Abstract
Objective
Systemic lupus erythematosus (SLE or lupus) is an autoimmune disease characterized by B-cell dysfunction, production of autoantibodies, and immune complex formation. Lupus is overrepresented in females, indicating that sex hormones play a role in the pathophysiology. Treatment with a tissue-selective oestrogen complex (TSEC) containing conjugated oestrogens and the selective oestrogen receptor modulator bazedoxifene (BZA) protects against postmenopausal vasomotor symptoms and osteoporosis, but its impact on organ damage in lupus is not fully understood.
Method
We used ovariectomized MRL/lpr mice, treated with two different physiological doses of 17β-oestradiol-3-benzoate (E2), BZA, or TSEC (E2 plus BZA), to assess early and late B-cell development and to determine histological disease manifestations in the kidneys and salivary glands.
Results
TSEC treatment reduced the frequency of the pre-BI population in bone marrow to levels equivalent to treatment with physiological doses of E2 alone but did not affect any of the other examined B-cell populations. Our earlier studies indicated that TSEC treatment did not aggravate disease development in ovariectomized MRL/lpr mice, while protecting against trabecular bone loss. Here, we follow up on our previous study and show that neither ovariectomy alone nor TSEC treatment of ovariectomized MRL/lpr mice influenced perivascular lymphocyte infiltration to the kidneys or salivary glands.
Conclusion
TSEC does not aggravate a mouse model of lupus, when given in doses that protect against postmenopausal lupus-associated bone loss. This indicates that further investigations into TSEC as a treatment for osteoporosis or vasomotor symptoms in postmenopausal women with SLE are warranted.
Systemic lupus erythematosus (SLE or lupus) is a disease characterized by inflammation and multiorgan damage. This is associated with dysfunctional B cells, immune complex formation, and perivascular leucocyte infiltration (Citation1). The incidence of SLE is higher in women (female to male ratio 9:1) and peaks during the reproductive years (Citation2, Citation3), indicating an influence of sex hormones.
Oestrogen has major effects on the immune system, e.g. on B-cell development and function, the complement system, cytokine production, and type 1 interferon (IFN)-regulated genes (Citation4–8), which all are affected in lupus. However, studies exploring the role of exogenous oestrogens on disease activity in SLE are conflicting. Two studies reported that oral contraceptives did not increase the risk of flares (Citation9, Citation10), while a slight increase was shown after hormone replacement therapy (HRT) in postmenopausal patients (Citation11). In mouse models of lupus, treatment with supraphysiological doses of oestradiol (E2) resulted in worsening of the disease characteristics and decreased survival (Citation12, Citation13).
Osteoporosis is a common secondary complication in SLE (Citation14). Although oestrogen protects against both postmenopausal bone loss and vasomotor symptoms (VMS), the use of HRT is associated with an increased risk of severe side effects (Citation15, Citation16). The selective oestrogen receptor modulator (SERM) bazedoxifene (BZA) has beneficial effects on bone with little influence on endometrium or breast tissue (Citation17); however, it does not alleviate VMS (Citation18). A tissue-selective oestrogen complex (TSEC) containing the combination of conjugated oestrogens and BZA maintains the established benefits of oestrogen on bone and VMS, while minimizing the adverse effects (Citation19). This combination (Duavive®/Duavee®) is approved for the treatment of VMS and prevention of postmenopausal osteoporosis. However, the efficacy and safety of TSEC for treatment of bone loss or VMS in postmenopausal women with SLE are unknown.
MRL/MpJ-Faslpr/J (MRL/lpr) mice spontaneously develop a disease phenotype resembling human lupus, and we previously reported that ovariectomy (OVX) of MRL/lpr mice is a suitable model for studies of postmenopausal bone loss in lupus (Citation20). Furthermore, treatment with the TSEC (E2 + BZA) protected the skeleton without influencing disease markers measured in urine and serum in this model (Citation21). However, histopathological examinations of lupus target organs would further clarify whether TSEC is a safe alternative for the treatment of VMS and osteoporosis in postmenopausal women with SLE.
B-cell alterations are a hallmark of lupus disease, and SLE patients exhibit significant changes in the development, function, and distribution of B-cell populations (Citation22, Citation23). We reported previously that TSEC treatment induces the same effect on B lymphopoiesis as E2 alone, using healthy C57BL/6 mice (Citation24). However, the effect of TSEC on B-cell populations in experimental lupus remains to be examined.
In this study, we show that neither the removal of sex hormones by OVX nor treatment of ovariectomized MRL/lpr mice (OVX MRL/lpr mice) with TSEC influences the perivascular infiltration of immune cells to the kidneys or salivary glands, while it reduces the frequency of pre-BI cells in the bone marrow. Thus, TSEC does not aggravate disease development in lupus-prone mice, when given in doses that protect against lupus-associated osteoporosis.
Method
Mice
All work was approved by the animal ethical review board in Gothenburg (Dnr: 95-2015) and the study is reported in accordance with ARRIVE guidelines. Female MRL/MpJ-Faslpr/J (MRL/lpr) mice and control mice MRL/MpJ (MRL/++) mice were purchased from the Jackson Laboratory (Bar Harbor, ME, USA). They were kept in groups of three to 10 mice per cage under controlled environmental conditions and fed soya-free chow and tap water ad libitum. Mice were weighed and controlled for proteinuria and haematuria using dipsticks (Multistix® 7; Siemens Healthcare Diagnostic, Tarrytown, NY, USA) once or twice every week. Proteinuria and haematuria were scored and quantified according to the manufacturer’s instructions.
Ovariectomy (OVX)
Mice were ovariectomized by removing the ovaries through a midline incision of the skin followed by flank incisions of the peritoneum, and the skin was closed with metallic clips. The same procedure was performed for sham surgery, except for removal of the ovaries. The mice were sedated with isoflurane (Baxter Healthcare Corporation, Deerfield, IL, USA), and subcutaneous injections of Carprofen (Rimadyl; Orion Pharma Animal Health, Sollentuna, Sweden) were used as postoperative analgesia.
Experimental model 1: MRL/++ and MRL/lpr mice
MRL/++ and MRL/lpr mice were subjected to OVX or sham surgery (nine or 10 per group) at 8 weeks of age. The experiment was terminated at the age of 21 weeks. Owing to a weight loss of more than 10%, two MRL/lpr mice were terminated before the endpoint.
Experimental model 2: treatment with TSEC
17β-Oestradiol-3-benzoate (E2; Sigma Aldrich, St Louis, MO, USA), bazedoxifene (BZA; Pfizer, New York, NY, USA), or a combination [tissue-selective oestrogen complex (TSEC): E2 + BZA] was dissolved in miglyol oil (Vendico Chemical, Malmo, Sweden). Pure miglyol was used as a vehicle control (Veh). MRL/lpr mice underwent OVX or a sham operation at 6–7 weeks of age. Sham-operated mice were injected with Veh, and OVX mice with the different treatments five times per week for 3 weeks.
High and low doses of E2, BZA, and TSEC were used:
Low dose: 0.15 μg E2/mouse/day, 36 μg BZA/mouse/day, and TSEC (0.15 μg E2/mouse/day + 36 μg BZA/mouse/day). The initial number of mice was 10 per OVX group and five in the sham group. One mouse in the OVX Veh group died before the experimental endpoint. One mouse in the OVX Veh group was removed from all analyses owing to unsuccessful OVX.
High dose: 0.5 μg E2/mouse/day, 48 μg BZA/mouse/day, and TSEC (0.5 μg E2/mouse/day + 48 μg BZA/mouse/day). The initial number of mice was 11 per OVX group and six in the sham group. One mouse in the OVX Veh group was removed from all analyses owing to unsuccessful OVX.
Histological analysis
Formalin-fixed kidneys and mandibular salivary glands were embedded in paraffin, cut at a thickness of 4 µm, and stained with haematoxylin and eosin (H&E). Images were taken with a Nikon Eclipse 80i microscope and were subsequently fused for estimation of the whole organ area. Whole kidneys and mandibular salivary glands were scored blindly using a 0–4 scoring system (0: no cellular infiltration; 1: minimal; 2: mild; 3: moderate; 4: severe).
Flow cytometry analysis
Bone marrow cells and splenocytes were obtained and analysed by flow cytometry, as described previously (Citation24, Citation25). In brief, spleen was mashed through 70 μm cell strainers to obtain single-cell suspensions. Bone marrow cells were flushed from the femur using a syringe and phosphate-buffered saline (PBS). Cells from spleen and bone marrow were treated with Tris-buffered 0.83% NH4Cl solution (pH 7.4) for lysing erythrocytes and then resuspended in fluorescence-activated cell sorting (FACS) buffer [fetal bovine serum (FBS) 2%, ethylenediaminetetraacetic acid (EDTA) 2 mM in PBS]. Cells were counted using an automated cell counter (Sysmex Europe, Nordenstedt, Germany). Bone marrow cells were incubated with Fc-gamma receptor block [anti-CD16/32 antibodies; Becton Dickinson (BD) Pharmingen, Franklin Lakes, NJ, USA], followed by staining with fluorochrome-conjugated antibodies: CD19-PE (BD), B220-FITC (Biolegend, San Diego, CA, USA), c-kit (CD117)-APC (Biolegend), CD19-PE (BD), CD25-APC (BD), and IgM-PE (Southern Biotechnology Associates, Birmingham, AL, USA). Splenocytes (0.5 × 106) were stained for IgM-PE (Southern Biotechnology), B220-FITC (Biolegend), CD21-APC-Cy7 (Biolegend), CD23-PE-Cy7 (eBioscience, Vienna, Austria), and CD93-APC (eBioscience). Forward and side scatter gates were used to discriminate doublets and debris, and fluorescence minus one (FMO) was used as a control. The cells were investigated using FACSVerse (BD) and the data were analysed using FlowJo software (Three Star, Ashland, OR, USA).
Statistical analysis
Statistical analyses were performed using GraphPad Prism (Graph Pad Software, La Jolla, CA, USA). Groups were compared using one-way analysis of variance (ANOVA) followed by Sidak’s post-hoc analysis, unless stated otherwise. Histological score data were analysed using Kruskal–Wallis followed by Dunn’s multiple comparison test. Kaplan–Meier curves for proteinuria and haematuria versus time were analysed using the log-rank test. In Experimental model 1 (MRL/lpr and MRL/++ mice, sham and OVX surgery), post-hoc tests ware used for comparisons between groups. In Experimental model 2 (treatment with TSEC), sham-operated mice were only compared with vehicle-treated OVX mice, while all OVX groups, independent of treatment, were compared against each other. A p-value ≤ 0.05 was considered statistically significant.
Results
OVX does not affect perivascular infiltration in the kidneys and salivary glands of MRL/lpr mice
To investigate how the absence of sex steroids affects disease symptoms in experimental lupus, MRL/lpr mice and their congenic control strain (MRL/++) were subjected to OVX or sham surgery at the age of 8 weeks (). Successful OVX was confirmed by weighing the uterus at the end of the experiment (). The development of proteinuria and haematuria did not differ between Sham and OVX MRL/lpr mice, although a tendency towards earlier onset and higher frequency of both proteinuria and haematuria was shown for OVX MRL/lpr mice. Sham and OVX MRL/++ mice displayed no signs of proteinuria or haematuria (Online Supplementary Figure 1A, B).
Figure 1. Ovariectomy (OVX) does not affect perivascular leucocyte infiltration in the kidneys or salivary glands of MRL/lpr mice. MRL/lpr mice and their congenic control strain (MRL/++) were subjected to sham surgery or OVX at 8 weeks of age and perivascular infiltration was analysed in whole sections of kidney and salivary gland. The severity was scored according to a 0–4 grade scale. (A) Schematic overview of the experimental set-up; (B) uterus weight; (C) perivascular leucocyte infiltration score in the kidney and (D) representative images of kidney infiltrations; (E) perivascular leucocyte infiltration in the salivary gland and (F) representative images of salivary gland infiltrations. Each data point represents an individual animal. The bars indicate the median (B) or median (C and E). Statistical analysis: one-way ANOVA followed by Sidak’s post-hoc analysis (B) or Kruskal–Wallis followed by Dunn’s multiple comparison test (C and E). *p < 0.05, **p < 0.01, ****p < 0.0001. Scale bar = 200 µm.
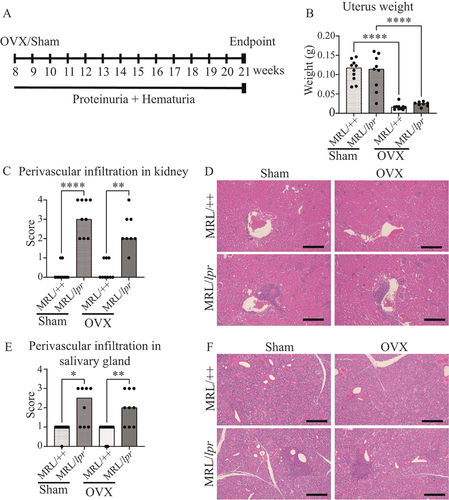
Kidney pathology was investigated by assessing the perivascular infiltration of inflammatory cells. MRL/lpr mice showed an increased score of infiltration in the kidney compared to the control MRL/++ strain in both sham-operated and OVX mice, but no differences in the level of infiltration between the Sham and OVX MRL/lpr groups were detected ().
Since the MRL/lpr mouse strain exhibit a phenotype similar to Sjögren’s syndrome, we examined perivascular infiltration in the mandibular salivary gland. MRL/lpr mice displayed an increased score of infiltration to the salivary glands compared to controls, but no differences in the level of infiltration between the Sham and OVX MRL/lpr groups were detected ().
Cells from bone marrow were isolated and analysed for T- and B-cell populations in Sham and OVX MRL/++ and MRL/lpr mice. MRL/lpr mice displayed an increased frequency of CD3 + positive T cells compared to the MRL/++ controls, whereas no difference was shown between Sham and OVX MRL/lpr mice (). No differences in the proportions of CD19+ B cells were found between the groups ().
Figure 2. Increased frequency of T cells in the bone marrow of MRL/lpr mice. MRL/lpr mice and their congenic control strain (MRL/++) were subjected to sham surgery or ovariectomy (OVX) at 8 weeks of age. Cells from the bone marrow was isolated and analysed by flow cytometry. Frequency of (A) T cells and (B) B cells. Each data point represents an individual animal. The bars indicate the mean. Statistical analysis: one-way ANOVA and Sidak’s post-hoc analysis. *p < 0.05, ***p < 0.001.
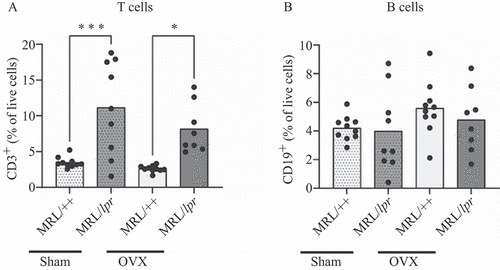
TSEC treatment does not influence perivascular infiltration in the kidneys and salivary glands of OVX MRL/lpr mice
Given the lack of difference in perivascular cell infiltration in the kidneys and salivary glands between Sham and OVX MRL/lpr mice, in combination with our recent publication showing that TSEC (E2 combined with BZA) can be used as a treatment for osteoporosis in a mouse model of postmenopausal lupus (Citation21), we evaluated the influence of TSEC treatment on kidney and salivary gland pathophysiology in the MRL/lpr lupus model.
Low dose of E2, BZA, or TSEC
MRL/lpr mice were subjected to either sham surgery or OVX. Sham-operated mice were treated with Veh and OVX mice were treated with Veh, low dose E2 (0.15 μg/mouse/day), BZA (36 μg/mouse/day), or TSEC (E2 + BZA). A schematic overview of the experimental set-up is shown in . Measurements of the uterus weights confirmed successful OVX surgery and showed that treatment of OVX mice with E2 restored the uterus weight to the same levels as in sham-operated mice, while OVX mice treated with BZA or TSEC had uterus weights comparable with the OVX Veh group [data shown in Nordqvist et al (Citation21)].
Figure 3. Tissue-selective oestrogen complex (TSEC) does not aggravate perivascular infiltration in the kidneys or salivary glands of OVX MRL/lpr mice. MRL/lpr mice were subjected to sham surgery or ovariectomy (OVX) at 6–7 weeks of age. In the low-dose experiment, sham-operated mice were treated with vehicle (Veh) and OVX mice were treated 5 days per week for 3 weeks with a low dose of 17β-oestradiol (E2; 0.15 μg/mouse/day), bazedoxifene (BZA; 36 μg/mouse/day), E2 + BZA (TSEC), or Veh. (A) Experimental set-up; perivascular cellular infiltration in (B) kidney and (C) salivary gland. In the high-dose experiment, sham-operated mice were treated with Veh and OVX mice were treated 5 days per week for 3 weeks with a high dose of E2 (0.5 μg/mouse/day), BZA (48 μg/mouse/day), TSEC, or Veh. (D) Experimental set-up; perivascular cellular infiltration in (E) kidney and (F) salivary gland. Each data point represents an individual animal. The bars indicate the median. Statistical analysis: Kruskal–Wallis followed by Dunn’s multiple comparison test.
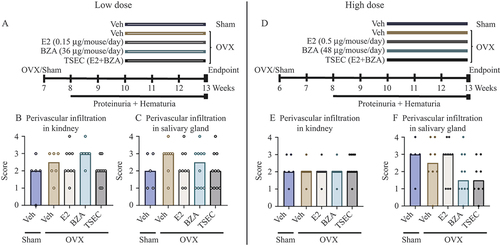
To determine whether TSEC treatment affected the perivascular infiltration of leucocytes in kidneys and salivary glands, these organs were sectioned and scored. The cellular infiltration in kidneys of MRL/lpr mice did not differ between Sham and OVX mice treated with Veh, or in response to any of the treatments in OVX mice (). In addition, histological scoring of perivascular infiltration in the salivary gland revealed no differences between the groups ().
High dose of E2, BZA, or TSEC
The experimental set-up was repeated with higher doses of E2 (0.5 μg/mouse/day), BZA (48 μg/mouse/day), or TSEC (E2 + BZA) (). Even though an increased dose of E2 was used, the uterus weight of the E2-treated mice did not exceed the uterus weight of the sham-operated mice. However, a small but significant increase in uterus weight was shown for the TSEC treatment group compared with the OVX Veh group, suggesting that BZA was not able to fully antagonize the high dose of E2 on the uterus [data shown in Nordqvist et al (Citation21)].
Perivascular kidney infiltration did not differ between any of the treatment groups after high-dose treatment of E2, BZA, or TSEC in MRL/lpr mice (). Moreover, scoring of cellular infiltration in the mandibular salivary gland revealed no differences between any of the groups ().
TSEC treatment reduces the frequency of pre-BI cells in the bone marrow of OVX MRL/lpr mice
E2, SERM, and TSEC have previously been shown to affect B-cell development in C57BL/6 mice (Citation24, Citation25). Therefore, we investigated B-cell subpopulations in the bone marrow and spleen from Sham and OVX MRL/lpr mice. Sham-operated mice were treated with Veh, and OVX mice with Veh and low or high doses of E2, BZA, or TSEC. A schematic overview of B-cell development in the bone marrow and B-cell differentiation in spleen is shown in .
Figure 4. Graphic overview of B-cell development and maturation in the bone marrow and spleen. The surface markers used to identify each B-cell subset are shown below the respective B-cell subtype. T1, transitional type 1 B cells; T2, transitional type 2 B cells; FO, follicular B cells; MZ, marginal zone B cells. The image was created using BioRender software.
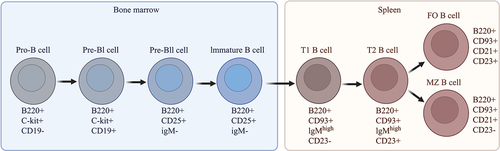
Low dose of E2, BZA, or TSEC
The two earliest populations of B cells during development in the bone marrow are the pro-B cells and pre-BI cells. Low-dose treatment with E2, BZA, or TSEC resulted in similar frequencies of pro-B cells between the groups (). Decreased frequencies of pre-BI cells were observed after treatment of OVX mice with E2, BZA, and TSEC compared to Veh treatment (), while no differences in the frequency of pre-BII or immature B cells were detected between the groups ().
Figure 5. Tissue-selective oestrogen complex (TSEC) treatment reduces the frequency of pre-BI cells in OVX MRL/lpr mice. MRL/lpr mice were subjected to sham surgery or ovariectomy (OVX) at 6–7 weeks of age. In the low-dose experiment, sham-operated mice were treated with Veh and OVX mice were treated 5 days per week for 3 weeks with a low dose of 17β-oestradiol (E2; 0.15 μg/mouse/day), bazedoxifene (BZA; 36 μg/mouse/day), E2 + BZA (TSEC), or Veh. B-cell populations in bone marrow were analysed by flow cytometry. Frequencies of (A) pro-B cells, (B) pre-BI cells, (C) pre-BII cells, and (D) immature B-cells. In the high-dose experiment, sham-operated mice were treated with Veh and OVX mice were treated 5 days per week for 3 weeks with a high dose of E2 (0.5 μg/mouse/day), BZA (48 μg/mouse/day), TSEC, or Veh. Frequencies of (E) pro-B cells, (F) pre-BI cells, (G) pre-BII cells, and (H) immature B cells. The results from four or five mice/per group in the low-dose E2, BZA, TSEC, and Veh groups are missing owing to technical issues. Each data point represents an individual animal. The bars indicate the mean. Statistical analysis: one-way ANOVA and Šídák’s multiple comparison test. ***p < 0.001, **p < 0.01, *p < 0.05.
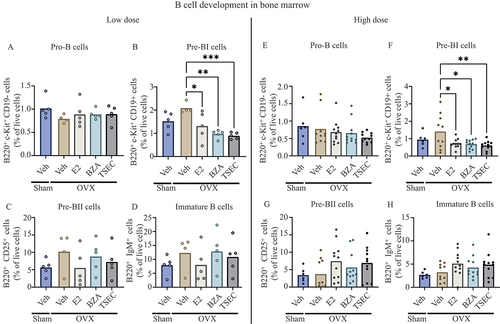
High dose of E2, BZA, or TSEC
B-cell development in bone marrow was also assessed after high-dose treatment of OVX mice with E2, BZA, and TSEC. As for the low-dose treatments, no differences in the frequency of pro-B cells were detected between any of the groups (), while treatment of OVX mice with E2, BZA, and TSEC resulted in reduced frequencies of pre-BI cells compared to Veh-treated mice (). No differences in the frequency of pre-BII or Immature B cells were detected between the groups ().
TSEC treatment does not affect B-cell differentiation in the spleen of OVX MRL/lpr mice
Low dose of E2, BZA, or TSEC
We analysed B-cell differentiation in the spleen after treatment of OVX mice with low-dose E2, BZA, and TSEC. Total spleen weights were similar between the treatment groups (). Transitional type 1 (T1) B cells were increased after OVX compared with Sham, and E2 treatment of OVX mice decreased T1 B cells to the same level as Sham. No differences after treatment of OVX mice with BZA or TSEC were detected (). Frequencies of transitional type 2 (T2) B cells as well as follicular (FO) B cells and marginal zone (MZ) B cells were similar between the groups ().
Figure 6. Treatment with low-dose E2 reduces the frequency of transitional type 1 B cells in OVX MRL/lpr mice. MRL/lpr mice were subjected to sham surgery or ovariectomy (OVX) at 6–7 weeks of age. In the low-dose experiment, sham-operated mice were treated with Veh and OVX mice were treated 5 days per week for 3 weeks with a low dose of 17β-oestradiol (E2; 0.15 μg/mouse/day), bazedoxifene (BZA; 36 μg/mouse/day), E2 + BZA (TSEC), or Veh. B-cell populations in spleen were analysed by flow cytometry. (A) Total spleen weight and frequencies of (B) transitional type 1 (T1) B cells, (C) transitional type 2 (T2) B cells, (D) follicular (FO) B cells, and (E) marginal zone (MZ) B cells. In the high-dose experiment, sham-operated mice were treated with Veh and OVX mice were treated 5 days per week for 3 weeks with a high dose of E2 (0.5 μg/mouse/day), BZA (48 μg/mouse/day), TSEC, or Veh. B-cell populations in spleen were analysed by flow cytometry. (F) Total weight of spleen and frequencies of (G) T1 B cells, (H) T2 B cells, (I) FO B cells, and (J) MZ B cells. Each data point represents an individual animal. The bars indicate the mean. Statistical analysis: one-way ANOVA and Šídák’s multiple comparison test. *p < 0.05.
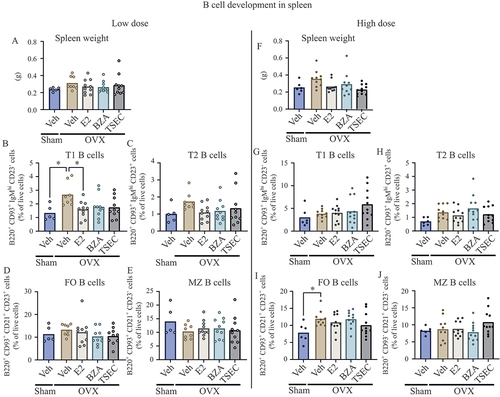
High dose of E2, BZA, or TSEC
B-cell differentiation in spleen was also investigated after treatment of OVX mice with high-dose E2, BZA, and TSEC. Total spleen weights did not differ between the treatment groups (). In contrast to the low-dose experiment, similar levels of T1 B cells were observed between Sham and OVX mice treated with Veh or E2 (). Furthermore, as shown in , the frequencies of T2 B cells did not differ between the groups. In contrast to the low-dose experiment, FO B cells were increased after OVX compared with Sham, but no differences were found in response to treatments (). Furthermore, treatment with high-dose E2, BZA, or TSEC did not change the frequency of MZ B cells ().
Discussion
In this study we follow up on our previous work which indicated that treatment with TSEC (E2 + BZA) does not worsen lupus disease characteristics in OVX MRL/lpr mice (Citation21), by further investigating cellular and histological mechanisms. We present encouraging data showing that treatment of OVX MRL/lpr mice with TSEC does not influence the infiltration of immune cells in the kidneys and salivary glands, although the frequency of pre-BI cells is reduced compared to vehicle-treated mice.
There is a clear female predominance in SLE. X-chromosome-linked genes have been shown to contribute to disease pathophysiology (Citation26), but the fact that the female prevalence peaks during child-bearing years also points towards a hormonal influence on the development of the disease. However, the role of oestrogen in SLE is complex, as the risk of flares is increased during pregnancy (Citation27), while the use of third generation oral contraceptives is well tolerated in patients with stable disease (Citation9, Citation10).
A previous study from our group revealed that depletion of sex hormones by OVX did not alter the disease development in MRL/lpr mice, assessed by proteinuria, haematuria, and disease-specific and inflammatory serum markers (urea, interleukin-6, immunoglobulins G and M, and anti-double-stranded DNA) compared to sham-operated mice (Citation21). Here, we confirm this finding and show no differences in perivascular cell infiltrations in the kidneys and salivary glands between Sham and OVX MRL/lpr mice. This is also in line with research conducted in lupus-prone NZM2410 or NZB/NZW F1 mice, where OVX did not affect the survival of the mice (Citation28, Citation29).
We further show that treatment of OVX MRL/lpr mice with two different doses of TSEC (E2 + BZA) did not affect the perivascular infiltration of inflammatory cells in the kidneys and salivary glands compared to vehicle controls. This is in line with the results from our previous study showing no differences in proteinuria, haematuria, or serum markers in response to TSEC treatment (Citation21). The effect of treatment with E2 or BZA alone did not differ from treatment with TSEC on typical lupus characteristics in the kidneys and salivary glands. However, postmenopausal treatment with E2 is not recommended owing to the increased risk of breast and endometrial cancer (Citation15), and BZA lacks effects on VMS (Citation18). As SLE is associated with both early menopause and an increased risk of developing osteoporosis (Citation30), treatment with TSEC could be beneficial for the prevention of bone loss and alleviation of VMS. Our results encourage further investigations into TSEC as a treatment option in postmenopausal lupus patients.
Alterations in B cells are associated with lupus disease development. SLE patients exhibit significant changes in the development and distribution of B-cell populations due to defects in immunological checkpoints during B-cell development (Citation22, Citation23). Oestrogen is known to influence B lymphopoiesis by inhibiting the differentiation from pro-B cells to pre-B cells in the bone marrow (Citation25, Citation31). Oestrogen also influences later stages of B-cell development in the spleen, where E2 treatment was shown to reduce the T1 B-cell population and increase the number of MZ B cells (Citation24, Citation25). We showed previously that TSEC induces the same effect on B-cell development as treatment with E2 alone in healthy C57BL/6 mice (Citation24), but B-cell development after TSEC treatment has not previously been investigated in experimental postmenopausal lupus. This study reveals reduced levels of pre-BI cells in OVX MRL/lpr mice after TSEC treatment. Although both the early and late phases of B-cell development in the bone marrow and spleen were investigated, no other populations were affected. In addition, we showed in our previous publication that immunoglobulin production is unaffected by TSEC treatment (Citation21). In contrast, TSEC treatment of healthy C57BL/6 mice also resulted in reductions in pre-BII, immature, and transitional B-cell populations, as well as increased MZ B cells in response to TSEC (Citation24). The differences between the studies are probably due to the dissimilar settings between healthy C57BL/6 and lupus-prone MRL/lpr mice. The bone marrow of mice with lupus displays an increased number of apoptotic cells and IFN-producing inflammatory cells, such as T cells, which can influence B-cell development (Citation32, Citation33). This is in line with the result from this study showing that the MRL/lpr mice have an increase in T cells in bone marrow compared to MRL/++ controls. There is a large spread between the individual MRL/lpr mice in most of the studied B-cell populations that may conceal possible effects of hormone treatments. Future studies in which B-cell development is investigated at different stages of lupus disease progression would further clarify the picture.
The dose of E2 used is crucial for the outcome of experimental studies in mouse models of lupus, as treatment with E2 in NZB/NZW F1 mice worsened the lupus disease (Citation12, Citation29). Notably, in those studies, silastic tubes containing 6–7 mg of E2 were used, resulting in considerably higher hormone serum levels than the doses given to mice in this study (0.15 and 0.5 µg/day). Administration of such high doses escalates the risk of urinary retention (Citation34) and subsequently accelerates the development of renal pathology. In this study, none of the E2 doses used led to uterus weights exceeding the uterus weights of sham-operated mice. Thus, the MRL/lpr mice were given physiological doses of E2 alone or in combination with BZA.
The study has some limitations. The treatment period lasted for 3 weeks, and to evaluate the effects of long-term use of TSEC in postmenopausal lupus, longer treatments are needed. However, although the MRL/lpr strain is suitable for studying lupus disease because of the resemblance to the human disease, severe symptoms such as pronounced lymphadenopathy prevented us from letting the mice age too far. A strain with a slower disease development may be more suitable for these studies. Furthermore, a broader and more specific panel of SLE disease activity markers could have been used to provide additional information about the effects of treatments on lupus disease activity.
In summary, treatment with TSEC in doses that were previously shown to protect against bone loss in this animal model of postmenopausal lupus resulted in a reduced frequency of pre-BI cells in bone marrow. Furthermore, the data from our previous study, which indicated that TSEC treatment does not worsen lupus disease characteristics, were strengthened by the fact that perivascular infiltrations to the kidneys or salivary glands were not affected by TSEC.
Conclusion
The results from this study indicate that TSEC treatment may be well tolerated in lupus, and further investigations into TSEC as a treatment option for osteoporosis and VMS in postmenopausal women with SLE are warranted.
Ethics approval
All work was approved by the animal ethical review board in Gothenburg (ethical permit number: 95-2015).
Authors’ contributions
CD, JN, HC, and UI planned the experiments; CD, JMS, JN, and CE performed the experiments. CD, JMS, HC, and UI analysed and interpreted the results of the experiments. CD, HC, and UI drafted the manuscript. CD, JMS, JN, CE, HC, and UI edited the manuscript. All authors approved the final version of the manuscript.
Supplemental Material
Download TIFF Image (15.1 MB)Acknowledgements
We thank Alicia Maria del Carpio Pons for excellent technical assistance. was created using BioRender software.
Disclosure statement
No potential conflict of interest was reported by the authors.
Data availability statement
The data supporting the conclusions of this study are available within the article.
Supplementary material
Supplemental data for this article can be accessed online at https://doi.org/10.1080/03009742.2023.2251753
Additional information
Funding
References
- Wahren-Herlenius M, Dörner T. Immunopathogenic mechanisms of systemic autoimmune disease. Lancet 2013;382:819–31.
- Weckerle CE, Niewold TB. The unexplained female predominance of systemic lupus erythematosus: clues from genetic and cytokine studies. Clin Rev Allergy Immunol 2011;40:42–9.
- Christou EAA, Banos A, Kosmara D, Bertsias GK, Boumpas DT. Sexual dimorphism in SLE: above and beyond sex hormones. Lupus 2019;28:3–10.
- Singh RP, Hahn BH, Bischoff DS. Interferon genes are influenced by 17β-estradiol in SLE. Front Immunol 2021;12:725325.
- Medina KL, Kincade PW. Pregnancy-related steroids are potential negative regulators of B lymphopoiesis. Proc Natl Acad Sci U S A 1994;91:5382–6.
- Erlandsson MC, Jonsson CA, Lindberg MK, Ohlsson C, Carlsten H. Raloxifene- and estradiol-mediated effects on uterus, bone and B lymphocytes in mice. J Endocrinol 2002;175:319–27.
- Young NA, Wu LC, Burd CJ, Friedman AK, Kaffenberger BH, Rajaram MV, et al. Estrogen modulation of endosome-associated toll-like receptor 8: an IFNα-independent mechanism of sex-bias in systemic lupus erythematosus. Clin Immunol 2014;151:66–77.
- Sundstrom SA, Komm BS, Ponce-de-Leon H, Yi Z, Teuscher C, Lyttle CR. Estrogen regulation of tissue-specific expression of complement C3. J Biol Chem 1989;264:16941–7.
- Petri M, Kim MY, Kalunian KC, Grossman J, Hahn BH, Sammaritano LR, et al. Combined oral contraceptives in women with systemic lupus erythematosus. N Engl J Med 2005;353:2550–8.
- Sánchez-Guerrero J, Uribe AG, Jiménez-Santana L, Mestanza-Peralta M, Lara-Reyes P, Seuc AH, et al. A trial of contraceptive methods in women with systemic lupus erythematosus. N Engl J Med 2005;353:2539–49.
- Buyon JP, Petri MA, Kim MY, Kalunian KC, Grossman J, Hahn BH, et al. The effect of combined estrogen and progesterone hormone replacement therapy on disease activity in systemic lupus erythematosus: a randomized trial. Ann Intern Med 2005;142:953–62.
- Roubinian JR, Talal N, Greenspan JS, Goodman JR, Siiteri PK. Effect of castration and sex hormone treatment on survival, anti-nucleic acid antibodies, and glomerulonephritis in NZB/NZW F1 mice. J Exp Med 1978;147:1568–83.
- Talal N. Natural history of murine lupus. Modulation by sex hormones. Arthritis Rheum 1978;21:S58–63.
- Sen D, Keen RW. Osteoporosis in systemic lupus erythematosus: prevention and treatment. Lupus 2001;10:227–32.
- Rossouw JE, Anderson GL, Prentice RL, LaCroix AZ, Kooperberg C, Stefanick ML, et al. Risks and benefits of estrogen plus progestin in healthy postmenopausal women: principal results from the Women’s Health Initiative randomized controlled trial. JAMA 2002;288:321–33.
- Beral V, Bull D, Reeves G. Endometrial cancer and hormone-replacement therapy in the Million Women Study. Lancet 2005;365:1543–51.
- Silverman SL, Christiansen C, Genant HK, Vukicevic S, Zanchetta JR, de Villiers TJ, et al. Efficacy of bazedoxifene in reducing new vertebral fracture risk in postmenopausal women with osteoporosis: results from a 3-year, randomized, placebo-, and active-controlled clinical trial. J Bone Mineral Res 2008;23:1923–34.
- Pickar JH, Lavenberg J, Pan K, Komm BS. Initial investigation into the optimal dose ratio of conjugated estrogens and bazedoxifene: a double-blind, randomized, placebo-controlled phase 2 dose-finding study. Menopause 2018;25:273–85.
- Pickar JH, Boucher M, Morgenstern D. Tissue selective estrogen complex (TSEC): a review. Menopause 2018;25:1033–45.
- Nordqvist J, Lagerquist MK, Grahnemo L, Koskela A, Islander U, Carlsten H. Osteoporosis in a murine model of postmenopausal lupus. Lupus 2020;29:58–66.
- Nordqvist J, Engdahl C, Scheffler JM, Gupta P, Gustafsson KL, Lagerquist MK, et al. A tissue-selective estrogen complex as treatment of osteoporosis in experimental lupus. Lupus 2022;31:143–54.
- Wardowska A, Komorniczak M, Skoniecka A, Bułło-Piontecka B, Lisowska KA, Dębska-Ślizień MA, et al. Alterations in peripheral blood B cells in systemic lupus erythematosus patients with renal insufficiency. Int Immunopharmacol 2020;83:106451.
- Iwata S, Tanaka Y. B-cell subsets, signaling and their roles in secretion of autoantibodies. Lupus 2016;25:850–6.
- Nordqvist J, Bernardi A, Islander U, Carlsten H. Effects of a tissue-selective estrogen complex on B lymphopoiesis and B cell function. Immunobiology 2017;222:918–23.
- Bernardi AI, Andersson A, Grahnemo L, Nurkkala-Karlsson M, Ohlsson C, Carlsten H, et al. Effects of lasofoxifene and bazedoxifene on B cell development and function. Immun Inflamm Dis 2014;2:214–25.
- Youness A, Miquel CH, Guéry JC. Escape from X chromosome Inactivation and the female predominance in autoimmune diseases. Int J Mol Sci 2021;22:1114.
- Eudy AM, Siega-Riz AM, Engel SM, Franceschini N, Howard AG, Clowse MEB, et al. Effect of pregnancy on disease flares in patients with systemic lupus erythematosus. Ann Rheum Dis 2018;77:855–60.
- Scott JL, Wirth JR, Eudaly J, Ruiz P, Cunningham MA. Complete knockout of estrogen receptor alpha is not directly protective in murine lupus. Clin Immunol 2017;183:132–41.
- Roubinian JR, Papoian R, Talal N. Androgenic hormones modulate autoantibody responses and improve survival in murine lupus. J Clin Invest 1977;59:1066–70.
- Ceccarelli F, Orefice V, Perrone G, Pirone C, Perricone C, Truglia S, et al. Premature ovarian failure in patients affected by systemic lupus erythematosus: a cross-sectional study. Clin Exp Rheumatol 2020;38:450–4.
- Smithson G, Medina K, Ponting I, Kincade PW. Estrogen suppresses stromal cell-dependent lymphopoiesis in culture. J Immunol 1995;155:3409–17.
- Park JW, Moon SY, Lee JH, Park JK, Lee DS, Jung KC, et al. Bone marrow analysis of immune cells and apoptosis in patients with systemic lupus erythematosus. Lupus 2014;23:975–85.
- Palanichamy A, Bauer JW, Yalavarthi S, Meednu N, Barnard J, Owen T, et al. Neutrophil-mediated IFN activation in the bone marrow alters B cell development in human and murine systemic lupus erythematosus. J Immunol 2014;192:906–18.
- Pearse G, Frith J, Randall KJ, Klinowska T. Urinary retention and cystitis associated with subcutaneous estradiol pellets in female nude mice. Toxicol Pathol 2009;37:227–34.