Abstract
Objective
To estimate the cost-effectiveness of single-use hydrophilic-coated intermittent catheters (HCICs) versus single-use uncoated intermittent catheters (UICs) for urinary catheterization.
Methods
The evaluation took a UK national health service (NHS) perspective. The population of interest were people using intermittent catheters, with either a spinal cord injury or multiple sclerosis. A Markov model was developed that estimated costs and clinical evidence over the lifetime of a hypothetical cohort and applied health-related quality-of-life estimates. Model inputs were sourced from published evidence, including a network meta-analysis to inform the treatment effect (reduction in catheter-associated urinary tract infections [CAUTIs]), and were supported by expert opinion. The model outputs included per-patient lifetime costs, quality-adjusted life years (QALYs), and the incremental cost effectiveness ratio (ICER). Event counts were also produced.
Results
Using HCICs instead of UICs could prevent seven CAUTI events per patient over a lifetime horizon (1.8 requiring secondary care). Overall, lifetime use of HCICs is £3,183 more expensive than use of UICs per patient. However, for these additional costs, 0.55 QALYs are gained. The ICER is £5,755 per additional QALY gained. Key drivers of the model results were identified and subject to sensitivity analyses. The results were found to be robust to parameter uncertainty.
Conclusion
HCICs are likely to be a cost-effective alternative to UICs, a result driven by substantial reductions in the number of CAUTIs. Their adoption across clinical practice could avoid a substantial number of infections, freeing up resources in the NHS and reducing antibiotic use in urinary catheter users.
PLAIN LANGUAGE SUMMARY
A new economic model was developed from a United Kingdom National Health Service perspective, to explore whether hydrophilic-coated intermittent catheters would be “worth” introducing for intermittent catheter users with either a spinal cord injury or multiple sclerosis. More specifically, costs were analyzed alongside clinical evidence and health-related quality-of-life data to investigate whether hydrophilic-coated intermittent catheters would offer a notable health benefit when compared with uncoated intermittent catheters for the assessed population, whilst keeping costs to the National Health Service sufficiently low. Model inputs were sourced from published evidence where possible, and experts were consulted otherwise. The results showed that, whilst lifetime use of hydrophilic-coated intermittent catheters is £3,183 more expensive than use of uncoated intermittent catheters per patient, the health benefit with hydrophilic-coated intermittent catheters offsets these costs, by definition a cost-effective result. This means that hydrophilic-coated intermittent catheters are likely to be a cost-effective alternative to uncoated intermittent catheters. Their adoption across clinical practice could avoid a substantial number of infections, thereby freeing up healthcare resources in the National Health Service and reducing antibiotic use in urinary catheter users.
Introduction
Injuries and conditions affecting the spinal cord can lead to impaired bladder function and, therefore, urinary retention, whereby an individual is unable to completely empty urine from the bladder. Two examples of such conditions are spinal cord injuries (SCI) and multiple sclerosis (MS), which are estimated to affect approximately 50,000 and 130,000 people in the UK, respectivelyCitation1,Citation2. Urinary retention is a common issue for these patient groups and can have serious consequences if left untreated, such as urinary tract infections (UTIs), urinary incontinence, bladder damage, and kidney damage, which can lead to kidney failure in some individualsCitation3.
Methods used to assist emptying of the bladder for those who are unable to void naturally are transurethral or suprapubic indwelling catheters and intermittent catheters (ICs)Citation3. According to the EAU guidelines and the National Institute of HealthCitation3,Citation4, intermittent catheterization, whenever possibly aseptic, is the gold standard. “Clean” intermittent catheterization, popularized by Lapides et al.Citation5, was found to be the safest method for bladder emptying, with the lowest potential for urological complications in patients with a SCICitation6. Despite this, UTIs are still a common complication seen in catheter usage, in a retrospective study with a 12-year follow-up, chronic or recurrent urinary tract infections were present in 42% of patients performing clean intermittent self-catheterizationCitation7.
Catheter-associated UTIs (henceforth referred to as CAUTIs) have a negative impact on health-related quality-of-life (HRQoL); they are at best a repeated inconvenience for patients, who already suffer with the more direct consequences of their injury or condition, and at worst they are life-threateningCitation8. The treatment of these infections places a substantial cost burden on the health system and contributes to the global issue of antibiotic resistanceCitation9. For these reasons, the prevention of CAUTIs has been highlighted as a national priority in the UKCitation10.
Intermittent catheters with hydrophilic coating are seen to reduce some of the risk factors for CAUTIs, particularly by reducing urethral trauma from repeated use of these productsCitation11. Hydrophilic coatings for intermittent catheters are often based on a hydrophilic polymer called polyvinylpyrrolidone, PVP. The PVP is dissolved in a solvent and coated onto the catheters by a dip coating or a spray coating process. After a thorough drying, the PVP coating is crosslinked and bonded to the catheter surface, to avoid removal during use and in some case during storage in an aqueous swelling medium.
The final coating properties such as the friction force or coefficient of friction (COF) for hydrophilic coatings are extremely low as the crosslinked PVP gel contains a certain or a sufficient amount of water, giving the coating a long dry-out time, which is very important for the user as a sufficient time for handling is needed before the coating starts to dry out and makes the insertion less smooth.
Furthermore, the catheter coating stays hydrated and lubricious during use (inserted in the urethra) as the aqueous swelling medium may contain an osmolality increasing compound (NaCl) making the catheter easy to move after emptying the bladder. The withdrawal frictional force is therefore lower than that of a conventional uncoated intermittent catheter (UIC)Citation12. Furthermore, hydrophilic-coated intermittent catheters (HCICs) may lower the risk of long-term urethral complications that could otherwise be exacerbated by repeatedly inserting a UIC. In the UK, both HCICs and UICs are indicated for single use onlyCitation13.
In order to optimize the care pathway for IC users, decision-makers require information on the cost-effectiveness of various treatment options. Cost-effectiveness analyses involve an assessment of an intervention’s health benefits against its costs, when compared with relevant alternatives. HCICs are more expensive products than UICs, and so a cost-effectiveness analysis can conclude whether they are likely to be good value for money for healthcare providers, such as the National Health Service (NHS) in the UK. However, existing cost-effectiveness evaluations of HCICs have been inconsistent in their findings, or their conclusions have been invalidated by the inclusion of inappropriate comparators, such as multiple use catheters (off-label use, not recommended by NICE)Citation14–16.
The purpose of this evaluation was to investigate the cost-effectiveness of single-use HCICs when compared with single-use UICs in a population of people with SCI or MS receiving healthcare in the UK.
Methods
No ethical approval was required for this analysis.
Description of the economic model
A model was developed in Microsoft Excel to estimate the cost-effectiveness of HCICs. Health economic models allow evidence to be synthesized from a variety of sources and extrapolated over an appropriate time horizon. They also provide a framework for testing various parameter scenarios and their influence on the cost-effectiveness of an intervention, to provide a more holistic answer to a decision problem.
The cohort modelled were IC users suffering from either SCI or MS; a 50:50 split was assumed in the base case. Single-use HCICs were compared with single-use UICs. A UK perspective was taken, focusing on the NHS and personal social services (PSS) as is standard for such evaluations. Therefore, the model adopted a cost-effectiveness threshold of £20,000 per quality-adjusted life year (QALY) gained, and applied a discount rate of 3.5% to both costs and QALYs, in line with the NICE methods and process manualCitation17.
The model used a Markov state-transition structure to predict the outcomes of a hypothetical cohort. Over time, the cohort moved from one health state (Alive) to another (Dead) based on the probability of mortality, which varied as the cohort aged. The modelling of CAUTIs, the main clinical driver of the model, was achieved by applying incidence rates to the living proportion of the cohort with a treatment effect applied for HCICs. This model structure is illustrated in .
CAUTIs occurring in the model were stratified into six categories, according to degree of severity. The categories were based upon clinical guidance and were intended to capture any meaningful differences in resource use or HRQoL outcomes. There was no impact of catheter type on the breakdown of CAUTIs; that is, one type of catheter was not assumed to cause more severe infections than another.
The model utilized annual cycles, meaning that movements between health states (Alive or Dead) only occurred at the end of each year. Half-cycle correction was applied to account for the fact that, on average, deaths would occur at the midpoint of each cycle.
For each cycle, mean costs and HRQoL utilities were assigned to the living cohort. The proportion of the cohort in the Dead state did not accrue costs and QALYs. The model utilized a lifetime horizon (meaning that the cohort were followed until death), to ensure that all differences in costs and benefits were captured in the evaluation. Total costs and QALYs were calculated for HCIC users and UIC users, and these were summarized in an incremental cost-effectiveness ratio (ICER) amongst other statistics.
Key model inputs
The characteristics of the modelled cohort were averages of the demographics for SCI and MS populationsCitation18,Citation19. These characteristics were used to ensure that mortality and HRQoL estimates in the model were age- and gender-appropriate. Background mortality was sourced from Office of National Statistics life tables for England and WalesCitation20. An elevated risk of mortality was applied for the SCI/MS population using standardized mortality ratios, which were applied multiplicatively to the general population estimatesCitation21,Citation22. In addition, the model captured the risk of death from infection, via the number of CAUTIs predicted to be fatal. See for general model inputs.
Table 1. General model inputs.
The baseline annual rate of CAUTIs was calculated as a weighted average of estimates from four randomized controlled trialsCitation23–26, as this was considered a more robust estimate than any one of the individual results. This rate was used to predict the number of CAUTIs that occurred per cycle in UIC users. A relative rate of infection – sourced from a network meta-analysisCitation27 – captured the expected reduction in number of CAUTIs when HCICs were used as an alternative, which was applied multiplicatively to the baseline rate to give the annual rate of CAUTIs in HCIC users. Proportions informally elicited from the clinical co-authors were assigned to estimate the outcome severity of these CAUTIs, with costs and HRQoL impacts assigned accordingly. See for infection-related inputs.
Table 2. Inputs predicting infection outcomes (independent of catheter type).
EQ-5D utilities were used to estimate health-related quality-of-life (HRQoL). Baseline utility for the general population was based on UK population normsCitation34. Decrements were applied for the SCI and MS catheterizing populations to capture the associated fall in utility, calculated by subtracting population-specific utilities from population norms for the general population. For people with an SCI who use catheters, a utility of 0.831 was used, sourced from a previous evaluation in this populationCitation14. For people with MS, a utility of 0.518 was sourced for somebody with an expanded disability status scale (EDSS) score of 5, as this was believed to be averagely representative of an MS patient with bladder impairmentCitation29. It was assumed that this accounts for catheter usage and so no further utility decrement was applied for catheterization. From these population-specific utilities came utility decrements of 0.08 and 0.33, respectively. An additional utility decrement of 0.017, approximately equivalent to a 2% reduction in HRQoL, was applied to UIC users to account for HRQoL differences beyond those attributable to UTIs, such as improved comfort and convenienceCitation30.
Each CAUTI event was associated with a utility decrement and duration of decrement, which were multiplied to provide an estimate for the QALY impact of each event. The durations should reflect the length of recovery for each event. To reflect the HRQoL impact of infections with severe complications, a utility decrement for sepsis was sourced from a NICE submissionCitation33. The durations of these severe infections are not evidenced for a UK setting so were sourced from Italian DRG data, and were considered by a UK clinical expert (and co-author) to be plausible in an NHS settingCitation27. For the less severe infections, utility decrements and the corresponding durations were modeler assumptions validated by clinical opinion.
Unit costs were combined with the frequency of catheter use to produce an average cost of catheterization for each treatment arm. The NHS Drug Tariff (November 2021) was used to source unit costs for commonly used HCIC catheter brands, and a simple average product cost was calculated for each sexCitation28. The average unit cost of a UIC, also derived from the NHS drug tariff, was sourced from an existing evaluation, and was inflated to match the cost year of this evaluation (2020/2021). One sachet of lubricant was costed for every UIC used. Five catheters were costed for per day, based on the EAUN recommendation of four to six catheters per dayCitation4.
For infections treated in primary care, a micro-costing approach was taken whereby the costs of each element of resource use (antibiotics, GP time, testing) were sourced and used to calculate an overall cost for each type of infection. NICE guidance (2018) was used to determine appropriate antibiotic dosage for the treatment of a CAUTICitation35. Antibiotics were costed using the drugs and pharmaceutical electronic market information tool (eMIT) or the British National Formulary (BNF)Citation36,Citation37.
An equal split in use of Nitrofurantoin, Trimethoprim and Amoxicillin was assumed for first-line antibiotic treatment in line with NICE guidanceCitation35; the same approach was taken with Pivmecillinam, Cefalexin, Co-amoxiclav, and Ciprofloxacin for second-line treatment. Additional healthcare-related costs associated with CAUTI treatment in primary care were identified, and clinical input was sought to obtain the corresponding resource use frequencies. These frequencies were multiplied with the corresponding unit costs and summed to produce a total cost of treating each type of infection. The inputs used in this micro-costing approach are provided in the Supplementary material.
For infections treated in secondary care, composite costs of treatment (incorporating all elements of resource use) were sourced for use in the model. The NHS reference costs were used where these could be aligned with the description of outcomes in the modelCitation31. The upper estimate of a literature-sourced cost of treating a catheter-associated blood stream infection (CABSI) was assumed reflective of the cost of treating an infection with severe complications (non-fatal), and was inflated to match the cost year of this evaluation (2020/2021)Citation32. For the cost of a fatal infection, an assumption was made to maintain proportionality with the HRQoL outcomes. The duration of an infection of this severity in the model is 1.76* the duration of an infection with severe complications, not leading to death. To maintain proportionality in the model, the cost applied is assumed to be 1.76* the cost of an infection with severe complications, not leading to death.
Sensitivity analyses
Deterministic sensitivity analysis (DSA) was performed to account for parameter uncertainty, with the outcomes presented in the form of a Tornado plot. Univariate DSA demonstrates the impact of changes in individual parameters, whilst all others remain fixed, giving an indication as to which parameters have the greatest impact on the model results.
Probabilistic sensitivity analysis (PSA) was also performed to account for multivariate uncertainty in the model. In PSA, parameter values are generated from a pre-specified distribution based on type of variable, and the results are summarized to provide an estimate of the probability of cost-effectiveness. The PSA in this evaluation was run with 10,000 iterations. A cost-effectiveness plane and a cost-effectiveness acceptability curve were generated.
Results
The results of the base case evaluation, as estimated by the mean of all probabilistic iterations, are summarized in . The results reflect that HCICs are £3,183 more expensive than UICs overall but are associated with 0.55 more QALYs over a patient’s lifetime (both results have been discounted). The average survival is greater for the HCIC arm, a result that is driven by a reduction in infection-related fatalities.
Table 3. Results of the evaluation.
The estimated ICER is £5,755 per QALY gained. This result is comfortably below the cost-effectiveness threshold in the UK of £20,000 per additional QALY gained, meaning the model finds HCICs to be cost-effective when compared with UICs in this population.
Net monetary benefit (NMB) represents the net benefit of HCICs compared with UICs, when all benefits are converted into monetary units. It was calculated on the basis of a willingness-to-pay of £20,000 per additional QALY gained. The NMB is £7,878, its positive value reflecting the favorable cost-effectiveness result.
HCICs were cost-effective in 89% of simulated cases. The graphical PSA outputs (cost-effectiveness plane and cost-effectiveness acceptability curve, both in ) illustrate and summarize the results of the 10,000 iterations.
Figure 2. Top panel ∼ Cost-effectiveness plane. Bottom panel ∼ Cost-effectiveness acceptability curve.
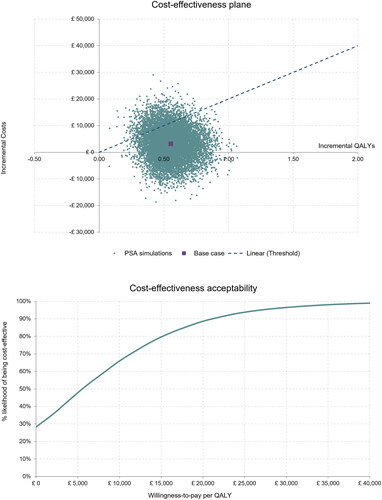
Also presented in is the number of CAUTI events predicted over a patient’s catheter-using lifetime for each treatment arm in the model, and the differences between them. The number needed to treat (NNT) is also presented for each event type; this indicates the number of people who would need to use HCICs rather than UICs (for their remaining lifetime) in order to avoid one such event. In total, seven CAUTIs are avoided; of these, 1.8 would require secondary care (either day-case or inpatient). Moreover, just one patient would need to be prescribed HCICs rather than UICs in order to prevent a hospitalization.
The results of the deterministic sensitivity analyses are presented in . Key findings were that even using the most conservative estimate of treatment effect (informed by the 95% confidence interval reported by Rognoni et al.Citation16), HCICs are still estimated to be cost-effective. Similarly, when the HRQoL benefit for patient preference is removed entirely (set to zero), HCICs are still estimated to be cost-effective. The cost of catheterization was the most influential parameter and the only one with potential to change the conclusion of the evaluation; if the cost of hydrophilic-coated catheters were to increase by 25% then these costs would no longer be offset and deemed good value for money, under the aforementioned cost-effectiveness threshold.
Discussion
The evaluation found HCICs to be cost-effective when compared to UICs in the modelled population. HCICs are not a cost-saving intervention, despite the events avoided, but the additional costs associated with their use are countered by additional QALYs and would be considered good value for money by NICECitation17. Whilst there is uncertainty around model parameters, the above conclusion holds in 89% of simulated cases.
The positive cost-effectiveness result of our evaluation was driven by two key benefits of HCICs. Firstly, there is an established reduction in risk of CAUTIs for people who use HCICs rather than UICs. The magnitude of this reduction was sourced from a network meta-analysis, the gold standard of evidenceCitation27. This translates to a HRQoL benefit for patients as well as cost savings for the healthcare system. Another consequence of the reduction in CAUTIs is that patients are expected to live longer, as their risk of infection-related mortality is reduced.
The other key benefit captured in this evaluation was the relative improvement in HRQoL directly associated with use of HCICs, compared with UICs. This benefit is not attributable to a reduction in infections; it instead reflects the perceived value of fewer steps, convenience, and improved comfort of the catheterizing procedure with an HCIC. The inclusion of this patient satisfaction benefit is aligned with recommendations from the International Consultation on Incontinence as well as existing evaluations in this areaCitation14,Citation38,Citation39.
In spite of the evidence supporting the use of these parameters, a conservative standpoint was taken in the evaluation and all reasonable efforts were taken to avoid overstating their impact. The conclusions of the analysis, however, were robust to the most conservative values assessed for these parameters, as can be seen from the results of the DSA.
In fact, the most uncertain parameters in the model – those informally elicited from clinical opinion – were not considered key drivers of the incremental results and thus this parameter uncertainty was relatively unproblematic for determining cost-effectiveness. Conversely, the cost parameters identified as being highly influential (demonstrated by their position at the top of the Tornado diagram, ) are known with a reasonable amount of certainty, as the base case unit costs were sourced from national cost databases. Micro-costing was used to compute catheterization costs, and given the resultant importance to the model results, these costs were varied by 25%, as opposed to 20%, to incorporate potential overhead costs for HCICs and UICs. It is unlikely that one type of catheterization would be subject to a 25% cost increase without a corresponding cost increase in the other type of catheter. As a result, the cost-effectiveness results can be considered robust to individual cases of parameter uncertainty.
Notably, the conclusion of our evaluation differs to that of an existing evaluation by Bermingham et al.Citation14, who found that UICs were the most cost-effective of the options they assessed. However, in their evaluation UICs were reused, which is off-label use. The NICE guideline development group, who were basing their recommendations upon Bermingham et al.’s research, determined that there was insufficient evidence to inform a recommendation for UICs as a reusable product. In scenario analysis, Bermingham et al. found that UICs ceased to be cost-effective when more than two catheters per day are used. Their evaluation also included gel reservoir catheters as an option, which were found to be slightly more effective than HCICs with a small additional cost and an ICER of approximately £3,000 per QALY gained. The guideline group ultimately determined that patients should be given a choice between hydrophilic and gel-reservoir cathetersCitation40. Our analysis did not include a comparison against gel-reservoir catheters for this reason; it is accepted that both options should be available for patients.
Another more recent evaluation by Clark et al. produced results very similar to ours, despite differences in model structure and parameterizationCitation15. Whilst their evaluation did not factor in patient satisfaction, it included a larger reduction in CAUTIs and so the overall QALY benefit was close to the one predicted by our evaluation. A further evaluation by Rognoni and TarriconeCitation16 also estimated HCICs to be cost-effective, despite, again, excluding the patient preference benefit.
Strengths and limitations of the analysis
Informal expert elicitation was utilized at several key points during model development. Firstly, the proposed model structure was presented to the clinical co-authors to ascertain how well it reflected the clinical pathway. All economic models are simplifications of reality, but it was deemed that this de novo model was appropriate in its assumptions and that its structure allowed the main differences in cost and benefits to be captured.
In addition to validation of the model structure, the clinical co-authors also participated in validating the key model inputs. As with structural limitations, some parameter uncertainty is inevitable in economic models. Where evidence was scarce and modeler assumptions were required, these assumptions were, wherever possible, confirmed to be clinically plausible.
The main limitations of this evaluation relate to the simplification of the treatment pathway that was necessary in developing a useful tool for decision-making. For instance, the issue of microbial resistance has been well-documented in the literature, and the costs associated with an antibiotic-resistant infection have not been captured explicitly in this evaluationCitation41. The results are, therefore, possibly understating the true benefit of the reduction in frequency of CAUTIs attributable to hydrophilic-coated catheters over uncoated catheters.
Moreover, long-term effects of UTIs, such as urinary incontinence, bladder damage, kidney damage, and kidney failure were not included within the analysis, which is a source of conservative bias.
Further, many CAUTIs may be considered recurrent, and it is likely that recurrent infections are associated with a higher cost (for example due to referrals to specialists, additional monitoring) as well as a greater impact on HRQoL. This, too, was not captured in the evaluation, and is another potential source of conservative bias in the results.
The progression of the underlying condition of the cohort was not modelled; it was assumed that the effect on HRQoL and mortality associated with MS, or an SCI was constant over time. This was a necessary simplifying assumption and should not substantially bias the incremental results.
In terms of the generalizability of the results of this evaluation, we acknowledge that the modelled cohort may not entirely reflect the population of interest. For instance, an assumption was made that the cohort should be constructed of an equal split of individuals with SCI and with MS. However, the cost-effectiveness results are robust to changes in this population breakdown; for instance, a cohort of 100% SCI individuals produces very similar results.
Further, the starting age of the MS portion of the cohort reflects the mean age of MS catheter users, and not the mean age of catheter initiation in these patients. This potential overestimation in the starting age effectively reduces the period over which hydrophilic-coated catheter users can accrue incremental benefits compared with uncoated catheter users and is therefore another source of conservative bias.
Overall, the limitations are likely to have led to an underestimation of the cost-effectiveness of hydrophilic-coated catheters, which strengthens the overall conclusion of the analysis. Nonetheless, these potential sources of bias should be considered when interpreting the results of this evaluation.
Conclusions
In summary, HCICs are highly likely to be a cost-effective alternative to UICs, a result that is driven by substantial reductions in the number of CAUTIs. Their adoption across clinical practice could avoid a substantial number of infections, thereby freeing up healthcare resource in the NHS and reducing antibiotic use in users of urinary catheters.
Transparency
Declaration of funding
Coloplast sponsored this economic analysis and contributed to the development and review of this article.
Declaration of financial/other relationships
Hannah Baker, Brooke Avey and Stuart Mealing are employees of York Health Economics Consortium, who were commissioned by Coloplast to provide consultancy, develop the economic model, and draft the manuscript. Line Overbeck Rethmeier and Marie Lynge Buchter are employees of Coloplast. Marcio Averbeck is a paid advisor to Coloplast, Medtronic, Boston Scientific, and GSK. Nikesh Thiruchelvam is a paid advisor to Coloplast. He also receives teaching fees from Boston Scientific. Peer reviewers on this manuscript have no relevant financial or other relationships to disclose.
Author contributions
All authors contributed to the model design, parameter elicitation and validation of results. Hannah Baker, Brooke Avey and Stuart Mealing were responsible for model construction. All authors contributed to the manuscript draft.
Table_S1_-_Micro-costing_UTI_treatment.docx
Download MS Word (16.7 KB)Acknowledgements
None.
References
- Spinal Injuries Association. Spinal cord injury paralyses someone every four hours, new estimates reveal. 2019. Available from: https://www.spinal.co.uk/news/spinal-cord-injury-paralyses-someone-every-four-hours-new-estimates-reveal/
- MS Society. MS in the UK. 2020.
- National Institute of Health (NIH). Definition & Facts of Urinary Retention 2019. Available from: https://www.niddk.nih.gov/health-information/urologic-diseases/urinary-retention/definition-facts
- Vahr S, Cobussen-Boekhorst H, Eikenboom J, et al. Evidence-based guidelines for best practice in urological health care: catheterisation (urethral intermittent in adults). Arnhem (The Netherlands): European Association of Urology Nurses. 2013. 35 p.
- Lapides J, Diokno AC, Silber SJ, et al. Clean, intermittent self-catheterization in the treatment of urinary tract disease. J Urol. 1972;107(3):458–461.
- Weld KJ, Dmochowski RR. Effect of bladder management on urological complications in spinal cord injured patients. J Urol. 2000;163(3):768–772.
- Wyndaele J-J, Maes D. Clean intermittent self-catheterization: a 12-year followup. J Urol. 1990;143(5):906–908.
- Newman DK, New PW, Heriseanu R, et al. Intermittent catheterization with single-or multiple-reuse catheters: clinical study on safety and impact on quality of life. Int Urol Nephrol. 2020;52(8):1443–1451.
- Vallejo-Torres L, Pujol M, Shaw E, et al. Cost of hospitalised patients due to complicated urinary tract infections: a retrospective observational study in countries with high prevalence of multidrug-resistant gram-negative bacteria: the COMBACTE-MAGNET, RESCUING study. BMJ Open. 2018;8(4):e020251.
- All Party Parliamentary Group for Continence Care. Continence Care Services England 2013: survey Report. APPG; 2013. Available from: http://www.appgcontinence.org.uk/wp-content/uploads/2020/02/Continence-Care-Services-England-Report-2013.pdf
- Kennelly M, Thiruchelvam N, Averbeck MA, et al. Adult neurogenic lower urinary tract dysfunction and intermittent catheterisation in a community setting: risk factors model for urinary tract infections. Adv Urol. 2019;2019:1–13.
- Stensballe J, Looms D, Nielsen P, et al. Hydrophilic-coated catheters for intermittent catheterisation reduce urethral micro trauma: a prospective, randomised, participant-blinded, crossover study of three different types of catheters. Eur Urol. 2005;48(6):978–983.
- National Institute for Health and Care Excellence (NICE). Healthcare-associated infections: prevention and control in primary and community care: clinical guideline. London: NICE; 2012.
- Bermingham SL, Hodgkinson S, Wright S, et al. Intermittent self catheterisation with hydrophilic, gel reservoir, and non-coated catheters: a systematic review and cost effectiveness analysis. BMJ. 2013;346:e8639.
- Clark J, Mealing S, Scott D, et al. A cost-effectiveness analysis of long-term intermittent catheterisation with hydrophilic and uncoated catheters. Spinal Cord. 2016;54(1):73–77.
- Rognoni C, Tarricone R. Healthcare resource consumption for intermittent urinary catheterisation: cost-effectiveness of hydrophilic catheters and budget impact analyses. BMJ Open. 2017;7(1):e012360.
- National Institute for Health and Care Excellence (NICE). Health technology evaluations: the manual (process and methods). London: NICE; 2022.
- National Spinal Cord Injury Statistical Centre. Facts and figures at a glance 2011. Available from: https://www.nscisc.uab.edu/
- Mahajan ST, Frasure HE, Marrie RA. The prevalence of urinary catheterization in women and men with multiple sclerosis. J Spinal Cord Med. 2013;36(6):632–637.
- Office for National Statistics. England and Wales National Life Tables 2018–2020. Available from: https://www.ons.gov.uk/peoplepopulationandcommunity/birthsdeathsandmarriages/lifeexpectancies/datasets/mortalityratesqxbysingleyearofage.
- Chamberlain JD, Meier S, Mader L, et al. Mortality and longevity after a spinal cord injury: systematic review and meta-analysis. Neuroepidemiology. 2015;44(3):182–198.
- Smyrke N, Dunn N, Murley C, et al. Standardized mortality ratios in multiple sclerosis: systematic review with meta‐analysis. Acta Neuro Scandinavica. 2022;145(3):360–370.
- Cardenas DD, Moore KN, Dannels-McClure A, et al. Intermittent catheterization with a hydrophilic-coated catheter delays urinary tract infections in acute spinal cord injury: a prospective, randomized, multicenter trial. PM R. 2011;3(5):408–417.
- Cardenas DD, Hoffman JM. Hydrophilic catheters versus noncoated catheters for reducing the incidence of urinary tract infections: a randomized controlled trial. Arch Phys Med Rehabil. 2009;90(10):1668–1671.
- De Ridder D, Everaert K, Fernández LG, et al. Intermittent catheterisation with hydrophilic-coated catheters (SpeediCath) reduces the risk of clinical urinary tract infection in spinal cord injured patients: a prospective randomised parallel comparative trial. Eur Urol. 2005;48(6):991–995.
- Woodbury MG, Hayes KC, Askes HK. Intermittent catheterization practices following spinal cord injury: a national survey. Can J Urol. 2008;15(3):4065–4071.
- Rognoni C, Tarricone R. Intermittent catheterisation with hydrophilic and non-hydrophilic urinary catheters: systematic literature review and meta-analyses. BMC Urol. 2017;17(1):4–11.
- Drug Tariff, PartIXA (Appliances) [Internet]. November 2021. Available from: https://www.nhsbsa.nhs.uk/pharmacies-gp-practices-and-appliance-contractors/drug-tariff.
- Orme M, Kerrigan J, Tyas D, et al. The effect of disease, functional status, and relapses on the utility of people with multiple sclerosis in the UK. Value Health. 2007;10(1):54–60.
- Averbeck MA, Krassioukov A, Thiruchelvam N, et al. The impact of different scenarios for intermittent bladder catheterization on health state utilities: results from an internet-based time trade-off survey. J Med Econ. 2018;21(10):945–952.
- NHS reference costs 2019-20 v2 [Internet]. 2021.
- Smith DR, Pouwels KB, Hopkins S, et al. Epidemiology and health-economic burden of urinary-catheter-associated infection in English NHS hospitals: a probabilistic modelling study. J Hosp Infect. 2019;103(1):44–54.
- National Institute for Health and Care Excellence (NICE). Bortezomib for previously untreated mantle cell lymphoma [TA370]. London: NICE; 2015.
- Kind PH. Geoffrey; Macran, Susan. UK population norms for EQ-5D. York: University of York; 1999.
- National Institute for Health and Care Excellence (NICE). Urinary tract infection (catheter-associated): antimicrobial prescribing. Recommendations. London: NICE; 2018.
- Drugs and pharmaceutical electronic market information tool (eMIT) [Internet]. 2021. Available from: https://www.gov.uk/government/publications/drugs-and-pharmaceutical-electronic-market-information-emit.
- National Institute for Health and Care Excellence (NICE). British National Formulary. London: NICE; 2021. Available from: https://bnf.nice.org.uk/drug/.
- Cottenden A, Fader M, Beeckman D, et al. Management of incontinence using continence products. 6th International Consultation on Incontinence. Tokyo, Japan: International Continence Society; International Consultation on Urological Diseases; 2017.
- Welk B, Isaranuwatchai W, Krassioukov A, et al. Cost-effectiveness of hydrophilic-coated intermittent catheters compared with uncoated catheters in Canada: a public payer perspective. J Med Econ. 2018;21(7):639–648.
- National Institute for Health and Care Excellence (NICE). Clinical guideline CG139: healthcare-associated infections: prevention and control in primary and community care. London: NICE; 2017.
- Murray CJ, Ikuta K, Sharara F, et al. Global burden of bacterial antimicrobial resistance in 2019: a systematic analysis. Lancet. 2022;399:629–655.