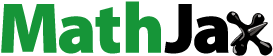
Background
Deep inspiration breath-hold (DIBH) for radiotherapy (RT) can mitigate breathing motion [Citation1–3], improve cone-beam computed tomography (CBCT) image quality [Citation4] and create a favorable anatomy regarding dose to organs at risk (OARs) [Citation1,Citation5–8]. Initial concerns about patient compliance [Citation9] have been reconsidered, as both spirometry based and voluntary, optical tracking based DIBH technique are tolerated by most patients [Citation3,Citation7,Citation10,Citation11]. However, reproducibility and dosimetric benefit vary between patients, so a method for individual assessment is warranted. It is desirable to supplement geometrical/anatomical evaluation of the reproducibility with an estimate of the dosimetric effect of an observed variation in DIBH level.
We provide a method to evaluate the dosimetric effect of intra-fractional variation in DIBH levels on a patient- and treatment plan specific level.
Material and methods
Patients and treatment planning
Four patients with locally advanced non-small cell lung cancer were selected from the INHALE trial (clinicaltrials.gov ID: NCT02540499), where patients referred for RT of locally advanced non-small cell lung cancer (LA-NSCLC) were planned in both free breathing (FB) and DIBH [Citation3]; DIBH plans used in the current work. Scans acquired for treatment planning were a 4-dimensional CT (4DCT) followed by three consecutive DIBH CTs. DIBHs were audio-visually guided using the RPM system version 1.7 (Varian Medical Systems, Palo Alto, CA).
The DIBH scan with the most average tumor position was chosen for planning. Population-based PTV margins were applied, taking amongst other the image match strategy into account and whether there patients had mediastinal or peripheral targets or both [Citation10]. Volumetric modulated arc therapy (VMAT) plans were created in the Eclipse treatment planning system (TPS) (Varian Medical Systems), using the AcurosXB algorithm, version 15.5.11 (calculating dose to media), with a prescribed mean dose of 2 Gy × 33 fractions (5 fractions/week) to the planning target volume (PTV). Each plan consisted of two partial arcs. Dose constraints on target and OARs were according to national guidelines [Citation12]. For further imaging and treatment planning details, see Josipovic et al. [Citation3].
The patients selected represented different scenarios in terms of anatomic tumor position and observed DIBH reproducibility. Patient #1–2 represented worst case scenarios with large variations between consecutive DIBH CTs. Patients #3–4 represented best case scenarios with different target constitution (). In the trial, the most advantageous plan regarding OAR dose and reproducibility of DIBH anatomy was used for treatment.
Figure 1. Primary tumor position of the four patients, for which the suggested method was tested (top row images) and summary of lesion and PTV characteristics (below). PTV margins were population based [Citation10], however, individually modified for patient #1 due to large tumor deviation between DIBHs.
![Figure 1. Primary tumor position of the four patients, for which the suggested method was tested (top row images) and summary of lesion and PTV characteristics (below). PTV margins were population based [Citation10], however, individually modified for patient #1 due to large tumor deviation between DIBHs.](/cms/asset/d8df4f35-c8d7-4b11-b4d4-1fbc06a7156b/ionc_a_2259084_f0001_c.jpg)
Intra-fractional robustness analysis
The intra-fractional plan robustness of DIBH plans was evaluated using the Eclipse Scripting Application Programming Interface (ESAPI), version 15.5. The treatment plans were manipulated by modification of the ‘ControlPoints’ properties, followed by automated volumetric dose calculation.
The script, available in the supplementary material, included the following steps:
(1) The two half arcs of the DIBH treatment plan were divided into four sub-arcs i, by evenly distributing each arc’s ControlPoints. This should represent sub-arcs of approximate duration of one DIBH (typically ∼20 s).
(2) The four sub-arcs were assigned randomly to one of the three DIBH CT scans for each of the 33 fractions (Supplementary Table S1).
(3) Each sub-arc i was pasted onto each DIBH scan j, resulting in twelve possible sub-arc/DIBH scan combinations. The monitor units MUi,j for all combinations were then calculated to reflect the proportion of fractions where DIBH scan j was randomly assigned to sub-arc i (in step (2)):
(4) All sub-arcs belonging to the same DIBH scan j were combined into one sub-plan.
(5) Volumetric dose was calculated for the three sub-plans.
A simulated sum dose was created by deformable image registration and dose summation of the three sub-plans, as described below. The whole workflow is illustrated in .
Deformable registration and dose summation
To estimate the total delivered dose, the DIBH scans were deformably registered to each other. The DIBH scan chosen for treatment planning in the clinical evaluation was used as reference. The deformable registration, as well as a dose summation, was performed using the Dose Summation Navigator in Velocity (Varian Medical Systems) version 4.0. A Deformable Multi Pass registration was carried out, with a volume of interest (VOI) including the entire body contour in the trans-axial directions and covering the entire lungs (with a 1–2 cm margin) in the cranio-caudal direction. The results of the deformations were visually inspected and approved by an oncologist (GFP) and two physicists (KH and MJ).
The composite dose volume – from now on referred to as the simulated sum dose – was imported back into Eclipse. Here, dose metrics for target coverage (V95% for PTV-N and PTV-T) and dose to OARs (Dmax for Body, Esophagus and Spinal cord, mean dose and V20Gy for Lungs minus GTV and V25Gy for Heart) were compared between the simulated sum dose and the original plan. Note that we chose to evaluate target coverage for the PTV and not for the CTV, due to the presence of intra-breath-hold motion to also be taken into consideration [Citation13]. The Dmax metric was defined as the minimum dose to the hottest 0.1 cm3 of a structure.
Quality assurance of the workflow
Script uncertainty analysis
Before using the script on consecutive DIBH scans, it was tested on three copies of the same scan, to assess any dosimetric difference between a plan with two arcs and a plan with four sub-arcs added together. At the same time, this served as a quality control of the script.
Intra-observer uncertainty in deformable registration
To estimate the dosimetric uncertainty related to the deformable registration process, the deformable registration was performed six times for one patient (pt #1). One observer (KH) repeated the registration using different settings. The Dose Summation Navigator in Velocity, limited to only one deformable registration, was used in three of the six registrations, while manual deformable registration was performed for the other three cases, using 2–3 sequential deformable registrations each with different VOIs (Supplementary Table S2). The proposed method’s robustness to these different deformation/registration approaches was quantified by a median and range for the dose-volume metrics for the six repeated deformations of patient #1.
Results
Script performance and deformable registration uncertainty
The analysis of the script uncertainty revealed negligible effect when dividing the original plan into four sub-arcs and adding them up. All target and OAR dose metric differences were within ± 0.3%-points and ± 0.3 Gy (Supplementary Figure S2).
The intra-observer uncertainty analysis performed for patient #1 resulted in a variation in the simulated sum dose distribution between [−1.6, 3.4] %-points for PTV-T, [−2.0, 1.4] %-points for PTV-N and within ± 0.7%-points and ± 0.4 Gy for all OAR metrics (Supplementary Table S3).
Dosimetric intra-fractional robustness
For patient #1, the simulated sum dose resulted in compromised target doses, both for PTV-T (original plan V95% = 97.0% vs. simulated sum dose V95% = 72.2%) and PTV-N (original plan V95% = 97.1% vs. simulated sum dose V95% = 90.2%). The corresponding differences for the OARs were < (0.7 Gy and 0.9%-points). In contrast, patients #2–4 had small dosimetric differences – within 1.0%-points and 0.7 Gy for both the targets and OARs ().
Figure 3. Dosimetric comparison of the original plan (x-axis) and the simulated sum dose (y-axis), representing an estimate of the dosimetric effect of anatomical differences between consecutive DIBHs. The shaded areas represent the values where planning constraints for OARs are not met. Each patient is represented by his/her own symbol. If planning constraints are met in the original plan, but not in the simulated sum dose, symbols are marked as red. Note that patient #4 has a T-site lesion only and is therefore not presented on the PTV-N plot.
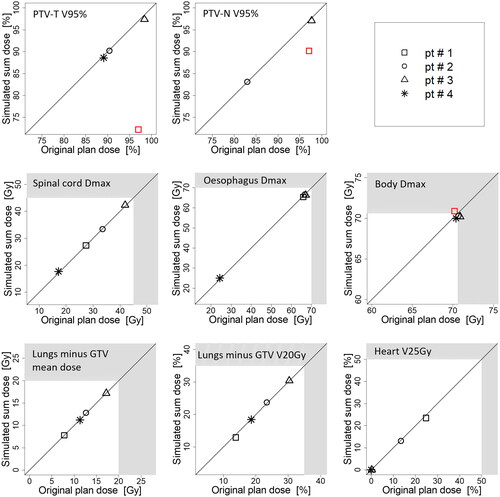
The deformable registration in Velocity was deemed successful in all four example cases, by visual inspection by three observers. Small deviations in the order of one slice separation (2 mm) were accepted due to uncertainties connected to the partial volume effect (Supplementary Figure S3).
Discussion
Modern RT techniques enable high dose conformality, but as the conformality increases, the intra- and inter-fractional robustness may eventually be compromised. The intra-fractional breathing motion in lung cancer RT can be mitigated by using a DIBH strategy [Citation1–3], but the robustness of this strategy is conditioned by the reproducibility of consecutive DIBHs. For a whole treatment course of 33 fractions, a DIBH patient would, to our clinical experience, typically hold his/her breath 33 × 4 = 132 times, i.e. four times per fraction or two times per half arc. A method for dosimetric evaluation based on consecutive DIBHs for individual patients and plans is presented in this study.
RayStation is to our knowledge currently the only commercial TPS that can handle multiple sets of CT scans for robust planning of photon RT [Citation14], however, not including other aspects of our method such as the division of beams. Other TPSs have tools for robustness evaluation but these are simple and typically only simulate translational shifts. Including multiple CT sets in both optimization and evaluation of dose to moving tumors in FB has been investigated previously [Citation15,Citation16]. The proposed method is meant to aid the respiratory motion management strategy decision, i.e. whether a patient should be treated with DIBH or not. This evaluation can be performed without the use of any complex software (the script, available as supplementary material, can easily be adapted to use within the Eclipse TPS), and is, therefore, possible to implement effortlessly in the clinic after appropriate testing. A drawback of the proposed method is that it is designed for use with the Eclipse TPS only. The method can likely be used with other TPS’s as well with proper translation of technical details and alternative scripting languages. Using the method does involve some manual steps, and further automation would be useful to reduce the labor intensity in busy clinics. A strength of the method is the random assignment of DIBHs to different parts of the treatment, i.e. simulating the effect of multiple DIBHs being required to complete each treatment fraction. A conceptually simpler method could be to paste the plan on the DIBH scan with the largest deviation of target position. However, this requires additional target and OAR contouring and would likely over-estimate the plan degradation. While such an approach may appear as a ‘safe choice,’ it does not recognize that each DIBH differing from the planning DIBH has its own specific areas of under/overdosage. A consequential abandoning of DIBH treatment could sometimes be the suboptimal compromise. Instead, increased margins, intensified DIBH training or, if available, gated MR-linac treatment could be alternative choices. We believe the proposed method gives the most relevant estimation of impact of inter-DIBH variations on the delivered dose. If DIBH plans are dosimetrically superior, it can be argued that the added dose of acquiring two additional pre-treatment CT scans to ensure plan robustness is acceptable according to the ALARA principle. A subsequent evolutionary step could be the generation of a robust plan by reoptimizing the dose in the most critical regions detected by the method.
The accuracy of our method depends on the reliability of the deformable registration. Discrepancies of up to 5%-points were observed with different settings. Therefore, we recommend that clinical action based on very small deviations is avoided. It is also important to note that the method has a random element to it, and that the suggested method prerequisites that the three repeated DIBH CT scans acquired at treatment planning are representative of the variation in DIBH throughout the full treatment course. This may need verification through repetition of DIBH CT for challenging cases.
In three of the four patient cases tested (patients #2–4), the clinical decision was to treat in DIBH – a decision that was based on geometrical measures of difference between consecutive DIBHs, along with dosimetric superiority of the DIBH plan for OAR doses [Citation3]. It is reassuring that the decision based on geometrical measures was supported when dosimetric evaluation was added. This is not to say that the dosimetric evaluation is redundant, as this additionally enables setting clinically relevant thresholds related to tumor control probability and normal tissue toxicity. The current dataset is, however, too small to evaluate the sensitivity and specificity of any of the methods.
To conclude, a method was presented to evaluate the intra-fractional robustness of DIBH RT treatment plans, based on repeated DIBH scans and dose summation. We believe the clinical implementation of such an evaluation method could provide more individualized selection criteria for robust delivery of DIBH RT of lung cancer, as well as other anatomical sites with intra-fractional motion uncertainties.
Supplemental Material
Download MS Word (1.3 MB)Disclosure statement
All authors report departmental research agreements with Varian Medical Systems and Viewray Inc.
Data availability statement
Data not available due to ethical/legal restrictions.
Additional information
Funding
References
- Barnes EA, Murray BR, Robinson DM, et al. Dosimetric evaluation of lung tumor immobilization using breath hold at deep inspiration. Int J Radiat Oncol Biol Phys. 2001;50(4):1091–1098.
- Ottosson W, Rahma F, Sjöström D, et al. The advantage of deep-inspiration breath-hold and cone-beam CT based soft-tissue registration for locally advanced lung cancer radiotherapy. Radiother Oncol. 2016;119(3):432–437. doi: 10.1016/j.radonc.2016.03.012.
- Josipovic M, Aznar MC, Thomsen JB, et al. Deep inspiration breath hold in locally advanced lung cancer radiotherapy: validation of intrafractional geometric uncertainties in the INHALE trial. Br J Radiol. 2019;92(1104):20190569. doi: 10.1259/bjr.20190569.
- Josipovic M, Persson GF, Bangsgaard JP, et al. Deep inspiration breath-hold radiotherapy for lung cancer: impact on image quality and registration uncertainty in cone beam CT image guidance. Br J Radiol. 2016;89:20160544.
- Josipovic M, Persson GF, Håkansson K, et al. Deep inspiration breath hold radiotherapy for locally advanced lung cancer: comparison of different treatment techniques on target coverage, lung dose and treatment delivery time. Acta Oncol (Madr). 2013;52(7):1582–1586.
- Ottosson W, Sibolt P, Larsen C, et al. Monte carlo calculations support organ sparing in deep-inspiration breath-hold intensity-modulated radiotherapy for locally advanced lung cancer. Radiother Oncol. 2015;117(1):55–63. doi: 10.1016/j.radonc.2015.08.032.
- Giraud P, Morvan E, Claude L, et al. Respiratory gating techniques for optimization of lung cancer radiotherapy. J Thorac Oncol. 2011;6(12):2058–2068. Decdoi: 10.1097/JTO.0b013e3182307ec2.
- Marchand V, Zefkili S, Desrousseaux J, et al. Dosimetric comparison of free-breathing and deep inspiration breath-hold radiotherapy for lung cancer. Strahlenther Onkol. 2012;188(7):582–589. doi: 10.1007/s00066-012-0129-9.
- Rosenzweig KE, Hanley J, Mah D, et al. The deep inspiration breath-hold technique in the treatment of inoperable non-small-cell lung cancer. Int J Radiat Oncol Biol Phys. 2000;48(1):81–87. doi: 10.1016/s0360-3016(00)00583-6.
- Josipovic M, Persson GF, Dueck J, et al. Geometric uncertainties in voluntary deep inspiration breath hold radiotherapy for locally advanced lung cancer. Radiother Oncol. 2016;118(3):510–514. doi: 10.1016/j.radonc.2015.11.004.
- Boda-Heggemann J, Knopf AC, Simeonova-Chergou A, et al. Deep inspiration breath HolddBased radiation therapy: a clinical review radiation. Int J Radiat Oncol Biol Phys. 2016;94(3):478–492. doi: 10.1016/j.ijrobp.2015.11.049.
- Danish Oncology Lung Group. DOLG’s radioterapigruppes rekommendationer for udførelse af strålebehandling ved lunge kræft (in Danish). Aarhus, Denmark: Danish Oncology Lung Group; 2017.
- Scherman Rydhög J, Riisgaard De Blanck S, Josipovic M, et al. Target position uncertainty during visually guided deep-inspiration breath-hold radiotherapy in locally advanced lung cancer. Radiother Oncol. 2017;123(1):78–84. doi: 10.1016/j.radonc.2017.02.003.
- Unkelbach J, Alber M, Bangert M, et al. Robust radiotherapy planning. Phys Med Biol. 2018;63(22):22TR02. doi: 10.1088/1361-6560/aae659.
- Bokrantz R, Fredriksson A. Scenario-based radiation therapy margins for patient setup, organ motion, and particle range uncertainty. Phys Med Biol. 2017;62(4):1342–1357. doi: 10.1088/1361-6560/aa524d.
- McCann C, Purdie T, Hope A, et al. Lung sparing and dose escalation in a robust-inspired IMRT planning method for lung radiotherapy that accounts for intrafraction motion. Med Phys. 2013;40(6):061705. doi: 10.1118/1.4805101.