Abstract
Background
Volumetric Modulated Arc Therapy (VMAT) offers better conformity, homogeneity and sparing of the heart and ipsilateral lung for locoregional radiotherapy in left-sided breast cancer compared to three-dimensional conformal radiotherapy (3D-CRT). However, conventional coplanar VMAT (cVMAT) can result in higher doses to the normal tissue on the contralateral side. This study investigates a non-coplanar VMAT-technique (ncVMAT) to mitigate this issue.
Material and methods
CT series of 20 left sided breast cancer patients were included for planning of locoregional breast radiotherapy including internal mammary nodes (IMN). Three treatment plans; 3D-CRT, cVMAT and ncVMAT, were generated for each patient with a prescription dose of 40.05 Gy in 15 fractions. Both VMAT-techniques consisted of a single arc in the axial plane, while ncVMAT included an additional arc in the sagittal plane. All plans were optimized to cover the clinical target volume (CTV) by 38.05 Gy for the breast and 36.05 Gy for lymph nodes, with as low as possible dose to organs at risk.
Results
Full CTV coverage was achieved for all plans. Both cVMAT and ncVMAT delivered more conformal and homogeneous target doses than 3D-CRT. Doses to the heart and ipsilateral lung were significantly lower with ncVMAT compared to both cVMAT and 3D-CRT. ncVMAT reduced doses to both the contralateral breast and lung compared to cVMAT and achieved levels similar to 3D-CRT for the contralateral breast and moderately higher doses for the contralateral lung. Delivery of high doses (>30 Gy) to the contralateral side was completely avoided with ncVMAT, contrary to the results for cVMAT and 3D-CRT.
Conclusion
ncVMAT reduced doses to the heart and ipsilateral lung as compared to both cVMAT and 3D-CRT. All contralateral dose metrics were reduced with the novel ncVMAT technique compared to cVMAT, and the mean contralateral breast doses were similar to 3D-CRT.
Background
Postoperative adjuvant radiotherapy significantly reduces the risk of local, regional and distant failure and improves overall survival for patients with early breast cancer [Citation1]. Treatment of locoregional lymph nodes provides a survival benefit for node positive patients [Citation2–4] and the inclusion of the internal mammary nodes (IMN) in the radiation fields has been shown to improve overall survival [Citation5–9].
Internal mammary node irradiation is technically challenging because of the close proximity to the heart. Increased dose to the heart has been correlated with increased cardiac mortality in older trials [Citation10], especially when combined with potentially cardiotoxic systemic treatments [Citation11,Citation12]. Furthermore, radiotherapy for breast cancer is significantly associated with increased risk of secondary malignancies decades after exposure, overall and in organs adjacent to the treatment field [Citation13,Citation14]. Balancing the pros and cons by including the IMN is thus difficult, as indicated by the great variation in recommendations between countries [Citation15].
The traditional treatment technique for locoregional radiotherapy in early breast cancer involves tangential photon fields covering the residual breast/chest wall and IMN, combined with anterior and posterior photon fields covering the periclavicular and axillary lymph nodes. Deep inspiration breath hold (DIBH) techniques, combined with CT-based 3D-Conformal Radiotherapy (3D-CRT), has significantly reduced radiation doses to the heart and the ipsilateral lung [Citation16]. Still, more advanced techniques, such as Volumetric Modulated Arc Therapy (VMAT) or in some cases proton beam therapy (PBT), may be required for difficult cases in order to achieve both adequate target coverage and acceptable heart and lung doses [Citation17]. However, the associated redistribution of doses with the use of VMAT may introduce new dosimetric issues usually not seen with 3D-CRT [Citation18–20]. Thus, there is a need for development of improved VMAT-approaches.
The aim of the present treatment planning study is to compare a novel non-coplanar VMAT-technique (ncVMAT) with conventional coplanar VMAT (cVMAT) and 3D-CRT. The hypothesis is that ncVMAT with an additional arc in the sagittal plane (gantry 50–0°, couch 90°) will reduce the dose to the contralateral side, the heart and the ipsilateral lung in patients receiving locoregional radiotherapy for early breast cancer, including IMN.
Materials and methods
Patient data and structures
CT series from 20 left-sided early breast cancer patients treated with breast conserving surgery followed by locoregional radiotherapy between July 2018 and July 2019 at Stavanger University Hospital, Norway, were included in this retrospective treatment planning study. The patients were positioned in a supine position with bilateral arm abduction above the head using an immobilization device (BreastBoard LX, MacroMedics BV, The Netherlands). The CT series were obtained during DIBH with a CT slice thickness of 2 mm. All participants provided written informed consent, and the project was approved by the regional committees for Medical research Ethics in Central Norway (ref 39660).
Clinical target volumes (CTVs) of the breast (CTVp_Breast), supraclavicular/axillary lymph nodes (CTVn) and the internal mammary lymph nodes including IC3 (CTVn_IMN) were delineated by one radiation oncologist, based on the ESTRO consensus guidelines [Citation21]. The corresponding planning target volumes (PTVs) were created with isotropic margins of 5 mm, except for PTVp_Breast, where the PTV was cropped 5 mm below skin surface. The various CTVs and PTVs were combined into single union structures, CTV_Union and PTV_Union, respectively.
Organs at Risk (OARs) included the heart, left anterior descending coronary artery (A-LAD), lungs, contralateral breast, the ipsilateral brachial plexus, thyroid gland, trachea, oesophagus, ipsilateral humeral head and spinal cord. The A-LAD was delineated in the anterior interventricular groove down to the apex of the heart. To monitor the dose to the contralateral side of the body midline, an additional structure, Contralateral Body, was generated.
Contralateral breast, ipsilateral brachial plexus and the thyroid gland were delineated by the radiation oncologist. The remaining OARs were delineated by a radiotherapy technologist and verified by the oncologist. The Eclipse treatment planning system, version 13.6 (Varian Medical Systems, Palo Alto, CA, USA) was used for all structure delineations.
Treatment planning
For each patient, three treatment plans were generated: 3D-CRT, cVMAT and ncVMAT. The dose matrix for all plans was 2x2x2 mm. A prescription dose of 40.05 Gy in 15 fractions to the median of each of the CTVs was pursued for all plan types. To avoid hot-spots this aim had to be relaxed with 3D-CRT for CTVn and CTVn_IMN.
All treatment plans were generated and optimised using RayStation v12A (RaySearch Laboratories AB, Stockholm, Sweden), with an Elekta Versa HD linac model (Elekta Solutions AB, Stockholm, Sweden) and the Collapsed Cone (CC) calculation algorithm.
3D-CRT-plans were developed according to clinical procedures at Stavanger University Hospital involving wide opposing 6 MV tangential fields covering the breast and IMN, half-beam matched with anterior and posterior fields, 6 MV and 15 MV respectively, covering the axillary and supraclavicular nodes. Occasionally low weighted 15 MV tangential fields were added in the main field directions to improve dose coverage interiorly. All main fields were conformed with 5 mm multileaf collimators (MLC) to cover the PTV with a 5 mm margin initially and subsequently blocked towards critical organs if necessary. Tangential fields were opened into air superficially to account for breast swelling or deformations. The isocenter was individually positioned inside the CTV at the transition between mammary tissue and supraclavicular lymph nodes.
VMAT-optimization was based on the clinical cVMAT-protocol at St. Olavs Hospital. Both cVMAT and ncVMAT included a single 6 MV arc in the transversal plane with gantry rotating from 178° to 280° with a 5° collimator angle. In addition, ncVMAT included a supplementary 6 MV arc in the sagittal plane using a coach rotation of 90° with a collimator angle of 85° and gantry rotation from 50° (inferiorly) to 0° (, upper panel). The sliding window sequencing, with 2 degrees spacing between the control points, was used for both VMAT techniques. The total monitor units (MU) were restricted to 750 for both VMAT-techniques; for ncVMAT, this restriction was split into 500 MU and 250 MU for the transversal and sagittal arc, respectively. The maximum delivery time was set to 150 s for the transversal arc and 60 s for the sagittal arc.
Figure 1. The left, Middle and right panels show beam arrangements and dose distributions from 3D-CRT, cVMAT and ncVMAT, respectively. The horizontal yellow lines at the sagittal view indicate the location of the transversal slices and vice versa for the yellow line at the transversal views. Dose color map is shown at the bottom left.
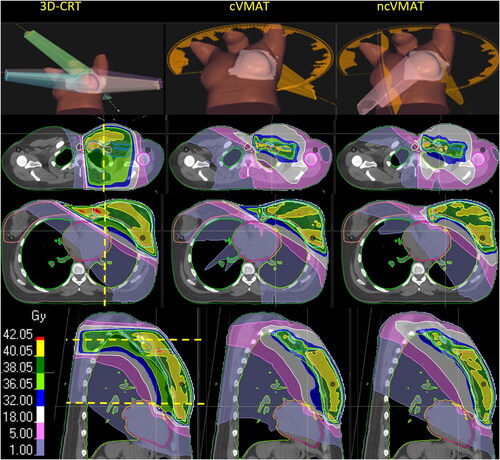
All plans were optimized to cover the CTVp_Breast by 38.05 Gy (95% dose level) and CTVn and CTVn_IMN by 36.05 Gy (90% dose level) and as low as possible doses to OARs, following the priorities in . While these priorities were achieved through careful selection of gantry angles and MLC-modifications for the 3D-CRT technique, VMAT-plans relied on adjustments of optimization objectives throughout the planning process. VMAT-plans were generated through an in-house developed automated process with no human input during the 30–40 min of plan development (Supplemental A). None of the plans were used for treatment of patients.
Table 1. Ranked priorities for the treatment planning.
Robustness of VMAT-plans with regard to breast swelling and set-up uncertainties was assured through a novel use of the robust planning feature in RayStation, which facilitates parallel optimization of several CT series simultaneously to take into account potential patient deformations. A breast swelling 15 mm anteriorly and laterally was simulated in this additional CT series (Supplemental B).
Statistics and reporting
Paired Wilcoxon test was used for statistical analysis of the differences between the plans. Differences were considered statistically significant for p < 0.05. Population based dose volume histograms (DVHs) were generated as an average of all 20 cases with 0.5 Gy spacing, starting at 0.5 Gy.
The definition of dose-volume-parameters was as follows:
Dx: the dose at volume covering x% of a structure
Dxcm3: the dose at volume covering x cm3 of a structure
Dmax: the dose at 0.01 cm3 of a structure (D0.01cm3)
Dmean: average dose
Vy: the volume of a structure covered by y% of the target dose
VyGy: the volume of a structure covered by a specified dose level
CI: Conformity Index = the absolute volume of PTV covered by the 90%-isodose divided by the total volume covered by the 90%-isodose
HI: Homogeneity Index = D95/D5
Results
illustrates the general dose distribution characteristics for a representative patient while population-based average DVHs are found in .
Figure 2. Population-based mean DVHs (N = 20) for the target volumes and OARs.
Treatment technique is indicated by blue solid line (ncVMAT), red dotted line (cVMAT) and black dashed line (3D-CRT).
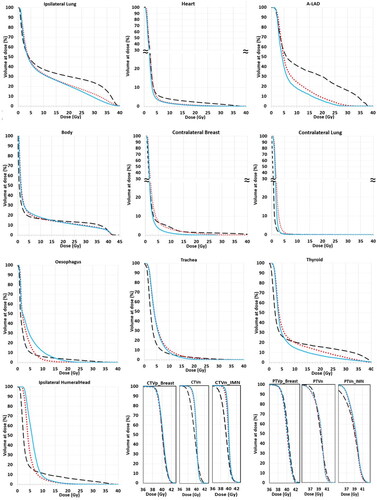
Target volumes
The dose metrics for the target volumes are presented in . All techniques provided adequate coverage of the CTVs. The cVMAT and ncVMAT plans displayed similar dose metrics for all targets, as is also illustrated by the almost inseparable target DVHs for cVMAT and ncVMAT (). For CTVp_Breast, also the 3D-CRT provided very similar dose metrics and DVHs as the VMAT plans. However, 3D-CRT achieved inferior D98, Dmean and HI for the lymph node CTVs compared to both VMAT techniques. The median PTVp_Breast coverage (D98) was almost identical for all plan types at 38.1 Gy. The VMAT plans also achieved a very consistent D98 for both lymph node PTVs at 36.1 ± 0.1 Gy for all cases. 3D-CRT delivered the highest D98 at 36.9 Gy for PTVn, but the lowest D98 for PTVn_IMN with a median of 33.7 Gy. Compared to 3D-CRT, VMAT significantly improved dose conformity, with CI values of 0.74 and 0.78 for cVMAT and ncVMAT, respectively, and 0.51 for 3D-CRT.
Table 2. Dose metrics for target volumes. Median pairwise differences between 3D-CRT (A), cVMAT (B) and ncVMAT (C) are indicated in bold script when significant (p < 0.05).
Organs at risk
The dose metrics for OARs are presented in and .
Table 3. Dose metrics for OARs. Median pairwise differences between 3D-CRT (A), cVMAT (B) and ncVMAT (C) are indicated in bold script when significant (p < 0.05).
The median mean heart dose (MHD) was significantly reduced with the VMAT techniques; from 2.5 Gy with 3D-CRT to 2.0 Gy with cVMAT and 1.8 Gy with ncVMAT. Both VMAT techniques also significantly reduced the D2 compared to 3D-CRT for the heart. Dmax for the A-LAD was also significantly lower for ncVMAT at 11.2 Gy as compared to 33.7 Gy and 23.1 Gy for 3D-CRT and cVMAT, respectively.
The mean dose and V17Gy to the ipsilateral lung were significantly reduced by more than 20% with both VMAT techniques as compared to 3D-CRT. Both VMAT-techniques also provided a significant reduction of V5Gy compared to 3D-CRT, especially for ncVMAT.
The lowest mean dose of 0.6 Gy to the contralateral lung was achieved with the 3D-CRT technique, but again the ncVMAT technique was superior to cVMAT with a median mean dose of 0.8 Gy compared to 1.1 Gy for cVMAT. V5Gy was below 1% for all techniques.
The mean dose to the contralateral breast was similar for ncVMAT and 3D-CRT, while cVMAT displayed 0.5 Gy higher dose than ncVMAT. The lowest Dmax was seen with ncVMAT.
The Dmax for the oesophagus, trachea, thyroid gland and ipsilateral humeral head, were reduced with both VMAT techniques compared to 3D-CRT. No large differences were seen for the mean doses to these organs and they were considered clinically acceptable for all plan types. Doses to spinal cord and brachial plexus were within tolerances for all plans.
Mean dose and maximum dose to the total body were significantly lower with both VMAT-techniques as compared to 3D-CRT and the volume receiving near therapeutic doses, represented by V32Gy, was significantly lower for ncVMAT at 2084cm3 compared to 2958 cm3 for 3D-CRT and 2204 cm3 for cVMAT. However, the 3D-CRT plans displayed lower volume fractions receiving doses below 10–15 Gy than for VMAT (). The volume receiving above 30 Gy on the contralateral side of the midline (Contralateral Body) was reduced to zero for ncVMAT, from 74.8 cm3 and 38.9 cm3 for 3D-CRT and cVMAT, respectively.
Robust analysis
The D98-values for PTVp_Breast remained above 36 Gy for all cases and all three techniques through the simulation of either set-up errors or left breast swelling (). The higher Body maximum dose (D2cm3) in 3-CRT plans originally, increased even more than for cVMAT and ncVMAT in both robustness scenarios. The ipsilateral lung demonstrated similar dose metric increases with anterior/lateral set-up errors for VMAT and 3D-CRT plans, while for the heart the dose metrics were less affected by the set-up errors with VMAT.
Discussion
The present study provides a plan comparison between a novel non-coplanar VMAT technique, conventional coplanar VMAT and 3D-CRT for the treatment of left-sided locoregional breast cancer. With the ncVMAT technique, we were able to demonstrate a reduction of the doses to the ipsilateral lung and heart compared to both cVMAT and in particular to 3D-CRT. We also found an improvement of all contralateral dose metrics with ncVMAT as compared to cVMAT. VMAT improved all dose metrics to the lymph node CTVs as compared to 3D-CRT.
The primary aim was to cover the CTV by 38.05 Gy (95% dose) for the breast and 36.05 Gy (90% dose) for lymph nodes. A reasonable PTV-coverage for 3D-CRT was secured for most cases by a 5 mm field margin to the PTV, while the VMAT-plans consistently achieved target coverage through the automated planning process. In order to fulfill dose constraints for heart and lungs, it was necessary to reduce the field margins in some cases with 3D-CRT, which frequently resulted in underdosing. This is in accordance with results from similar studies using 3D-CRT techniques [Citation17,Citation22]. However, as the IMN-region is regarded the second most important part of the target volume after the primary site [Citation9,Citation23], a reduction of set-up margins towards the penumbra of the parallel tangential fields of the 3D-CRT technique may be critical for the treatment outcome.
The use of an expanded CT series for robust VMAT-planning was proven satisfactory, similar to what has been demonstrated by others when using a virtual bolus in the optimization stage [Citation24]. However, this latter approach may suffer from dose deterioration due to the necessary final recalculation of doses without the bolus. The present robust co-optimization of both the original and expanded CT series (virtual bolus) eliminates this step and secures optimal dose distribution in the original CT.
Evaluating the risk of late effects after locoregional breast radiotherapy with VMAT techniques is challenging, since most long term data are based on conventional 3D-CRT. While DVHs for 3D-CRT mostly show a sector of the OARs receiving target dose and another sector spared altogether, VMAT usually results in more varying DVH-shapes which might lead to different late effect outcomes, despite similarity in single dose metrics [Citation25]. Therefore, even though the VMAT plans in this study were aimed at keeping the dose deposition within traditionally irradiated sectors, the correlation to established recommendations for mean dose and volume covered by specific isodoses may not be valid.
A linear correlation between the mean heart dose (MHD) and ischemic heart disease has been demonstrated [Citation11,Citation26]. However, the D2 and V25Gy (for normo fractionation) have demonstrated better normal tissue complication probability (NTCP)-predictions than the MHD [Citation27]. The Quantec recommendations [Citation28] indicate that V25Gy < 10% corresponds to a NTCP risk below 1% for partial irradiation of the heart. By lowering both the MHD and the volume of the heart receiving high doses, the present study shows that VMAT has the potential to reduce heart complications as compared to 3D-CRT. With the ncVMAT technique the Dmax to the A-LAD was also lower, thus providing a potential to reduce radiation-induced stenosis [Citation29].
The risk of radiation induced pneumonitis is correlated with both mean dose to the lung and the volume receiving various dose levels [Citation30,Citation31]. A safe mean lung dose (MLD) with respect to pneumonitis has not been established and an increased risk of radiation pneumonitis of 12% for every Gy increase in MLD is reported [Citation31]. The reduction of the mean dose to the ipsilateral lung in the present study from 12.6 Gy for 3D-CRT to 9.7 Gy (cVMAT) to 9.2 Gy (ncVMAT) should thus be expected to reduce the risk of radiation pneumonitis. For ncVMAT, a reduction in irradiated lung volume was found for all dose levels above 2.5 Gy in the ipsilateral lung DVH, as compared to 3D-CRT.
Radiotherapy-induced second malignancy is another severe and potentially lethal side effect after breast radiotherapy [Citation32]. In particular, lung cancers have a potential to counteract much of the survival-gain from the treatment, especially for smokers [Citation12]. While the mean lung dose has been shown to correlate with the excess rate of second lung cancer [Citation33], other response characteristics for higher dose levels are discussed [Citation34,Citation35]. Thus, despite the significantly reduced total lung doses with ncVMAT, the absolute benefit in terms of risk of second lung cancer is still uncertain. There is also an increased risk of cancer in the oesophagus, the contralateral breast [Citation36] as well as bone and soft tissue sarcomas [Citation36] after breast radiotherapy. The low mean dose to both the contralateral breast, oesophagus and total body observed in the present VMAT-techniques, is thus reassuring.
With 3D-CRT the dose to trachea, oesophagus, thyroid gland and humeral head are usually controlled by moderate angling and modifications of the field borders of the AP-PA fields. For the VMAT plans in the present study the doses to these organs were controlled by low weighted mean dose objectives in the optimization process. This resulted in lower maximum doses for the VMAT-plans compared to 3D-CRT, while mean doses were kept at a clinically acceptable level similar to 3D-CRT. Leaving these secondary OARs completely out of the optimization process may however increase the dose and thereby potentially increase the risk for shoulder morbidity [Citation37], hypothyroidism [Citation36], acute oesophagitis [Citation38] and second oesophagus cancer [Citation14].
Compared to 3D-CRT, both VMAT techniques provided a reduction of the high dose volume (V32Gy) to the body, which potentially might reduce the risk of fatigue [Citation39]. Despite some reduced low dose areas with 3D-CRT, the integral dose was significantly higher for 3D-CRT than for both VMAT-techniques. Nevertheless, low dose spill is still a reason for some skepticism towards IMRT/VMAT in breast cancer treatment [Citation20] and 3D-CRT is therefore often the recommended technique [Citation23,Citation40]. By adding a non-coplanar arc and controlling the VMAT with proper optimization objectives as in the present study, the doses to the contralateral lung and breast are approaching the level achieved with 3D-CRT. Another important dosimetric aspect of ncVMAT, is the ability to eliminate the high dose volume (V30Gy) on the contralateral side of the midline (Contralateral Body), which remains significant both in 3D-CRT (74.8 cm3) and cVMAT (38.9 cm3). Breast cancer survivors have an elevated risk of developing a new primary contralateral breast cancer after radiation exposure [Citation13] and avoiding high doses to the contralateral side will simplify a subsequent treatment session to the contralateral breast, if needed.
Recently, another study has investigated a technique similar to ncVMAT [Citation41]. A dosimetric improvement by adding a beam rotating in the sagittal plane was found there as well. However, due to different optimization priorities, and maybe different delineation protocols for the breast and IMN, that study reported unacceptable high MHD at 11.16 and 9.22 Gy for the coplanar and non-coplanar VMAT, respectively. Thus, the additional sagittal arc is only one of many features that can be included in the treatment planning toolbox. An automated optimization process that pushes all OAR doses to their individually achievable minimum, as in the present study, is another. A recent study compared automated cVMAT-plans to 3D-CRT for a similar patient cohort [Citation22]. With VMAT, they reported a similar benefit for the heart doses as in our study, while ipsilateral lung doses were the same (V20Gy) or even higher (Dmean) compared to 3D-CRT. This is in contrast to a 20% reduction in these ipsilateral lung parameters in our implementation. They also reported a 4-fold higher mean dose to the ipsilateral humeral head with cVMAT compared to 3D-CRT. These differences underscore the importance of including appropriate optimization objectives for all relevant OAR-structures, even some traditionally not considered with 3D-CRT-plans, when VMAT is implemented.
Treatment planning studies are prone to operator bias and it is challenging to design studies that avoid this altogether [Citation42]. The automated approach mitigate this issue and secure that both VMAT-techniques are subject to an equally thorough optimization process. A similar automation strategy is unavailable for 3D-CRT. To secure an adequate plan quality, these plans were instead developed at a clinic with long traditions using 3D-CRT both as standard in the clinical routine and for inclusion in clinical studies [Citation23].
Although ncVMAT provide a general reduction in dose to OARs as compared to cVMAT, some challenges with the technique must be addressed. Most important is that the total time for the patient on the treatment coach would increase 2–3 min to allow for both couch repositioning and time to deliver the additional arc. Furthermore, tight tolerances for the mechanic couch isocenter is a necessity. The choice of gantry angles from 50 to 0 degrees for the additional sagittal arc, was not tested on the actual patient cases in this retrospective study, but chosen from preliminary investigations on test subjects. Prospective investigations with modified fixation and optimized gantry angles together with long-term patient follow-up will be necessary to reveal the true potential of this novel ncVMAT technique.
Conclusion
In this study, a non-coplanar VMAT technique outperforms both traditional cVMAT and, in particular, 3D-CRT in terms of doses to the ipsilateral lung and heart. While all plans provided adequate CTV coverage, superior coverage of PTVn_IMN was achieved with the VMAT techniques. With ncVMAT, the mean doses to the contralateral breast and lung were reduced compared to cVMAT, approaching dose levels found with 3D-CRT. Furthermore, ncVMAT eliminated the deposition of high dose (>30 Gy) across the midline, enabling safe treatment in case of a contralateral breast cancer in the future. Overall, ncVMAT offers significant benefits over 3D-CRT and traditional cVMAT in patients with an indication for locoregional radiotherapy for early breast cancer, including IMN.
Abbreviations | ||
IMN | = | internal mammary nodes |
VMAT | = | volumetric modulated arc therapy |
cVMAT | = | coplanar VMAT |
ncVMAT | = | non-coplanar VMAT |
3D-CRT | = | 3-dimensional conformal radiotherapy |
CTV | = | clinical target volume |
PTV | = | planning target volume |
PBT | = | proton beam therapy |
DIBH | = | deep inspiration breath hold |
A-LAD | = | coronary artery-left anterior descending |
OAR | = | organ at risk |
CI | = | conformity index |
HI | = | homogeneity index |
DVH | = | dose volume histogram |
MHD | = | mean heart dose |
MLC | = | multileaf collimator |
MLD | = | mean lung dose |
NTCP | = | normal tissue complication probability |
Supplemental Material
Download MS Word (1 MB)Supplemental Material
Download MS Word (321 KB)Data availability statement
The data that support the findings of this study are available from the corresponding author, [JF], upon request.
Disclosure statement
No potential conflict of interest was reported by the authors.
Additional information
Funding
References
- Darby S, McGale P, Correa C, Early Breast Cancer Trialists’ Collaborative G., et al. Effect of radiotherapy after breast-conserving surgery on 10-year recurrence and 15-year breast cancer death: meta-analysis of individual patient data for 10,801 women in 17 randomised trials. Lancet. 2011;378(9804):1707–1716.
- Overgaard M, Hansen PS, Overgaard J, et al. Postoperative radiotherapy in high-risk premenopausal women with breast cancer who receive adjuvant chemotherapy. Danish breast cancer cooperative group 82b trial. N Engl J Med. 1997;337(14):949–955. doi: 10.1056/NEJM199710023371401.
- Ragaz J, Jackson SM, Le N, et al. Adjuvant radiotherapy and chemotherapy in node-positive premenopausal women with breast cancer. N Engl J Med. 1997;337(14):956–962. doi: 10.1056/NEJM199710023371402.
- McGale P, Taylor C, Correa C, et al. Effect of radiotherapy after mastectomy and axillary surgery on 10-year recurrence and 20-year breast cancer mortality: meta-analysis of individual patient data for 8135 women in 22 randomised trials. Lancet. 2014;383(9935):2127–2135. doi: 10.1016/S0140-6736(14)60488-8.
- Thorsen LB, Offersen BV, Danø H, et al. DBCG-IMN: a Population-Based cohort study on the effect of internal mammary node irradiation in early node-positive breast cancer. J Clin Oncol. 2016;34(4):314–320. doi: 10.1200/JCO.2015.63.6456.
- Thorsen LB, Thomsen MS, Berg M, et al. CT-planned internal mammary node radiotherapy in the DBCG-IMN study: benefit versus potentially harmful effects. Acta Oncol. 2014;53(8):1027–1034. doi: 10.3109/0284186X.2014.925579.
- Kim J, Chang JS, Choi SH, et al. Radiotherapy for initial clinically positive internal mammary nodes in breast cancer. Radiat Oncol J. 2019;37(2):91–100. doi: 10.3857/roj.2018.00451.
- Borm KJ, Simonetto C, Kundrát P, et al. Toxicity of internal mammary irradiation in breast cancer. Are concerns still justified in times of modern treatment techniques? Acta Oncol. 2020;59(10):1201–1209. doi: 10.1080/0284186X.2020.1787509.
- Thorsen LBJ, Overgaard J, Matthiessen LW, et al. Internal mammary node irradiation in patients with node-positive early breast cancer: fifteen-year results from the danish breast cancer group internal mammary node study. J Clin Oncol. 2022;40(36):4198–4206. doi: 10.1200/JCO.22.00044.
- Rutqvist LE, Lax I, Fornander T, et al. Cardiovascular mortality in a randomized trial of adjuvant radiation therapy versus surgery alone in primary breast cancer. Int J Radiat Oncol Biol Phys. 1992;22(5):887–896. doi: 10.1016/0360-3016(92)90784-f.
- Darby SC, Ewertz M, McGale P, et al. Risk of ischemic heart disease in women after radiotherapy for breast cancer. N Engl J Med. 2013;368(11):987–998. doi: 10.1056/NEJMoa1209825.
- Taylor C, Correa C, Duane FK, et al. Estimating the risks of breast cancer radiotherapy: evidence from modern radiation doses to the lungs and heart and from previous randomized trials. J Clin Oncol. 2017;35(15):1641–1649. doi: 10.1200/JCO.2016.72.0722.
- Stovall M, Smith SA, Langholz BM, et al. Dose to the contralateral breast from radiotherapy and risk of second primary breast cancer in the WECARE study. Int J Radiat Oncol Biol Phys. 2008;72(4):1021–1030. doi: 10.1016/j.ijrobp.2008.02.040.
- Grantzau T, Overgaard J. Risk of second non-breast cancer after radiotherapy for breast cancer: a systematic review and meta-analysis of 762,468 patients. Radiother Oncol. 2015;114(1):56–65. doi: 10.1016/j.radonc.2014.10.004.
- Duane FK, McGale P, Teoh S, et al. International variation in criteria for internal mammary chain radiotherapy. Clin Oncol (R Coll Radiol). 2019;31(7):453–461. doi: 10.1016/j.clon.2019.04.007.
- Hjelstuen MH, Mjaaland I, Vikström J, et al. Radiation during deep inspiration allows loco-regional treatment of left breast and axillary-, supraclavicular- and internal mammary lymph nodes without compromising target coverage or dose restrictions to organs at risk. Acta Oncol. 2012;51(3):333–344. doi: 10.3109/0284186X.2011.618510.
- Ranger A, Dunlop A, Hutchinson K, et al. A dosimetric comparison of breast radiotherapy techniques to treat locoregional lymph nodes including the internal mammary chain. Clin Oncol (R Coll Radiol). 2018;30(6):346–353. doi: 10.1016/j.clon.2018.01.017.
- Ashby O, Bridge P. Late effects arising from volumetric modulated arc therapy to the breast: a systematic review. Radiography (Lond). 2021;27(2):650–653. doi: 10.1016/j.radi.2020.08.003.
- Dumane VA, Bakst R, Green S. Dose to organs in the supraclavicular region when covering the internal mammary nodes (IMNs) in breast cancer patients: a comparison of volumetric modulated arc therapy (VMAT) versus 3D and VMAT. PLoS One. 2018;13(10):e0205770. doi: 10.1371/journal.pone.0205770.
- Alongi F, Giaj-Levra N, Fiorentino A, et al. Low-dose bath with volumetric modulated arc therapy in breast cancer: much ado about nothing? Tumori. 2016;102(4):335–336. doi: 10.5301/tj.5000516.
- Offersen BV, Boersma LJ, Kirkove C, et al. ESTRO consensus guideline on target volume delineation for elective radiation therapy of early stage breast cancer, version 1.1. Radiother Oncol. 2016;118(1):205–208. doi: 10.1016/j.radonc.2015.12.027.
- Engstrøm KH, Brink C, Nielsen MH, et al. Automatic treatment planning of VMAT for left-sided breast cancer with lymph nodes. Acta Oncol. 2021;29:1–7.
- Offersen BV. The Skagen Trial 1 2015. Available from: https://www.dbcg.dk/PDF/SKAGEN_Trial1_protokol_ver_1.0_sept_2015_28.09.2015.pdf.
- Rossi M, Virén T, Heikkilä J, et al. The robustness of VMAT radiotherapy for breast cancer with tissue deformations. Med Dosim. 2021;46(1):86–93. doi: 10.1016/j.meddos.2020.09.005.
- Prunaretty J, Bourgier C, Gourgou S, et al. Different meaning of the mean heart dose between 3D-CRT and IMRT for breast cancer radiotherapy. Front Oncol. 2022;12:1066915. doi: 10.3389/fonc.2022.1066915.
- Laugaard Lorenzen E, Christian Rehammar J, Jensen M-B, et al. Radiation-induced risk of ischemic heart disease following breast cancer radiotherapy in Denmark, 1977–2005. Radiother Oncol. 2020;152:103–110. doi: 10.1016/j.radonc.2020.08.007.
- Moiseenko V, Einck J, Murphy J, et al. Clinical evaluation of QUANTEC guidelines to predict the risk of cardiac mortality in breast cancer patients. Acta Oncol. 2016;55(12):1506–1510. doi: 10.1080/0284186X.2016.1234067.
- Gagliardi G, Constine LS, Moiseenko V, et al. Radiation dose-volume effects in the heart. Int J Radiat Oncol Biol Phys. 2010;76(3 Suppl.):S77–S85. doi: 10.1016/j.ijrobp.2009.04.093.
- Wennstig AK, Garmo H, Isacsson U, et al. The relationship between radiation doses to coronary arteries and location of coronary stenosis requiring intervention in breast cancer survivors. Radiat Oncol. 2019;14(1):40. doi: 10.1186/s13014-019-1242-z.
- Marks LB, Bentzen SM, Deasy JO, et al. Radiation dose–volume effects in the lung. Int J Radiat Oncol Biol Phys. 2010;76(3 Suppl):S70–S76. doi: 10.1016/j.ijrobp.2009.06.091.
- Karlsen J, Tandstad T, Sowa P, et al. Pneumonitis and fibrosis after breast cancer radiotherapy: occurrence and treatment-related predictors. Acta Oncol. 2021;60(12):1651–1658. Decdoi: 10.1080/0284186X.2021.1976828.
- Grantzau T, Overgaard J. Risk of second non-breast cancer among patients treated with and without postoperative radiotherapy for primary breast cancer: a systematic review and meta-analysis of population-based studies including 522,739 patients. Radiother Oncol. 2016;121(3):402–413. doi: 10.1016/j.radonc.2016.08.017.
- Grantzau T, Thomsen MS, Væth M, et al. Risk of second primary lung cancer in women after radiotherapy for breast cancer. Radiother Oncol. 2014;111(3):366–373. doi: 10.1016/j.radonc.2014.05.004.
- Abo-Madyan Y, Aziz MH, Aly MM, et al. Second cancer risk after 3D-CRT, IMRT and VMAT for breast cancer. Radiother Oncol. 2014;110(3):471–476. doi: 10.1016/j.radonc.2013.12.002.
- Schneider U. Mechanistic model of radiation-induced cancer after fractionated radiotherapy using the linear-quadratic formula. Med Phys. 2009;36(4):1138–1143. doi: 10.1118/1.3089792.
- Choi SH, Chang JS, Byun HK, et al. Risk of hypothyroidism in women after radiation therapy for breast cancer. Int J Radiat Oncol Biol Phys. 2021;110(2):462–472. doi: 10.1016/j.ijrobp.2020.12.047.
- Johansen S, Fosså K, Nesvold IL, et al. Arm and shoulder morbidity following surgery and radiotherapy for breast cancer. Acta Oncol. 2014;53(4):521–529. doi: 10.3109/0284186X.2014.880512.
- Rose J, Rodrigues G, Yaremko B, et al. Systematic review of dose-volume parameters in the prediction of esophagitis in thoracic radiotherapy. Radiother Oncol. 2009;91(3):282–287. doi: 10.1016/j.radonc.2008.09.010.
- Reidunsdatter RJ, Rannestad T, Frengen J, et al. Early effects of contemporary breast radiation on health-related quality of life – predictors of radiotherapy-related fatigue. Acta Oncol. 2011;50(8):1175–1182. doi: 10.3109/0284186X.2011.604345.
- National action program with guidelines for diagnosis, treatment, and follow-up of patients with breast cancer. Norwegian, 2021. Available from: https://nbcgblog.files.wordpress.com/2021/03/nasjonalt-handlingsprogram-for-pasienter-med-brystkreft-01.03.2021-16-utgave.pdf.
- Xu Y, Ma P, Hu Z, et al. Non-coplanar volumetric modulated arc therapy for locoregional radiotherapy of left-sided breast cancer including internal mammary nodes. Radiol Oncol. 2021;55(4):499–507. doi: 10.2478/raon-2021-0045.
- Hansen CR, Crijns W, Hussein M, et al. Radiotherapy treatment plannINg study guidelines (RATING): a framework for setting up and reporting on scientific treatment planning studies. Radiother Oncol. 2020;153:67–78. doi: 10.1016/j.radonc.2020.09.033.