ABSTRACT
The purpose of this study was to observe the therapeutic effect of extracorporeal shock wave (ESW) on extensional joint contracture of knee joint in rats and its mechanism on articular capsule fibrosis. Thirty-two SD rats were randomly divided into blank control, immobilization, natural recovery, and ESW intervention groups. Except for the control group, the left knee joints of other rats were fixed with external fixation brace for 4 weeks when they were fully extended to form joint contracture. The effect of intervention was assessed by evaluating joint contracture, total cell count and collagen deposition in joint capsule, and protein expression levels of TGF-β1, p-Smad2/3, Smad2/3, p-JNK, JNK, I and III collagen in joint capsule. ESW can effectively reduce arthrogenic contracture, improve the histopathological changes of anterior joint capsule, inhibit the high expression of target protein and the excessive activation of TGF-β1/Smad2/3/JNK signal pathway. Inhibition of excessive activation of TGF-β1/Smad2/3/JNK pathway may be one of the potential molecular mechanisms by which extracorporeal shock wave can play a role.
1. Introduction
Joint contracture, which is a central complication after joint surgery or longtime immobilization, is a common clinical problem affecting joint functionCitation1,Citation2. As the largest synovial joint and the main load-bearing joint of the body, the knee joint is easy to be injured in sports and accidents, resulting in knee joint contracture. After the occurrence of knee joint contracture, the range of motion (ROM) of the joint is limited, accompanied by varying degrees of functional declineCitation3, resulting in irreversible joint disability. Previous literatures have shown that joint capsule is the key structure that can limit the ROM of contracture knee jointCitation4,Citation5. Fibrotic changes of joint capsule tissue are the most important pathological change of joint contractureCitation3,Citation6. The pathological changes of joint capsule fibrosis included inflammation, proliferation of collagen fibers, proliferation of fibroblasts, production of matrix metalloproteinases and abnormal expression of growth factorsCitation7,Citation8.
The fibrotic process of joint capsule is regulated by many factors. Previous animal experimental studies have shown that overexpression of transforming growth factor-β (TGF-β) 1, a fibrogenic factor during joint fixation, is one of the important mechanisms leading to joint capsule fibrosisCitation9. Smad protein is one of the important transcription factors downstream of TGF-β signaling pathway. Hao J et al.Citation10 found that the expression of TGF-β, Smad2/3, and Smad4 increased in myocardial scar tissue of rats with myocardial infarction. Wang et al.Citation11 found that naringin significantly reduced the deposition of α-smooth muscle actin, fibrosis-related cytokines, and collagen in fibrotic NRK-52E cells through the TGF-β/Smad signal pathway, which could effectively antagonize the progression of renal interstitial fibrosis. In addition, some studies have shown that c-Jun N-terminal kinase (JNK) under the action of TGF-β can directly control the phosphorylation of the Smad3 protein, promote the interaction between Smad3 and Smad4 and the activation of JNK, or indirectly affect the transcriptional activity of Smad, thus slowly and continuously promoting the activation of JNKCitation12,Citation13. The biological activity of TGF-β1 can be regulated by the abnormal expression of JNK protein, which can affect the development of fibrotic diseases to some extentCitation14,Citation15. JNK signaling pathway is involved in the development of liver, kidney, heart, lung, and other tissue fibrosis, and its regulatory mechanism is very complexCitation16. At present, there are few reports on the role of TGF-β/Smad and JNK pathways in joint capsule fibrosis. In view of the expression of JNK in tissue fibrosis, we hypothesized that there was a similar expression of JNK in joint capsule fibrosis.
The biotherapeutic effect of extracorporeal shock wave (ESW) is mainly achieved by mechanical transmission and cavitation effect, which can promote tissue healing and angiogenesis, reduce inflammation and pain, release adhesion, and improve local blood circulationCitation17,Citation18. It has been reported that ESW can accelerate regeneration after acute skeletal muscle injuryCitation19. Cui HS et al.Citation20 discussed the cellular and molecular biological mechanism of ESW treatment of scar tissue. After ESW treatment, the expression of TGF-β1 decreased, α-smooth muscle actin, type I collagen, and fibronectin decreased significantly. Our team’s previous animal experimentsCitation21,Citation22 confirmed the biological effects of divergent ESW in improving muscle fibrosis and muscle contracture. ESW is widely used in musculoskeletal diseases, but the treatment of arthrogenic contracture by ESW is rarely reported. Based on this, this study mainly focused on the therapeutic effect of ESW on arthrogenic contracture and explored its anti-fibrosis mechanism.
The main purpose of this study was to determine whether ESW can effectively improve joint capsule fibrosis and arthrogenic contracture. On the basis of the established model of extension joint contracture in rats, this study was discussed from the following aspects: (1) improvement of joint contracture; (2) improvement of histopathological characteristics of the joint capsule; and (3) changes in the protein expression of TGF-β1, p-Smad2/3/Samd2/3, and p-JNK/JNK in the joint capsule.
2. Methods
Animals
A total of 32 skeletally mature male Sprague Dawley rats (approximately 3 months old, 200–250 g) obtained from the Experimental Animal Center of Anhui Medical University, Hefei, China, were used in this experiment. The rats were randomly divided into the following four groups: control group (C), immobilization for 4 weeks group (I), natural recovery group (R), and ESW treatment group (S). There were 8 rats in each group, where they were housed two per cage (370 × 270 × 160 mm) at 22–27°C with a 12-hours light–dark cycle and allowed free access to food and water. In group C, the left knee joint was not fixed to serve as a control. In group I, the left knee joint was only fixed for 4 weeks at full extension using the fixation brace (Patent No.202120470158.0) and fixation method was noted in the previous studiesCitation23 (). In group R, the left knee joint was fixed for 4 weeks before the rats underwent 4 weeks of self-recovery, without any special rehabilitation protocol. In group S, the left knee joint was fixed for 4 weeks before the rats underwent 4 weeks of rESW. In order to observe whether the brace fixed the knee joint in the fully extended position, the rats were anesthetized with 2% pentobarbital sodium and placed flat under the X-ray (Siemens; Germany) camera to take the X-ray film of the left hindlimb after the knee brace was fixed. The health status of the rats and the immobilization of the brace were observed daily, and the immobilization strength was adjusted in time to ensure effective immobilization. The rats were in good condition, and only one died due to mites.
Figure 1. Schematic diagram of knee joint contracture model, interventions, measurement of joint contracture and joint capsule sampling.
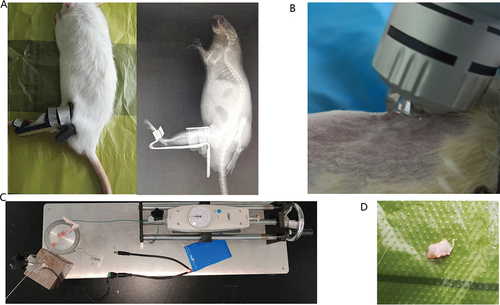
All procedures performed in this animal experiment were approved by the Institutional Animal Care and Use Committee of Anhui Medical University (LLSC20221126).
Intervention methods
A total of 8 rats in the S group were treated with ESW twice a week for 4 weeks. A radial shock wave device (DolorClast, Switzerland) and its “Radial” handpiece was used in the experiment twice in a week. The 6-mm applicator was used, and the left hindlimb was cleaned at the beginning of the experiment. To avoid experimental errors due to anesthesia, the rats were artificially fixed with suitable strength to avoid injury due to excessive struggle. The head of the rat was put into the opaque glove, with the knee joint outside the glove, and the limbs of the rat were properly fixed so as to reduce the damage caused by excessive struggle of the rat. Then, the ultrasound gel was spread evenly over the skin surface of the suprapatellar pouch of the left hindlimb, avoiding air between the treatment head of the radial shock and skin, which could minimize the loss of shock wave energy and maintain stable transmission of the wave. Each rat received less than 500 times impulses of shock waves at a small dose of 1.5 bar, 4 Hz to the left suprapatellar bursa area, in order to adapt the rats to the stimulation. According to the instruction manual of the manufacturer and our previous experimental experiences, the rat received 2000 times impulses at an experimental dose of 2.5 bar, 6 Hz to the same area (). Immediately after the application of the shockwave, the left knee of the rats was examined for swelling and hematoma. Meanwhile, cold using ice packs for 10 min.
Measurement of arthrogenic contracture
At the end point, the left hind limb of the rat was disarticulated at the hip and the knee joint after the rats were euthanized with an overdose of sodium pentobarbital. A mechanical measuring device (Patent No.ZL202120996643.1) and measuring method for joint ROM, designed in the previous studies, were used in this studyCitation23 (). The Kirschner wire was inserted parallel to the femur from the rear of the tip of the greater trochanter of the femur. The Kirschner wire was linked to the femur and fixed. The distal end of the tibia was fixed on the rotary table with a disposable plastic bandage. The digital dynamometer was fixed above the slide rail. A thin rope was fixed between the groove of the rotary table and the digital dynamometer. When the drive wheel is turned, the rotary table rotates and synchronously drives the tibia to rotate. The angle change between the femur and tibia can be calculated according to the scale of the rotary table. The radius of the disk (namely the arm of force) was constant, so the torque and the force are linear. The torque applied was calculated by multiplying the force by the constant radius of the disc. The standard torque for measuring ROM of the knee joint is 5.3N-cm. This moment can pull the knee joint to its maximum flexion angle, but it will not damage the soft tissue. The previous picture is blurred and replaced. The ROM of the left knee joint in each group was measured after myotomy with a standard torque of 0.053 N.m. The following formula was used for calculating the degree of arthrogenic contracture:
Arthrogenic contracture = ROM after myotomy (of the control knee)-ROM after myotomy (of the contracted knee)
Tissue preparation and pathological staining
After measuring ROM, the anterior joint capsule of the left knee joint was taken and divided into equal-sized samples (). Some tissue samples were fixed with 4% of paraformaldehyde at 4°C for 24 hours. Each specimen was cut into a series of 6 µm sections with a microtome, deparaffinized, and then stained with H&E and Masson to detect pathological changes in the total number of cells and collagen deposition of the joint capsule. Sections of each sample were stained following the steps in the instructions for use of the H&E and Masson staining kits, and the HE stained sections were observed under a microscope with a magnification of 400×(Pannoramic MIDI; 3DHISTECH, Europe), and the Masson stained sections were observed under a microscope with a magnification of 200×, with 6 fields of view randomly selected and photographed, and analyzed by Image Pro Plus 6.0 software. The number of cells and the deposition of collagen fibers in the joint capsule were observed by HE and Masson staining. The cells in the joint capsule mainly include fibrotic cells and inflammatory cells, which are mainly distinguished by morphological identification. Fibrotic cells are fusiform, with less cytoplasm, weak eosinophilia, small nucleus, and deep staining. Under the electron microscope, the rough endoplasmic reticulum and Golgi apparatus in the cells were not developed. We counted these different types of cells together to simply show inflammation and fibrosis in the joint capsule.
It is known that there are four types of collagen. Under the polarized light microscope, four types of collagen fibers can be displayed according to the difference in birefringence and coloration using the Sirius red staining method. Collagen 1 shows a strong yellow, is closely arranged, and highly refractive. Collagen 3 shows green, fine fiber, with weak birefringence. Dye the sections of each sample according to the steps in the instructions for the use of the Sirius red staining kit, observe the sections of Sirius red staining under the polarized light microscope (nikon C-SP 770,788) with a magnification of 200 times, randomly select and take 3 fields of view, and analyze them through the Image Pro Plus 6.0 software.
Three rat tissue samples were frozen in liquid nitrogen and then stored at −80°C for subsequent protein experiments. Due to budget and time constraints, we had to consider the number of samples that could be processed for western blotting. We aimed to strike a balance between resource allocation and obtaining meaningful data. Prior to conducting the main experiment, we performed a pilot study that indicated a strong and consistent effect size in the parameters being measured. This gave us confidence that using three samples per group would be sufficient to observe the intended effect. In addition, as part of our commitment to minimizing animal use in research, we followed the principles of the 3Rs (Replacement, Reduction, and Refinement). By selecting only three rats per group for western blot analysis, we were able to minimize the number of animals subjected to more invasive procedures. We ensured that the selected rats were representative of their respective groups by randomizing the selection process and confirming that their baseline characteristics were comparable.
Protein extraction and Western blot detection
The joint capsule samples were ground into powder with liquid nitrogen using a grinder and homogenized in RIPA buffer (Beyotime, China) containing protease inhibitors at 4°C. Homogenates were centrifuged at 12,000 g for 30 min, three times at 4°C, and the resulting supernatants were collected. The protein concentrations were determined using the bicinchoninic acid method. Protein lysates were separated on a 10% sodium dodecyl sulfate-polyacrylamide electrophoresis gel and transferred onto polyvinylidene fluoride membranes. After being blocked with 5% nonfat dry milk in Tris-buffered saline Tween-20 (TBST) at room temperature for 2 h 30 min, the membranes were incubated with rabbit anti-TGF-β1 antibody (1:1500; Affinity), rabbit anti-Smad2/3 antibody (1:1500; Affinity), rabbit anti-phospho-Smad2/3 antibody (1:1500; Affinity), rabbit anti-JNK antibody (1:1500; Affinity), rabbit anti-phospho-JNK (1:1500; Affinity), rabbit anti-Collagen I antibody (1:2500; Affinity), and rabbit anti-Collagen III antibody (1:1000; Affinity) at 4°C overnight. On the second day, after being washed in TBST solution three times for 10 min per wash, the membranes were incubated with peroxidase-conjugated affinipure goat anti-rabbit IgG-HRP (1:7000; Affinity) as the secondary antibody for 1 h 30 min at room temperature. After being washed three times with TBST or 10 min per wash, the membranes were evaluated with the enhanced chemiluminescence system in accordance with the manufacturer’s instructions. The band densities were quantified using Image J software (National Institutes of Health, USA). TGF-β1, collagen I, and collagen III protein levels were calculated by comparison with the amount of GAPDH (1:15000; Affinity) as a loading control. JNK and Smad2/3 were used to normalize p-JNK and p-Smad2/3, respectively.
Statistical Analyses
All experimental data were expressed as mean ± standard deviation, and the data were analyzed and processed with the SPSS 21.0 system. All data were in line with normal distribution and homogeneity of variance, which were tested by the Shapiro-Wilk method and the Levene method. Differences between groups were analyzed by a post-hoc SNK test and a one-way ANOVA. The arthrogenic contracture, the degree of collagen deposition, the number of total cells, and the expression levels of TGF-β1, Smad2/3, p-Smad2/3, JNK, p-JNK, collagen I and collagen III were analyzed by ANOVA across the groups. The difference was statistically significant with a P value of less than 0.05 (P < 0.05).
3. Results
Arthrogenic contracture
The arthrogenic contracture in the four groups is shown in (group C, 0°±0°; group I, 41.20°±1.32°; group R, 39.71°±0.96°; group S, 30.51°±1.30°). The arthrogenic contracture in group I and R was significantly higher than that in group C (C vs I, P = 0.0000; C vs R, P = 0.0000). Compared with group I, the arthrogenic contracture in group R was alleviated, and the difference was not statistically significant (P = 0.0576). Compared with group I and group R, the arthrogenic contracture of group S was significantly improved (I vs S, P = 0.0000; R vs S, P = 0.0000).
Figure 2. Arthrogenic contracture, H&E staining, and Masson staining.
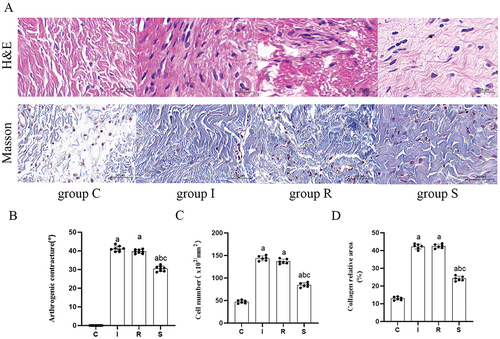
Cell count
The cell counts of the four groups are shown in (group C, 47.33 ± 3.25, group I, 143.83 ± 4.84, group R, 136.83 ± 4.67, group S, 84.50 ± 4.35). HE staining showed that, compared with group C, the number of cells in the anterior joint capsule of the knee joint was significantly increased in group I and group R (C vs I, P = 0.0000; C vs R, P = 0.0000). Compared with group I, the cell count in group R was slightly lower (P = 0.0805). Compared with group I and group R, the number of cells in group S decreased significantly (I vs S, P = 0.0001; R vs S, P = 0.0000).
Collagen deposition
The collagen deposition area of the four groups is shown in (group C, 12.95 ± 0.84, group I, 42.27 ± 1.31, group R, 42.46 ± 1.06, group S, 24.18 ± 1.21). The collagen deposition in groups I and R was significantly higher than that in group C (C vs I, P = 0.0000; C vs R, P = 0.0000). Compared with group I, the collagen deposition in group R had no significant change (P = 0.9931). Compared with group I and group R, the collagen deposition in group S decreased significantly (I vs S, P = 0.0000; R vs S, P = 0.0000).
Under the polarized light microscope, the deposition of type I and III collagen is shown in . Compared with group C, the deposition of type I and III collagen in groups I and R increased significantly (collagen I: C vs I, P = 0.0000; C vs R, P = 0.0000; collagen III: C vs I, P = 0.0000; C vs R, P = 0.0000). Compared with group I, there was no significant change in type I and type III collagen in group R (collagen I: P = 0.4283; collagen III: P = 0.2083). Compared with group I and group R, the deposition of type I and III collagen in group S decreased significantly (collagen I: I vs S, P = 0.0001; R vs S, P = 0.0000; collagen III: I vs S, P = 0.0001; R vs S, P = 0.0000).
Figure 3. Sirius red staining.
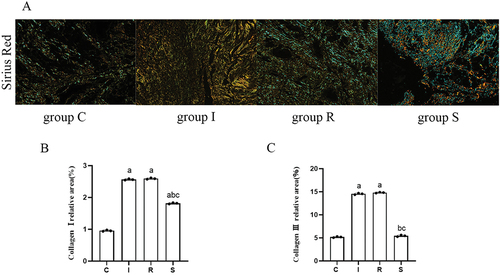
Protein expression levels
The protein expression levels of TGF-β1, p-Smad2/3/Smad2/3, p-JNK/JNK, collagen I and III in the anterior joint capsule of the four groups of knees are shown in . Compared with group C, the expression levels of TGF-β1 (C vs I, P = 0.0000; C vs R, P = 0.0000), p-Smad2/3/Smad2/3 (C vs I, P = 0.0001; C vs R, P = 0.0000), p-JNK/JNK (C vs I, P = 0.0000; C vs R, P = 0.0000), collagen I (C vs I, P = 0.0028; C vs R, P = 0.0051) and III (C vs I, P = 0.0000; C vs R, P = 0.0000) in groups I and R were all increased, the expression level of TGF-β1 (P = 0.0000) and collagen III (P = 0.0000) in group S was increased, and the expression levels of p-Smad2/3/Smad2/3 (P = 0.9864), p-JNK/JNK (P = 0.3621) and collagen I (P = 0.8398) in group S were not significantly changed. Compared with group I, the expression levels of TGF-β1 (P = 0.9999), p-Smad2/3/Smad2/3 (P = 0.3721), and p-JNK/JNK (P = 0.6459) in group R did not obviously change. Compared with groups I and R, the expression levels of TGF-β1 (I vs S, P = 0.0000; R vs S, P = 0.0000), p-Smad2/3/Smad2/3 (I vs S, P = 0.0001; R vs S, P = 0.0000), p-JNK/JNK (I vs S, P = 0.0000; R vs S, P = 0.0000), collagen I (I vs S, P = 0.0075; R vs S, P = 0.0146) and III (I vs S, P = 0.0279; R vs S, P = 0.0033) in group S were significantly decreased.
Figure 4. Protein expression levels.
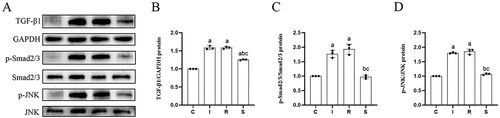
Figure 5. Protein expression levels.
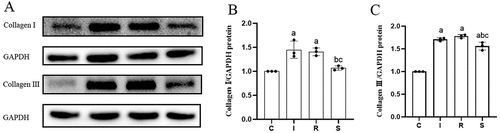
4. Discussion
ESW can effectively improve the severity of knee joint contracture after immobilization for 4 weeks by inhibiting joint capsule fibrosis and reducing collagen deposition. The results suggest that ESW may be an effective intervention method for joint contractures caused by immobilization.
In this study, an aluminum splint fixation brace for the rat knee joint prepared by our group was used to fix the straightened position of the left knee joint for 4 weeks. The rats were killed at each time point, and the contracture angle of the left knee joint after myotomy was measured. The results showed that after 4 weeks of fixation, the contracture angle of the left knee joint was significantly higher than that of the C group. However, after 4 weeks of natural recovery, the angle of knee contracture did not change significantly compared with the I group, suggesting that free movement can not improve the severity of arthrogenic contracture, which is consistent with the results of previous studiesCitation6,Citation23. The degree of knee joint contracture was significantly improved after ESW treatment. ESW is a kind of pressure wave produced by aerodynamics that has a mechanical effect and a cavitation effect. When the shock wave enters the human body, it will produce different mechanical stress effects at the interface of different tissues because of the different media it touches. Produce different tensile and compressive stresses, so as to release joint and soft tissue adhesion, improve fibrosis, and reduce the severity of joint contractureCitation21,Citation22,Citation24. Our team’s previous studies have shownCitation23 that ESW may improve joint fibrosis and reduce joint contracture by inhibiting the high expression of TGF-β1, fibroblast growth factor 2, extracellularly regulated protein kinase 2, and the overactivation of MAPK/ERK. ESW can improve the metabolism of the treated area, reduce inflammatory reactions, promote tissue regeneration, unblock occluded microvessels and epithelial cell regeneration, and play a role in tissue wound repairCitation24, Citation25. This may be the reason why ESW inhibits joint capsule fibrosis and improves knee joint contracture. On this basis, this experiment continued to investigate the effect of ESW on the progression of knee contracture joint capsule fibrosis and the expression of TGF-β1, p-JNK/JNK, and p-Smad2/3/Smad2/3 in the fibrotic joint capsule.
Excessive proliferation of fibroblasts and inflammatory cells is one of the important features of fibrotic diseases. In other diseases, fibroblasts can transform acute inflammation into chronic, persistent inflammationCitation26,Citation27. It also plays an important role in the process of joint capsule fibrosis. It can migrate and accumulate around the joint capsule during the formation of joint contractureCitation28,Citation29. Abdel MP et al.Citation29 have also shown that fibroblasts can be activated by fibrotic factors, such as TGF-β, and promote the proliferation of type I and III collagen. A study using a rat model of joint fibrosis showed that the expression of type I collagen mRNA and the number of myofibroblasts producing extracellular matrix increased in the fixation groupCitation5. The results of the pathological staining of the anterior joint capsule in this study showed that the number of cells and the level of collagen deposition in the I group were higher than those in the C group. After 4 weeks of natural recovery, the number of cells and the level of collagen deposition did not change significantly, suggesting that natural recovery could not effectively reduce the collagen deposition and cell number in the contractured knee joint capsule. The collagen deposition and the number of cells decreased significantly in the S group, suggesting that the ESW may improve the severity of joint capsule fibrosis by reducing the number of inflammatory and fibrotic cells and collagen I, III deposition, which is similar to previous studiesCitation30. Simplicio CL et al.Citation31 have also shown that low-energy ESW can stimulate the polarity shift of macrophages from M1 to M2, resulting in good anti-inflammatory effects. Low energy ESW can also effectively inhibit the formation of hypertrophic scars by inhibiting the density of fibroblastsCitation32. Kondo Y et al.Citation33 studied the effects of stretch and hyperthermia on soleus muscle contracture in rats. The results showed that collagen deposition was improved in the stretch group, and stretching effectively promoted the recovery of muscle contracture. In connective tissue, fibroblasts are sensitive to mechanical stimulation, and ESW may regulate tissue physical properties and cell morphology through mechanical stimulation, which may be an important reason for reducing cell migration and collagen deposition.
Previous studies have shown that TGF-β1/Smad signal pathway is one of the main signal pathways in fibrotic diseases, which affects the occurrence and development of fibrosis, which is consistent with the results of this studyCitation34. Zhang QB et al.Citation9 have also shown that proper mechanical stimulation can inhibit the overexpression of TGF-β1 mRNA in fibroblasts and reduce the degree of subsequent fibrosis. In this study, it was found that after joint immobilization, the expression levels of TGF-β1 and p-Smad2/3/Smad2/3 in the anterior joint capsule of the knee increased significantly in the I group, suggesting that there is an association between the overexpression of TGF-β1 and p-Smad2/3/Smad2/3 and joint capsule fibrosis. After 4 weeks of natural recovery, the protein expression levels of TGF-β1 and p-Smad2/3/Smad2/3 had no significant change, but significantly improved after ESW treatment, suggesting that ESW may reduce the severity of joint capsule fibrosis by regulating the expression of TGF-β1 and Smad2/3 proteins. When the ESW passes through the human tissue, the pressure in the tissue changes rapidly and rapidly forms the mechanical stimulation caused by the expansion and collapse of small bubbles, which is an important mechanism for the biotherapeutic effect of the shock wave.
The regulatory mechanism of the JNK signal transduction pathway in fibrotic diseases is complex. Firstly, JNK activation can promote the process of fibrosis along with TGF-β/Smad and other signal pathways. Second, it can also promote fibrosis by accelerating mitochondrial division and endoplasmic reticulum stress. In this study, the expression levels of p-JNK/JNK protein increased significantly in the I group, suggesting that there is a causal relationship between the overexpression of p-JNK/JNK and the formation of joint capsule fibrosis. It increased after natural recovery, but it was not statistically significant. The level of p-JNK/JNK decreased significantly after ESW treatment, suggesting that ESW may reduce joint capsule fibrosis and improve the severity of joint contracture by regulating the expression levels of JNK and p-JNK proteins.
There are some limitations to this study. In this experiment, we only detected the expression levels of TGF-β1, p-JNK/JNK, and p-Smad2/3/Smad2/3, but failed to explain the specific regulation mechanisms of JNK signal pathway and TGF-β1/Smad2/3 signal pathway in joint fibrosis. In the future, combined with in vitro experiments, we will further explore the possible synergistic effect of JNK signal pathway and TGF-β1/Smad2/3 signal pathway in the pathogenesis of joint contracture.
5. Conclusion
During the fixation of the rat knee joint contracture model, joint dysfunction and anterior knee joint capsule fibrosis occurred. As one of the important causes of arthrogenic contracture, anterior joint capsule fibrosis of the knee joint may have a causal relationship with the protein expression levels of TGF-β1, p-JNK/JNK, p-Smad2/3/Smad2/3, collagen I and III.
ESW may reduce the expression levels of TGF-β1, p-Samd2/3/Smad2/3, and p-JNK/JNK in the joint capsule, inhibit anterior joint capsule fibrosis, reduce knee joint contracture, and improve knee joint function.
List of abbreviations
Authors’ contributions
Chao Hu conceived of the study, participated in its design and coordination, and drafted the manuscript. Quan Bing Zhang carried out the molecular studies. Yun Zhou participated in its design and coordination and helped to draft the manuscript. Feng Wang performed the statistical analysis in the revised manuscript. Hua Wang participated in the revision of the manuscript. All authors read and approved the final manuscript.
Ethics approval and consent to participate
Animal care and experimental procedures were performed in accordance with the Guidelines for Animal Experimentation of Anhui Medical University and were approved by the Institutional Animal Care and Use Committee (LLSC20221126).
Availability of data and material
The datasets used and analyzed during the current study are available from the corresponding author on reasonable request. All the data generated or analyzed during this study are included in this published article. The manuscript, including related data, figures, and tables, has not been previously published and is not under consideration elsewhere.
Ethics approval and consent to participate
Animal care and experimental procedures were performed in accordance with the Guidelines for Animal Experimentation of Anhui Medical University and were approved by the Institutional Animal Care and Use Committee (LLSC20221126).
Authors’ contributions
Chao Hu conceived of the study, participated in its design and coordination, and drafted the manuscript. Quan Bing Zhang carried out the molecular studies. Yun Zhou participated in its design and coordination and helped to draft the manuscript. Feng Wang performed the statistical analysis in the revised manuscript. Hua Wang participated in the revision of the manuscript. All authors read and approved the final manuscript.
Disclosure statement
No potential conflict of interest was reported by the authors.
Additional information
Funding
References
- Gaston P, Will EM, Keating JF. Recovery of knee function following fracture of the tibial plateau. J Bone Joint Surg Br. 2005;87(9):1233–1236. doi:10.1302/0301-620X.87B9.16276.
- Anakwe RE, Middleton SD, Jenkins PJ, McQueen MM, Court-Brown CM. Patient-reported outcomes after simple dislocation of the elbow. J Bone Joint Surg Am. 2011;93(13):1220–1226. doi:10.2106/JBJS.J.00860.
- Zhou Y, Zhang QB, Zhong HZ, Liu Y, Li J, Lv H, Jing JH. Rabbit model of extending knee joint contracture: progression of joint motion restriction and subsequent joint capsule changes after immobilization. J Knee Surg. 2020;33(1):15–21. doi:10.1055/s-0038-1676502.
- Trudel G, Uhthoff HK. Contractures secondary to immobility: is the restriction articular or muscular? An experimental longitudinal study in the rat knee. Arch Phys Med Rehabil. 2000;81(1):6–13. doi:10.1016/S0003-9993(00)90213-2.
- Sasabe R, Sakamoto J, Goto K, Honda Y, Kataoka H, Nakano J, Origuchi T, Endo D, Koji T, Okita M. Effects of joint immobilization on changes in myofibroblasts and collagen in the rat knee contracture model. J Orthop Res. 2017;35(9):1998–2006. doi:10.1002/jor.23498.
- Zhou HD, Trudel G, Goudreau L, Laneuville O. Knee joint stiffness following immobilization and remobilization: a study in the rat model. J Biomech. 2020;99:109471. doi:10.1016/j.jbiomech.2019.109471.
- Monument MJ, Hart DA, Befus AD, Salo PT, Zhang M, Hildebrand KA. The mast cell stabilizer ketotifen reduces joint capsule fibrosis in a rabbit model of post-traumatic joint contractures. Inflamm Res. 2012;61(4):285–292. doi:10.1007/s00011-011-0409-3.
- Hagiwara Y, Ando A, Chimoto E, Tsuchiya M, Takahashi I, Sasano Y, Onoda Y, Suda H, Itoi E. Expression of collagen types I and II on articular cartilage in a rat knee contracture model. Connect Tissue Res. 2010;51(1):22–30. doi:10.3109/03008200902859406.
- Zhang QB, Zhou Y, Zhong HZ, Liu Y. Effect of stretching combined with ultrashort wave diathermy on joint function and its possible mechanism in a rabbit knee contracture model. Am J Phys Med Rehabil. 2018;97(5):357–363. doi:10.1097/PHM.0000000000000873.
- Hao J, Ju H, Zhao S, Junaid A, Scammell-La Fleur T, Dixon IM. Elevation of expression of Smads 2, 3, and 4, decorin and TGF-β in the chronic phase of myocardial infarct scar healing. J Mol Cell Cardiol. 1999;31(3):667–678. doi:10.1006/jmcc.1998.0902.
- Wang RC, Wu GL, Dai TT, Lang YT, Chi ZC, Yang SL, Dong DS. Naringin attenuates renal interstitial fibrosis by regulating the TGF-β/Smad signaling pathway and inflammation. Exp Ther Med. 2021;21(1):1–12. doi:10.3892/etm.2020.9464.
- Velden JL, Alcorn JF, Guala AS, Badura EC, YM JH. C-Jun N-terminal kinase 1 promotes transforming growth factor-β1-induced epithelial-to-mesenchymal transition via control of linker phosphorylation and transcriptional activity of Smad3. Am J Respir Cell Mol Biol. 2011;44(4):571–581. doi:10.1165/rcmb.2009-0282OC.
- Engel ME, McDonnell MA, Law BK, Moses HL. Interdependent Smad and JNK signaling in transforming growth factor-β-mediated transcription. J Biol Chem. 1999;274(52):37413–37420. doi:10.1074/jbc.274.52.37413.
- Hashimoto S, Gon Y, Takeshita I, Matsumoto K, Maruoka S, Horie T. Transforming growth Factor-β1 induces phenotypic modulation of human lung fibroblasts to myofibroblast through a c-Jun-NH2-terminal kinase-dependent pathway. Am J Respir Crit Care Med. 2001;163(1):152–157. doi:10.1164/ajrccm.163.1.2005069.
- Zhang A, Ding G, Huang S, Wu Y, Pan X, Guan X, Chen R, Yang T. C-Jun NH2-terminal kinase mediation of angiotensin II-induced proliferation of human mesangial cells. Am J Physiol Renal Physiol. 2005;288(6):1118–1124. doi:10.1152/ajprenal.00220.2004.
- Meng XM, Nikolic-Paterson DJ, Lan HY. TGF-β: the master regulator of fibrosis. Nat Rev Nephrol. 2016;12(6):325–338. doi:10.1038/nrneph.2016.48.
- d’Agostino MC, Craig K, Tibalt E, Respizzi S. Shock wave as biological therapeutic tool: from mechanical stimulation to recovery and healing, through mechanotransduction. Int J Surg. 2015;24(Pt B):147–153. doi:10.1016/j.ijsu.2015.11.030.
- Rassweiler JJ, Knoll T, Kohrmann KU, McAteer JA, Lingeman JE, Cleveland RO, Bailey MR, Chaussy C. Shock wave technology and application: an update. Eur Urol. 2011;59(5):784–796. doi:10.1016/j.eururo.2011.02.033.
- Zissler A, Steinbacher P, Zimmermann R, Pittner S, Stoiber W, Bathke AC, Sanger AM. Extracorporeal shock wave therapy accelerates regeneration after acute skeletal muscle injury. Am J Sports Med. 2017;45(3):676–684. doi:10.1177/0363546516668622.
- Cui HS, Hong AR, Kim JB, Yu JH, Cho YS, Joo SY, Seo CH. Extracorporeal shock wave therapy alters the expression of fibrosis-related molecules in fibroblast derived from human hypertrophic scar. Int J Mol Sci. 2018;19(1):124. doi:10.3390/ijms19010124.
- Huang PP, Zhang QB, Zhou Y, Liu AY, Wang F, Xu QY, Yang F. Effect of radial extracorporeal shock wave combined with ultrashort wave diathermy on fibrosis and contracture of muscle. Am J Phys Med Rehabil. 2021;100(7):643–650. doi:10.1097/PHM.0000000000001599.
- Wang F, Li W, Zhou Y, Huang PP, Zhang QB. Radial extracorporeal shock wave reduces myogenic contracture and muscle atrophy via inhibiting NF-κB/HIF-1α signaling pathway in rabbit. Connect Tissue Res. 2022;63(3):298–307. doi:10.1080/03008207.2021.1920934.
- Zhang R, Zhang QB, Zhou Y, Zhang R, Wang F. Possible mechanism of static progressive stretching combined with extracorporeal shock wave therapy in reducing knee joint contracture in rats based on MAPK/ERK pathway. Biomol Biomed. 2023;23(2): 277–286. doi:10.17305/bjbms.2022.8152.
- Ogden JA, Toth-Kischkat A, Schultheiss R. Principles of shock wave therapy. Clin Orthop Relat Res. 2001;11(387):8–17. doi:10.1097/00003086-200106000-00003.
- Parsonage G, Filer AD, Haworth O, Nash GB, Rainger GE, Salmon M, Buckley CD. A stromal address code defined by fibroblasts. Trends Immunol. 2005;26(3):150–156. doi:10.1016/j.it.2004.11.014.
- Buckley CD, Pilling D, Lord JM, Akbar AN, Scheel-Toellner D, Salmon M. Fibroblasts regulate the switch from acute resolving to chronic persistent inflammation. Trends Immunol. 2001;22(4):199–204. doi:10.1016/S1471-4906(01)01863-4.
- Vi L, Gan BS, O’Gorman DB. The potential roles of cell migration and extra-cellular matrix interactions in Dupuytren’s disease progression and recurrence. Med Hypotheses. 2010;74(3):510–512. doi:10.1016/j.mehy.2009.10.009.
- Satish L, Johnson S, Wang JH, Post JC, Ehrlich GD, Kathju S. Chaperonin containing T-complex polypeptide subunit eta (CCT-eta) is a specific regulator of fibroblast motility and contractility. PLos One. 2010;5(4):e10063. doi:10.1371/journal.pone.0010063.
- Abdel MP, Morrey ME, Barlow JD, Kreofsky CR, An KN, Steinmann SP, Morrey BF, Sanchez-Sotelo J. Myofibroblast cells are preferentially expressed early in a rabbit model of joint contracture. J Orthop Res. 2012;30(5):713–719. doi:10.1002/jor.21588.
- Zhou YF, Yang K. Prevention of arthrofibrosis during knee repair by extracorporeal shock wave therapy: preliminary study in rabbits. Injury. 2019;50(3):633–638. doi:10.1016/j.injury.2019.01.054.
- Simplicio CL, Purita J, Murrell W, Santos GS, Dos Santos RG, Lana J. Extracorporeal shock wave therapy mechanisms in musculoskeletal regenerative medicine. J Clin Orthop Trauma. 2020;11(Suppl 3):S309–318. doi:10.1016/j.jcot.2020.02.004.
- Zhao JC, Zhang BR, Hong L, Shi K, Wu WW, Yu JA. Extracorporeal shock wave therapy with low-energy flux density inhibits hypertrophic scar formation in an animal model. Int J Mol Med. 2018;41(4):1931–1938. doi:10.3892/ijmm.2018.3434.
- Kondo Y, Nakano J, Sakamoto J, Kataoka H, Yokoyama S, Honda Y, Origuchi T, Yoshimura T, Okita M. Effects of prolonged stretching and thermotherapy on muscle contracture of immobilized rat soleus muscle. J Phys Ther Sci. 2012;24(6):541–547. doi:10.1589/jpts.24.541.
- Schlesinger SY, Seo S, Pryce BA, Tufa SF, Keene DR, Huang AH, Schweitzer R. Loss of Smad4 in the scleraxis cell lineage results in postnatal joint contracture. Dev Biol. 2021;470:108–120. doi:10.1016/j.ydbio.2020.11.006.