired daily due to imaging dose concerns (CTDI dose is 41.9 mGy per scan for an average adult). Plan recalculation on the verification CBCT was performed on a stand-alone Ethos emulator and required substantial manual import/export maneuvers. The dose covering the hottest 2% (D2%) and mean doses of the bladder and rectum (Dmean), and the minimum dose covering 95% of the CTV and PTV dose (D95%) dose volume histogram (DVH) data were extracted from scheduled, adapted and verification plans. For comparison, all DVH data were individually normalized per fraction to the reference plan for each patient. All DVH data were collected and compared per fraction, no dose accumulation was performed.
All comparative statistics were performed using Wilcoxon paired test, using patients as their own control. Spearman correlations were used for assessing associations. The null hypothesis was that there was no difference between the scheduled and adaptive plans, with a two-sided significance level at p = 0.05.
Results
20 patients treated between January 2021 and September 2023 were included in this study. All patients were female with a median age of 70. Patient characteristics are described in .
Table 1. Patient demographics.
Dosimetric data from 20 patients (527 fractions) were analyzed. Out of the 335 fractions, where the adapted plan was chosen, 293 fractions were offline simulated and verification plans were calculated. 42 adapted fractions were not recalculated due to import problems in the emulator. This problem appeared to occur at random except for patient 5 where the emulator couldn’t handle any of the CBCT scans due to these being acquired in two contiguous scans and subsequently merged. Qualitative data from daily questionnaires were collected for 17 patients (465 fractions).
The adaptive plan was chosen for 335 out of 527 fractions (63.5%). The proportions of adapted vs. scheduled plans and the aggregated distributions of the reasons not to adapt per patient are shown in . Dominant reasons for not adapting were ‘no significant dosimetric gain’ (75 fractions) and ‘Medical Doctor not available for treatment’ (50 fractions).
Figure 2. Distribution of chosen adapted plans for patient 1–20. Reasons for not choosing an adapted plan is also shown for each patient. Patient 1 was the pilot patient and was only treated with the adaptive workflow for the last 8 fractions. Patient 1–3 wasn't included in the daily registration and reasons are therefore unknown.
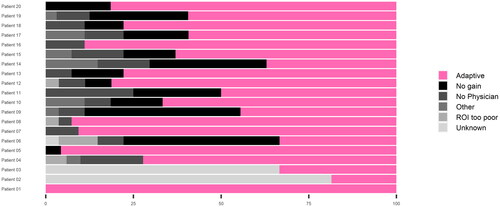
Target and OAR doses were individually normalized per fraction to the reference plan for each patient, and these doses were compared between scheduled, adapted and verification plans. Dose coverage of the CTV measured by the mean D95% was 98 ± 5% (standard deviation) for the scheduled plan and 100.0 ± 0.3% and 100.0 ± 0.8% for the adapted plan on the setup and verification CBCT respectively. This shows a significant improvement of CTV coverage with the adapted plan (p < 0.0001) which was retained at the verification step (p < 0.0001). For PTV mean D95% was 97% ± 7% for the scheduled plan and 100.0 ± 0.3% and 99.0 ± 2.0% (p < 0.0001) for the adapted plan on the setup and verification CBCT respectively. See for example of a patient with interfractional anatomical changes that could not be accounted for with IGRT using traditional PTV formalism. The mean dose to bladder increased from the scheduled to adapted plans with 106% ± 22% and 107% ± 24% (p = 0.043). This increase was higher at verification with mean 112% ± 36% (p = 0.007). The large standard deviation observed were caused by three patients having very low doses to bladder and three other patients having a small overlapping volume of PTV the bladder resulting in interfractional variation of bladder dose. Rectum mean doses were significantly higher for the scheduled plans with mean of 102% ± 27% compared to the adaptive with mean of 99% ± 24% (p = 0.0007). However, at verification it was significantly higher than scheduled plan with mean 108 ± 25% (p = 0.03), see. . The dose received by to the 2% hottest volume of bladder showed a significantly increase from scheduled to adapted plans while rectum dose was unchanged by adaptation (see Appendix for supplementary figures).
Figure 3. Example of vulvar carcinoma patient with anatomical change due to lymphocele and the direct consequence in dose coverage. A) Reference plan, B) Daily CBCT using scheduled plan, C) Daily CBCT using adapted plan. Isodose levels 95%, 90%, 80% and 50% are visualized. CTV and PTV contours are shown in pink and blue, respectively.
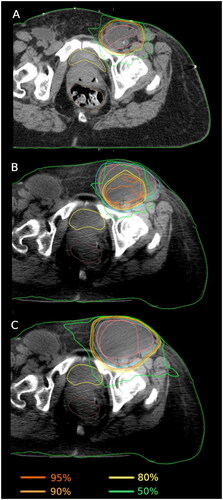
Figure 4. Normalized dose to reference plan for scheduled plans, adapted plans and verification plans for bladder (mean dose), rectum (mean dose), CTV (D95%) & PTV (D95%) respectively for patients 1–20. Dashed lines indicate reference dose. The figure excludes 2 upper outliers on bladder scheduled and adapted, 1 upper outlier on bladder verification, 1 upper outlier on rectum adapted and verification, and 2 and 3 lower outliers on CTV and PTV scheduled respectively, to improve visualization.
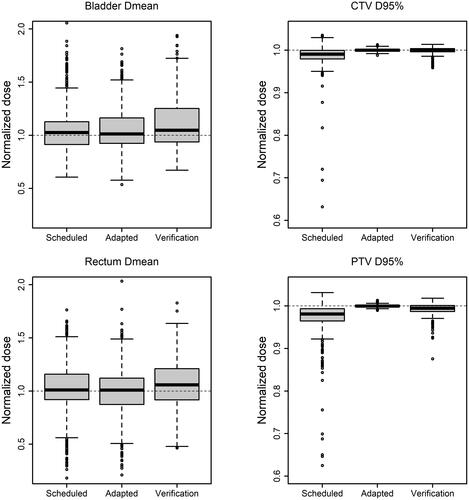
All treated plans met the predefined dose constraints.
The number of slices where OARs and CTV structures were edited varied substantially between patients (). For bladder no edits were required for 29% of fractions, minor edits for 8% of fractions and intermediate/major edits 63% of fractions. Rectum needed no edits for 46% of fractions, minor edits for 14% of fractions and intermediate/major edits for 40% of fractions. Lastly, CTV needed no edits for 46% of fractions, minor edits for 11% of fractions and intermediate/major edits for 43% of fractions.
Table 2. Average CBCT slice edits [%] (and range) of bladder, rectum, and CTV contour for patient 4-20.
For bladder, patients 5, 6, 7 and 11 only needed minor recontouring with a mean change of 3% of the slices while the rest of the patients had larger need for recontouring with a mean change of >35%. The aforementioned patients all had reproducible bladder volume and high CBCT image contrast throughout treatment, which aided automatic bladder delineation. For rectum we observed a general good delineation by the system with a mean change of <13%. The number of slices with edits needed for CTV varied a lot between the patients with a mean of 17%. In general, number of slices with edits of the machine-generated contours did not show any clear association with the proportion of fractions adapted, see. .
Figure 5. Correlation between proportion of recontoured slices and the time for adaptation for patient 4–20. Each data point represents mean number of slices needed recontouring (bladder, rectum, CTV or total) for each patient. Red lines describe linear fit.
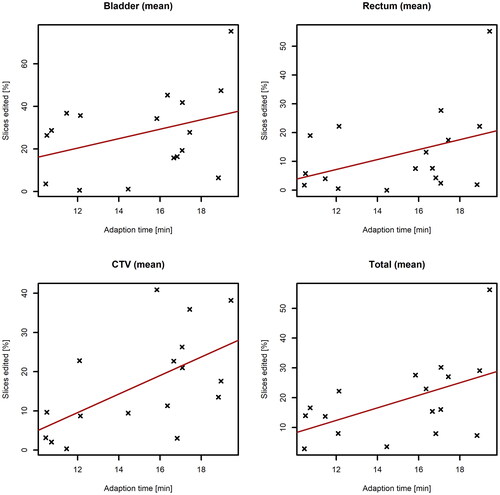
Median adaption time was 15 min and the 25th and 75th percentile was 12 and 17 min, respectively. A correlation coefficient of 0.37 and 0.43 were calculated between adaption time and number of slices edited for bladder and rectum respectively, which suggests only weak correlation, however some moderate increasing trends were observed for CTV recontoured slices and total recontoured slices with a correlation coefficient of 0.6 and 0.52 respectively, see .
Discussion
To our knowledge this is the first report of real-world data of clinical implementation of daily adaptive therapy for vulvar carcinoma. The data suggests that online adaptive radiotherapy for vulvar carcinoma is a technically viable path forward, but also that there are substantial opportunities for improvement. The main demonstrated benefit is the CTV coverage improvement, and it is reassuring that adaptive plans were shown to be robust to anatomical change during the adaption process. Together, this may facilitate even smaller margins.
Online ART in the context of vulvar carcinoma improves target coverage by adapting to large interfractional variations not covered by PTV in conventional IGRT treatment.
The quite frequent absence of MD resources to oversee the adaptive process in this study is a concern that may be institution-specific and reimbursement-related, but nevertheless indicate that the human resources needed could be a bottleneck to address in future studies.
A limitation of the robustness analysis is that we did not perform a post-treatment CBCT but analyzed stability on the verification CBCT prior to dose delivery. This choice was made as the additional imaging dose could not be justified in this routine treatment setting. Intrafractional motion is not well studied in vulvar carcinoma patients, however it has been shown that prostate intrafractional displacement tends to increase with time by Li et al. and the same trend can be assumed to exist for other pelvic area patients [Citation15]. However, it is considered unlikely that substantial changes would occur during the approximately 5 min delivery time when the dosimetric impact of anatomical changes occurring during adaption time (15 min) was as limited as observed in . We therefore conclude that for vulvar carcinoma patients the comparison of scheduled and adapted plan on the setup CBCT is a reliable representation of the delivered dose.
The adaptive plans were recalculated using the emulator and the simulation required manual recontouring of OARs and CTV which introduces some uncertainties. A physician was not present at this time-consuming procedure, and it is acknowledged that this may have a minor impact on the results. The involved physicist had access to physician approved contours which was used to produce an acceptable estimate.
No reduction in mean dose to bladder or rectum was found when comparing adaptive and scheduled plans. PTV margins were reduced by 2 mm after the third patient’s treatment, which reduced the amount of healthy tissue in the treatment volume compared to previous IGRT margins. The reduction was made based on an assessment of adequate dose coverage with the smaller margins and was confirmed by the analysis of CTV coverage and robustness presented here. Reducing margins further would increase the need for daily adaptation. At present that would require an MD to be available for every fraction to ensure accurate CTV delineation. A systematic assessment of such improvements would be recommended, for example following the IDEAL or R-IDEAL recommendations for exploration and assessment of technological innovations [Citation16–18].
Mean total treatment time, including patient positioning, image acquisition, adaption, and beam-on time was estimated 45 min, as time to position patient was not recorded. Compared with the normal IGRT treatment time of 20 min, the adaptive workflow is acceptable given the demonstrated improvement in target coverage, especially if dose to OARs can be reduced. We observed some outliers where the adaption time was up to 40 min which made the total treatment time up to an hour. Comparing with the ART prostate study by Byrne et al. [Citation19] we observe more need for edits as they showed no and minor edits were 11% and 81% of fractions to OARs (rectum, bladder, prostate and seminal vesicle) respectively and no or minor change to CTV was 91%. The ART prostate study by Sibolt et al. [Citation13] also reported less need for change of OARs and CTV. An explanation was that PTVs for vulvar carcinoma were larger than for prostate which led to larger overlap with healthy tissue, requiring increased accuracy of OAR delineation. Furthermore, 12 of the patients in this study received weekly cisplatin and antiemetic drugs which might have led to more gas and faeces in bowel and rectum due to the impact on the gastrointestinal system of these drugs, while neither of the patient groups in the Byrne or Sibolt cohorts receive concomitant chemotherapy. Appearance or changes to volumes of air, IV contrast agent or medical devices such as urinary catheters were noted to cause difficulties for the DIR and increases the need for manual editing of structures by the physicians.
The adapted plan was rejected almost 40% of the time and we observed that certain patients had a low rate of adaptation which most frequent was explained by no relevant dosimetric gain using the adapted plan compared to the scheduled plan. The second most frequent reason for not choosing an adapted plan was that no physicians were available at the accelerator during treatment. In these cases, we cannot be sure whether an adaptive plan or scheduled plan would have been preferred. The need for specialized physicians trained in daily adaption is therefore critical for complex cases, whereas simpler adaptation cases can be performed by RTTs [Citation13]. For eight fractions the automatic contouring was of such poor quality that manual recontouring was considered unfeasible due to expected adaption time and uncertainty exceeding clinical acceptable goals.
A practical challenge arises if the quality of the CBCT is insufficient for delineation. In this study, eight fractions had to be treated with the scheduled plan because the physician was unable to contour the CTV, even with the assistance of automatic contour propagation on the CBCT. Improvement of the quality of the CBCTs is therefore key to extend the use of ART.
Concluding on the efficiency of daily adaptive radiotherapy for vulvar carcinoma, it should be acknowledged that a formal health economic assessment is well beyond the scope of this paper and probably too early considering the state of development of the treatment technique. However, we believe that with gradual improvements of procedures, improvement in the CBCT quality and efficiency, online adapted treatment of vulvar carcinoma patients becomes a viable routine option in a not-too-distant future and can be expected to lead to dosimetric benefits.
It’s acknowledged that the present report is an assessment of dosimetric precision and technical feasibility of online adaptive RT for vulvar carcinoma. We have not attempted to capture clinical outcome as the study would be severely underpowered to provide informative results thereof. We did not demonstrate any benefit in terms of normal tissue complication probability. It is possible that a PTV margin reduction enabled by the improved CTV coverage with online adaptation may reduce dose to normal tissue compared to IGRT. Estimation of the clinical benefit of improved CTV coverage is too uncertain for meaningful modeling exercises.
This is, however, the first study to our knowledge of daily online adaptive radiotherapy for gynecological cancer and provides indication of feasibility and a direction for future studies of optimal use of this emerging technology.
Conclusion
Online ART has become our departmental standard offer for patients with vulvar carcinoma. There is a significant gain to CTV dose coverage with online ART workflow compared to IGRT workflow with identical PTV margins. The adaptive workflow is robust with respect to the longer total treatment time and smaller PTV margin. Further development should focus on efficacy, image quality improvements and assessment of the potential to convert the improved CTV coverage to margin reductions reducing exposure of healthy tissue.
Supplemental Material
Download MS Word (266.3 KB)Disclosure statement
The authors report institutional teaching and research contracts with Varian Medical Systems, Brainlab and ViewRay.
Data availability statement
Corresponding author can be contacted for access to data and will provide data upon reasonable request. However, this requires that the ethical and data protection regulations allow sharing of the requested data.
References
- The Global Cancer Observatory. Glocal Cancer Observatory - Vulva fact sheet Internet]. 2020. https://gco.iarc.fr/today/data/factsheets/cancers/21-Vulva-fact-sheet.pdf
- Baandrup L, Varbo A, Munk C, et al. In situ and invasive squamous cell carcinoma of the vulva in Denmark 1978-2007-a nationwide population-based study. 2011.
- Oonk MHM, Slomovitz B, Baldwin PJW, et al. Radiotherapy Versus inguinofemoral lymphadenectomy as treatment for vulvar cancer patients with micrometastases in the sentinel node: results of GROINSS-V II. J Clin Oncol. 2021;39(32):3623–3632. doi: 10.1200/JCO.21.00006.
- Oonk MHM, Planchamp F, Baldwin P, et al. European society of gynaecological oncology guidelines for the management of patients with vulvar cancer. 2017. http://links.lww.
- DGCG. Vulvar carcinoma - national guidelines (in Danish) [Internet]. 2022. http://www.dgcg.dk/index.php/guidelines/vulvacancer
- Maggino T, Landoni F, Sartori E, et al. Patterns of recurrence in patients with squamous cell carcinoma of the vulva a multicenter CTF study. 2000. 10.1002/1097-0142
- Van Herk M, Remeijer P, Rasch C, et al. The probability of correct target dosage: dose-population histograms for deriving treatment margins in radiotherapy. Int J Radiat Oncol Biol Phys. 2000;47(4):1121–1135. doi: 10.1016/s0360-3016(00)00518-6.
- Sonke J-J, Aznar M, Rasch C. Adaptive radiotherapy for anatomical changes. Semin Radiat Oncol. 2019;29(3):245–257. doi: 10.1016/j.semradonc.2019.02.007.
- Yen A, Shen C, Albuquerque K. The new kid on the block : Online adaptive radiotherapy in the treatment of gynecologic cancers. Curr Oncol. 2023;30(1):865–874. doi: 10.3390/curroncol30010066.
- Green OL, Henke LE, Hugo GD. Practical clinical workflows for online and offline adaptive radiation therapy. Semin Radiat Oncol. 2019;29(3):219–227. doi: 10.1016/j.semradonc.2019.02.004.
- Lim-Reinders S, Keller BM, Al-Ward S, et al. Critical review online adaptive radiation therapy. Int J Radiat Oncol Biol Phys. 2017;99(4):994–1003. doi: 10.1016/j.ijrobp.2017.04.023.
- Archambault Y, Boylan C, Bullock D, et al. Making ON-line adaptive radiotherapy possible using artificial intelligence AND machine learning FOR efficient daily re-planning. Med Phys Int J. 2020;8(2):77–86.
- Sibolt P, Andersson LM, Calmels L, et al. Clinical implementation of artificial intelligence-driven cone-beam computed tomography-guided online adaptive radiotherapy in the pelvic region. Phys Imaging Radiat Oncol. 2021;17:1–7. doi: 10.1016/j.phro.2020.12.004.
- Systems VM. Ethos algorithms reference guide. Varian Medical Systems. 2021.
- Li HS, Chetty IJ, Enke CA, et al. Dosimetric consequences of intrafraction prostate motion. Int J Radiat Oncol Biol Phys. 2008;71(3):801–812. doi: 10.1016/j.ijrobp.2007.10.049.
- Verkooijen HM, Kerkmeijer LGW, Fuller CD, et al. R-IDEAL: a framework for systematic clinical evaluation of technical innovations in radiation oncology. Front Oncol. 2017;7(APR):59. doi: 10.3389/fonc.2017.00059.
- McCulloch P, Altman DG, Campbell WB, et al. No surgical innovation without evaluation: the IDEAL recommendations. Lancet. 2009;374(9695):1105–1112. doi: 10.1016/S0140-6736(09)61116-8.
- Ergina PL, Barkun JS, McCulloch P, IDEAL Group, et al. IDEAL framework for surgical innovation 2: observational studies in the exploration and assessment stages. BMJ. 2013;346(jun18 3):f3011–f3011. doi: 10.1136/bmj.f3011.
- Byrne M, Archibald-Heeren B, Hu Y, et al. Varian ethos online adaptive radiotherapy for prostate cancer: early results of contouring accuracy, treatment plan quality, and treatment time. J Appl Clin Med Phys. 2021;23(1):e13479.