Abstract
We have analysed mitochondrial DNA and shell morphometric data from all species and subspecies of Placostylus land snails in New Zealand. These subspecies were originally described on the basis of shell morphology. The genetic data show that each of the three species P. bollonsi, P. hongii and P. ambagiosus are well differentiated and monphyletic for mitochondrial DNA, consistent with their species status. Variation among subspecies within each of the three species was minimal with sharing of haplotypes among subspecies. Morphometric analysis of shell shape characters shows large amounts of environmental plasticity. On the basis of these data we have synonymised all subspecies within each of the three Placostylus species. Consequently, the New Zealand fauna now consists of three Placostylus species, P. ambagiosus, P. hongii and P. bollonsi, and no subspecies. We recommend conservation of multiple populations within each species to ensure preservation of genetic variation.
Introduction
Land snails in the genus Placostylus Beck, 1837 (Bulimulidae), known in New Zealand as flax snails, are among the most familiar members of New Zealand's unique invertebrate fauna; although they also occur in Lord Howe Island, New Caledonia, and some other western Pacific Islands such Fiji, Vanuatu and the Solomons (Ponder et al. Citation2003; Trewick et al. Citation2009). Two of the three species in New Zealand, Placostylus bollonsi Suter, 1908, and Placostylus ambagiosus Suter, 1906, currently include described subspecies whereas the third, Placostylus hongii Lesson, 1830, does not (). The New Zealand species of Placostylus have long been considered threatened (e.g. Powell Citation1947; Ramsay & Gardner Citation1977; Ogle Citation1979) and are now legally protected under the New Zealand Wildlife Act 1953 (Seventh Schedule). Placostylus hongii and one population of P. bollonsi are considered range restricted whereas the other four populations of P. bollonsi and all but one population of P. ambagiosus are ranked in the two highest threat classifications (Nationally Critical or Nationally Endangered) by Hitchmough et al. (Citation2007).
Table 1 Numbers of snail shells used for analysis from each location together with subjective habitat scores for vegetation and apparent moisture at each site.
The three species of Placostylus in New Zealand were described using morphological characteristics of the shell including apical angles and ratios of height to maximum diameter, numbers of tubercles in the aperture, and colours of the periostracum and internal aperture. Suter (Citation1908) also added some details of the radula and reproductive organs for P. bollonsi. Three subspecies of P. bollonsi and 11 subspecies of P. ambagiosus were distinguished by Powell (Citation1938, Citation1947, Citation1948, Citation1951) from differences in shell morphology and since then 15 new populations of P. ambagiosus have been located but their taxonomic status is unknown (Brook Citation2002).
The three New Zealand species of Placostylus are relatively large (50.0–113.5 mm) and mostly live on the ground where they feed on leaves that have fallen from broadleaf plants (Parrish et al. Citation1995, Powell Citation1979). Only the hatchling snails of P. hongii and occasionally those of P. ambagiosus are briefly arboreal whereas some species and closely related species overseas are arboreal as older juveniles or adults (e.g. Solem 1959; Goodacre & Wade Citation2001; Heller Citation2001; Townsend et al. Citation2005; Brescia et al. Citation2008). All populations of Placostylus in New Zealand are highly restricted geographically—they occur either on islands or small areas of the mainland. Many also survive in habitat that has been modified by humans. The islands where they are found now have no mammals whereas their locations on the mainland are susceptible to predation from introduced mammals such as rodents, pigs and hedgehogs (Parrish et al. Citation1995).
Placostylus bollonsi originally occurred on three islands in the Three Kings group, 56 km to the north of the New Zealand mainland. Three subspecies of P. bollonsi were described: P. b. arbutus, P. b. bollonsi and P. b. caperatus. All three occupy different areas of Manawatawhi/Great Island and P. b. bollonsi also occurs on North East Island and West Island. The wider distribution of P. b. bollonsi was possibly due to translocation between the islands by Māori who once lived there (Powell Citation1948, Citation1951; Climo Citation1973). In addition, P. b. arbutus was translocated from Manawatawhi/Great Island to South West Island, which appeared to lack Placostylus, in 2003 to provide prey for the endangered carnivorous snail Rhytidarex buddlei (Rhytitidae) (Brook & Whaley Citation2008). Placostylus ambagiosus occurs naturally in the Te Paki/North Cape region at the northern end of the North Island, a well known area of endemism (e.g. Druce et al. Citation1979; Goulstone et al. Citation1993; de Lange et al. Citation2003; Barker Citation2005; Larochelle & Larivière Citation2005; Marshall & Barker Citation2007; Chapple et al. Citation2008; Winterbourn Citation2009; Buckley & Bradler Citation2010; Buckley et al. Citation2010). Placostylus hongii occurs along the east coast of Northland between Whangaroa and Whangarei Heads and on some of the nearby islands (Powell Citation1979). Both North Island species survive in remnant populations occupying small areas except for P. hongii which also occurs in large numbers on 10 islands in the Poor Knights Islands (Brook & McArdle Citation1999; Brook Citation2002). There are suggestions that some of the populations of P. hongii snails on islands may have resulted from being moved by Māori (Great Barrier Island, Mokohinau Islands, Marotere Islands and Poor Knights Islands) but this has not been confirmed (Climo 1973; Powell 1975, 1979; Hayward & Brook Citation1981; Triggs & Sherley Citation1993; Brook Citation1999). Brook & McArdle (Citation1999) concluded that P. hongii are native to the Poor Knights Islands and probably Mokohinau Islands and were taken from them to other islands and the mainland by Māori. They expressed doubt about whether the Marotere Islands (Hen and Chickens Islands) populations were endemic.
Penniket (Citation1981), Brook & Laurenson (Citation1992) and Sherley (Citation1996) examined the subspecific classifications of the New Zealand species of Placostylus in relation to morphometric analyses. Penniket (Citation1981) showed that in P. ambagiosus the shell height and shape were related to habitat type and exposure. He found, using discriminant analysis and correlation analysis, that the snails fell into three main groups that showed a progressive change in relation to distance from the sea and shelter with the smallest shells being in exposed situations near the sea and the largest being in sheltered inland forest. Penniket (Citation1981) also reported that the shells of P. hongii snails from Peach Cove and the Poor Knights Islands showed no differences from the shells of P. ambagiosus snails from similar forest and suggested that ‘P. hongii may be more closely related to P. ambagiosus than the separate specific names suggest’ (p. 8). Brook & Laurenson (Citation1992) found that shells of P. b. arbutus, P. b. bollonsi and P. b. caperatus on Manawatawhi/Great Island were morphologically distinct thus supporting Powell (Citation1948), but that the shell morphologies of P. b. bollonsi on West Island and North East Island differed from those of P. b. bollonsi on Manawatawhi/Great Island. Brook & Laurenson (Citation1992) also found, using principal component analysis (PCA), that the shell morphologies had remained stable within each population over the period between 1945–46 and 1982–83, except that there was some indication that morphological variation may have increased slightly in the P. b. bollonsi and P. b. arbutus populations over that time. Sherley (Citation1996) also used PCA and reported that only the West Island population of P. bollonsi was distinct from the other four populations on the Three Kings Islands, and that the 12 populations of P. ambagiosus formed three coarse and overlapping groups. Shells from one population of P. hongii (Whangaruru) were grouped with one of the groups of P. ambagiosus.
No subspecies of P. hongii have been described but Brook & McArdle (Citation1999) examined the shells of snails from all known sites including where they were extinct. They found both considerable variation and much overlap in shell morphology between populations. This variation was in part related to local environmental factors of substratum type and vegetation type. They suggested the latter was probably related to microclimatic differences between low shrubland and forest.
The first genetic study of New Zealand Placostylus using allozyme electrophoresis by Triggs & Sherley (1993) provided support for three species but found little differentiation between the three subspecies of P. bollonsi. They found extensive genetic differentiation between populations of P. ambagiosus snails that could be related to isolation by geological barriers and geographic distance and that these populations fell into six genetically distinct groups. On the basis of this genetic information Brook (Citation2003) subsequently considered that ‘there seems to be little taxonomic justification for continued recognition of formal subspecies within P. bollonsi’ (p. 8).
The phylogenetic study of mitochondrial cytochrome oxidase subunit I (COI) sequences by Ponder et al. (Citation2003) showed that the New Zealand Placostylus do not form a monophyletic group, that P. bollonsi was sister to all the species they sampled, and that P. ambagiosus and P. hongii were sister taxa to one another and related to P. fibratus from New Caledonia. The same result was achieved by Trewick et al. (Citation2009) who analysed COI and mitochondrial large subunit ribosomal RNA sequences. This result was consistent with the sub generic split in the genus that places P. bollonsi in the subgenus Basileostylus and P. ambagiosus and P. hongi in the subgenus Maoristylus. Ponder et al. (Citation2003) used few samples from the New Zealand species of Placostylus but did report that at least the north-east population of P. bollonsi may be distinct from other populations of P. bollonsi on Manawatawhi/Great Island. Their results from four populations of P. ambagiosus (P. a. annectens and P. a. watti vs. P. a. lesleyae and Tirikawa) support the primary genetic division of P. ambagiosus populations reported by Triggs & Sherley (1993).
The existence of described subspecies within P. ambagiosus led to each isolated population being considered a distinct entity for conservation purposes with the result that considerable conservation effort was spent on them (e.g. Parrish et al. Citation1995). However, shell-shape and shell-height of snails are known to be ‘notoriously’ plastic (Hemp & Bertness Citation1984; Goodfriend Citation1986) with differences between local populations of snails being the result of genetic differences, local environmental effects or a combination of both (e.g. Goodfriend Citation1986; Wilber & Owen 1964; Welter-Schultes Citation2000; Estebenet & Matin 2003; Anderson et al. Citation2007; Madec & Bellido Citation2007). Previous authors showed that this may be the case in Placostylus (Brook & McArdle 1999; Brook Citation2003). Thus, we undertook a genetic analysis of these snails to confirm their specific status and determine whether the subspecies were justified. We also undertook a preliminary investigation using multivariate statistical techniques to determine whether moisture and vegetation type might affect shell morphology. The overall aim was to determine whether the conservation effort directed towards these species could be more efficiently and effectively applied by concentrating on a reduced number of populations chosen to capture the species’ genetic variation and evolutionary potential. We thereby address suggestions made long ago by Choat & Scheil (1980) and Penniket (Citation1981).
Previous authors have also noted the need for translocations and captive-rearing experiments to examine the possibility that environmental factors affect the size and shape of these snails (Choat & Scheil 1980; Penniket Citation1981). Brook & McArdle (Citation1999) reported varying results in the difference between shells of a translocated population of P. hongii snails on Motuhoropapa Island and snails from the source population on Archway Island, Poor Knights Islands. However, although the habitats where the snail occur on these islands were different—they were mostly found in shrubland on Archway Island whereas they were released in forest on Motuhoropapa Island—the results were inconclusive (Brook & McArdle Citation1999). We now have data from two translocations of P. a. paraspiritus, and from captive-rearing of P. a. paraspiritus and P. hongii snails (Sherley Citation1990; Stringer & Grant Citation2007) as well as morphometric data and genetic samples from most of the populations that are still extant for all three species of Placostylus in New Zealand. Hence it is timely to publish results of both the morphological and genetic differences between the various species and populations of these snails together with morphological differences between the source populations and those that were captive-reared or translocated. Our aims were:
-
to confirm the specific status of the three New Zealand species of Placostylus;
-
to examine whether the subspecies described for P. bollonsi and P. ambagiosus are valid or whether their morphological differences in shell shape and height are due to responses to local factors; and
-
to determine suitable conservation units that account for the genetic variation within each species and any morphological differences if appropriate.
Methods
Taxon sampling
New Zealand species and subspecies of Placostylus were sampled from most of the locations where they are known to be extant (). Brief descriptions of the localities together with some habitat information are given by Brook & Laurenson (1992), Brook & McArdle (Citation1999), and Brook (Citation2002) and localities where samples were taken for DNA analysis are given in Appendix 1. No measurements were taken from P. hongii snails on some of the small islets in the Poor Knights Islands group, Taranga Kawau, Unuwhao, Ngaruariki Stream, Kauaetewhakapeke valley, Poroiki Hill, and Rangiora Bay (Brook & McArdle Citation1999; Brook Citation2002). The measurement data used by Sherley (Citation1996) were included and we took new measurements from some of the same shells used by Brook & McArdle (Citation1999) including those in the G.R. Parrish collection (Gut Rock off Fanal Island [Mokohinau Islands], the island off Cape Wiwiki, Orokawa Bay, Aorangi Island, and Motuhoropapa Island). Additional shell measurements were taken from live snails found on a first encounter basis, and the snails were then replaced where they were found. Measurements from laboratory-reared snails of P. a. paraspiritus and P. hongii were taken before they were released. The parent population of the latter species was Aorangi Island, Poor Knights Islands.
Shell measurements
Nine different shell measurements were taken with vernier calipers (±0.02 mm) (). The apertures of all shells measured had well-developed thickened edges indicating further shell growth had ceased except for the thickened edge itself which increases in thickness <1 mm per year (Penniket Citation1981; Stringer et al. Citation2004; Stringer & Grant Citation2007). Samples of shells were obtained from 37 locations (). The empty shells and the shells of live snails were usually measured in the field and returned. Some of the empty shells were the same as those used previously by Sherley (Citation1996) and Brook & McArdle (Citation1999).
Morphometric analyses
Means and 95% confidence intervals for shell height were determined for snails from each location or subspecies. These are shown graphically so that differences between them can be observed by inspection. An optimisation classification tree analysis was used to compute the mean shell height based on species and the habitat variables of moisture level and vegetation type. For this analysis the vegetation types were reduced to three by combining similar vegetations in order to reduce the number of variables for estimation. Thus ‘shrubland’ included vegetation types 1, 2 and 3 in , ‘scrub’ was 4, and ‘forest’ was 5 and 6 combined.
Comparisons between species, moisture levels and vegetation types were investigated using a linear mixed effects model with maximum likelihood estimation in R (version 2.9.2) with location or subspecies as a random effect to account for repeated measures within location using the same habitat levels as in the classification tree analysis. Based on the result P. ambagiosus and P. hongii were not significantly different and were considered to be the same for future analysis. Moisture levels of dry and normal were similarly considered to be the same for further analysis because they did not differ significantly, while scrub and forest were amalgamated during modelling using the Akaike Information Criterion.
Multivariate analysis of shell-shape were done after standardisation (mean 0, standard deviation [SD] 1) to emphasise differences in shape rather than size. Wilks-Shapiro tests (using R) indicated that multivariate normality was unlikely (P. ambagiosus W=0.9085, p=3×10−16; P. bollonsi W=0.8916 p=5×10−8; P.hongii W=0.9374, p=2×10−10) so differences in shell-shape were primarily analysed using non-metric multidimensional scaling (NMS) with PC-ORD (v5, MjM Software, Oregon, USA). The analysis was done using the ‘auto’ setting in PC-ORD (100 iterations, 50 runs of both real and randomised data, Monte Carlo test) and the Euclidean distance measure (McCune & Grace Citation2002). A second analysis was done using principal component analysis (PCA). This was based on the correlation matrix and done on the normalised data set, relaxing the assumption of multivariate normality for descriptive purposes because bivariate plots of the variables (not shown) within each species showed they were approximately linear (McCune & Grace Citation2002). PCA was also chosen because it had been used to analyse measurements of some of these snails in the past (Brook & Laurenson Citation1992; Sherley Citation1996; Brook & McArdle Citation1999). Differences between the shell-shapes of species and subgroups of the snails were compared using a multiple response permutation procedure (MRPP) in PC-ORD using the results from NMS.
The overall vegetation of the habitat where the snails were found was scored subjectively by authors RP and GS independently of the analyses () as:
-
shrubs separated by low vegetation on dry ultramaffic soil;
-
shrubs on small rocky islets;
-
shrubs separated by tall sedges and/or grasses;
-
mixed scrub/forest;
-
forest on islands; and
-
mainland forest.
DNA extraction, PCR amplification and sequencing
Foot muscle tissue from specimens were individually homogenised in 500 µl of extraction buffer (0.01M EDTA, 0.05M NaCl, 0.5M Tris-HCl pH 8.0, 2% SDS). 10 µl of 10 mg/ml proteinase K (Boehringer Mannheim) was added and the homogenate incubated at 65oC for 1 hr. Samples were extracted twice with phenol/chloroform/iso-amyl alcohol (25:24:1), followed by an extraction with chloroform/iso-amyl alcohol. The DNA was precipitated in 50 µl of TE buffer following RNAse treatment (Sambrook et al. Citation1989). Alternatively, some samples were extracted using the Aqua pure genomic DNA tissue kit (BIO RAD, USA). A 655 bp region of the COI gene was amplified by Polymerase Chain Reaction (PCR) using primers LCO-1490 (5’ GGTCAACAAATCATAAAGATATTGG 3’) and HCO-2198 (5’ TAAACTTCAGGGTGACCAAAAAATCA 3’) designed by Folmer et al. (Citation1994). Amplifications were carried out in 50 µl volume and consisted of 10 pmol of each primer, 10 mM Tris-Cl pH 8.3, 1.5 mM MgCl, 50 mM KCl and 0.2 mM of each dNTP. The addition of 2 units of Taq polymerase (Boehringer Mannheim) followed an initial 2 min denaturation at 94oC step. The remaining cycling conditions consisted of denaturation at 94oC for 1 min, annealing at 50oC for 1 min, and extension at 72oC for 1 min 30 sec for 35 cycles. A final cycle included a 5 min extension at 72oC. The resulting PCR products were purified using the QiagenTM PCR direct purification kit, following the procedure outlined by the manufacturer. Purified PCR products were sequenced using Big Dye TM Terminator Cycle Sequencing Ready reaction Mix v3.1 kit (Applied Biosystems, USA). Cycle sequencing products were analysed on ABI 3100 Avant genetic analyzer (Applied Biosystems, USA).
Analysis of sequence data
For the sequencing analyses samples from the New Caledonian species P. fibratus and the Lord Howe species P. bivarocosus were used to root the resulting phylogenetic trees with a basal polytomy because Ponder et al. (Citation2003) showed that the New Zealand species do not form a monophyletic group. Sequence data were analysed using the software SequencherTM (Gene Codes Corporation Ann Arbor, M1, USA) and then aligned by eye because there were no insertions or deletions present. Summary sequence statistics were calculated using Paup*4.0b10 (Swofford 2002) and DNASp4.0 (Rozas et al. Citation2003). Maximum likelihood parameters were selected using DT-ModSel (Minin et al. Citation2003) in conjunction with Paup*4.0. These parameters were used to estimate the maximum likelihood tree with a heuristic search (with TBR branch swapping, initial tree found by stepwise addition and 10 random addition sequence replicates). Bootstrap estimates for the maximum likelihood tree were calculated from 100 replicates with neighbour joining start trees and a limit of 150,000 TBR rearrangements per replicate. We estimated maximum parsimony networks using TCS1.21 (Clement et al. Citation2000).
We used coalescent models as implemented in Beast v1.4.7 (Drummond & Rambaut Citation2007) to reconstruct the demographic history of Placostylus species. We used an exponential growth rate model under a molecular clock with the best fit model to estimate population size changes with P. ambagiosus. The other species of Placostylus were not analysed in this way because they lacked suitable sample size and had a very low number of varied sites. The prior distributions used were: transition/transversion parameter (exponential, mean=1), proportion of invariable sites (uniform, 0–1) exponential growth rate parameter (uniform, −1×105 – 1×105) and tree height (exponential, mean=0.1). Preliminary MCMC runs were made using Beast v1.4.7 to determine appropriate chain length and operator values. A total of 5 final runs of 10 million cycles were performed and combined for calculation of parameter estimates.
We also analysed the allozyme data from Triggs & Sherley (1993) using the Mkv model Lewis (Citation2001) in MrBayes 3.1.1 (Ronquist & Huelsenbeck Citation2003). Each locus was coded as a character with the complement of alleles as multistates. We used an exponential prior (10) on the branch lengths and a flat prior on topology. The Bayesian inference was run for 10 million cycles with a 10% burnin. The run was repeated 5 times to ensure convergence.
Results
Shell size and shape
Shell heights of snails from different locations often differed for all three New Zealand species of Placostylus, although P. bollonsi shells were, in general, larger than the other two species (). However, when moisture and vegetation type at the locations were taken into account then shells of P. ambagiosus and P. hongi snails were of similar height (p=0.089) whereas those of P. bollonsi were significantly larger (p<0.001). For all species, vegetation type and to a lesser extent moisture level significantly influenced shell height (, , ). Snails living in scrub and forest were always larger than those living in shrubland habitat. Within scrub and forest, P. bollonsi were clearly larger than P. ambagiosus and P. hongii. The latter two species had larger shell heights when living in damp habitats than in dry and normal moisture habitats. The only difference in shell height between P. ambagiosus and P. hongii occurred when they lived in shrubland under normal moisture levels when P. hongii tended to be smaller than P. ambagiosus (, , ).
Figure 3 Relationship between shell length and location for New Zealand species of Placostylus land snails. Taxa are ordered firstly by species and then by geographic proximity. Habitat types are given in .
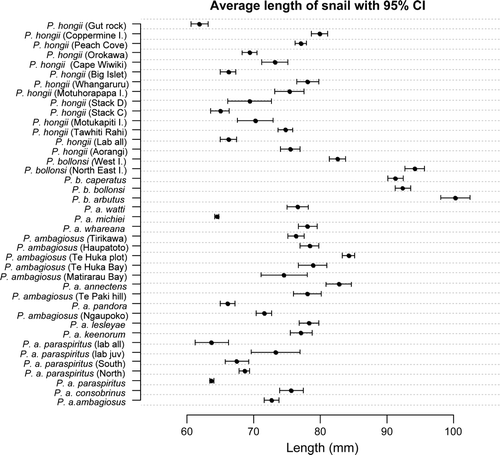
Figure 4 Mean shell height in relation to species, habitat factors of dampness level and vegetation type using a classification tree analysis. Mean shell heights in mm.
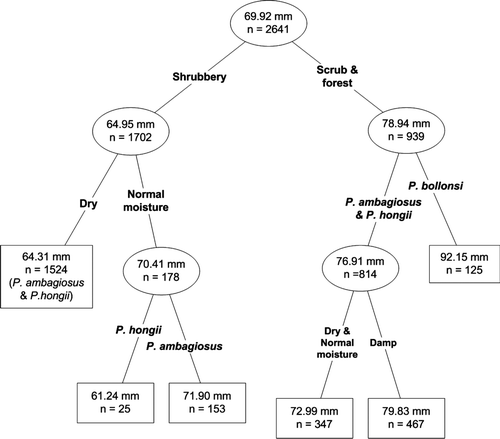
Table 2 Mean shell height (mm) in relation to species, habitat factors of moisture level and vegetation type. Vegetation types and moisture levels are provided in
Table 3 Relationship between shell height and the habitat variables of moisture level and vegetation type as shown by modelling using a mixed effects model
The shells of entirely laboratory-reared snails of P. ambagiosus were of similar height to those in the parent colony near Cape Maria van Diemen (: P. a. paraspiritus vs, P. a. paraspiritus, lab all) whereas those reared from juveniles that had been collected from the wild were significantly larger than those in the parent colony (: P. a. paraspiritus vs, P. a. paraspiritus, lab juv). In contrast, P. hongi snails that were entirely laboratory-reared were significantly smaller than those in the parent colony on Aorangi Island, Poor Knights Islands (: P. hongii from Aorangi vs. P. hongii lab all). In addition, the shells of P. a. paraspiritus snails that had been translocated to moister habitat were larger than those from the parent colony (: P. a. paraspiritus vs. P. a. paraspiritus North and South).
Shell-shapes of the three species showed wide variation although all three species differed significantly from each other (MRPP test: P. ambagiosus vs. P. hongii p<0.009; P. ambagiosus or P. hongii vs. P. bollonsi p<1×10−8). The shells of P. bollonsi snails differed the most from the other two species whereas there were only slight differences between the shells of P. ambagiosus and P. hongii: their centroids were close together (A) and most of their shell-shapes overlapped. When the untransformed data were analysed with PCA, the first three axes accounted for 93% of the variation and gave similar results to MRPP (A). However, shell-shape also tended to vary in relation to vegetation type (, ; ) and when shells of P. ambagiosus and P. hongii from the same habitat type were compared those found in forest on the mainland were not significantly different ().
Figure 5 Relationship between the shapes of P. ambagiosus, P. bollonsi and P. hongii shells from the field as shown; A, Non-metric multidimensional scaling and; B, Principal component analysis. Data were 9 different measurements from each shell. Percentage figures on axes give proportion of variation accounted for by the analysis.
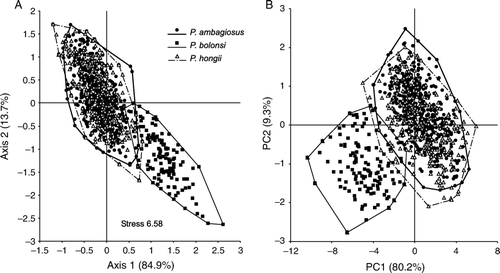
Figure 6 Variation in shape of P. ambagiosus, P. bollonsi and P. hongii shells in relation to the vegetation where they live as shown with non-metric multidimensional scaling using 9 measurements taken from each shell. Outer limits of the distributions (convex polygons as shown in ) are shown and centroids are included in A.
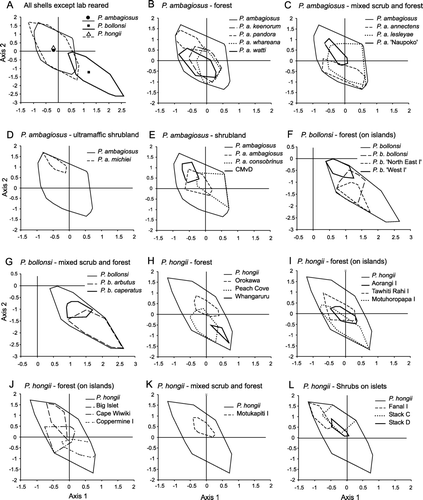
Figure 7 Variation in shell-shape of P. ambagiosus, P. bollonsi and P. hongii shells in relation to the vegetation where they live as shown principal component analysis using 9 measurements taken from each shell. Outer limits of the distributions (convex polygons as shown in ) are shown and centroids are included in A.
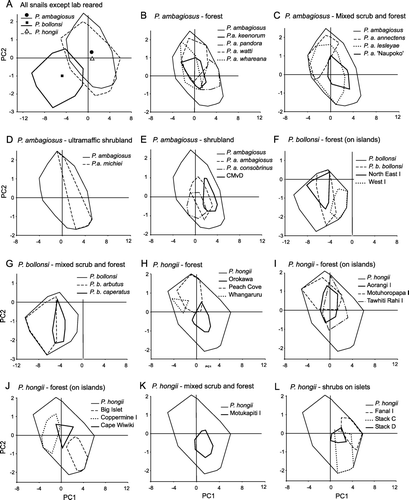
Table 4 Comparisons between the shell-shapes of snails from different habitat types
Table 5 Comparisons between the shell-shapes of P. ambagiosus and P. hongii from the same habitats
The shapes of shells from snails that were reared in the laboratory and translocated to another location differed from those at the source population and those that were reared in the laboratory also differed from those that had been translocated (; ).
Figure 8 Effect on the shell-shape of Placostylus ambagiosus and P. hongi of laboratory rearing and transfer to a different habitat type as shown with non-metric multidimensional scaling. Convex bounding polygons and centroids are shown for both reared and translocated snails and their parent populations. Outer convex polygons show variation for the species as given in .
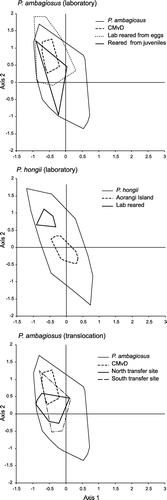
Table 6 Comparisons between the shell-shapes of laboratory reared snails and snails from populations that had originated by translocation with snails from the source populations
Phylogenetic and population genetic analysis of mtDNA sequence data and allozymes
All sequences have been submitted to GenBank with accession numbers from HQ011425 to HQ011500. Full details are given in Appendix 1. The length of the alignment was 660 sites with no insertions of deletions, after ends were removed. There were 26, 7 and 142 varied sites at the 1st, 2nd and 3rd codon positions respectively. The total number of parsimony informative sites was 132. The best-fit model in the Decision Theory method was the HKY85+I model. The maximum likelihood parameter values were base frequencies (A=0.43, C=0.15, G=0.12, T=0.30), transition/transversion ratio=3.26, and proportion of invariable sites, p inv=0.680. The base frequencies are consistent with nucleotide composition from other molluscs over this region (Holland & Hadfield Citation2002).
In agreement with the results of Triggs & Sherley (1993) and Ponder et al. (Citation2003), the phylogenies showed a clear distinction between all three Placostylus species, which were monophyletic for both the COI data (, ) and the allozyme data (). Bootstrap values from the COI data and posterior probabilities from the allozyme data were 76% or greater. The maximum parsimony haplotypes networks () for each of the three species showed no reticulations indicating very low levels of homoplasy within species. Many bootstrap values for intraspecific nodes were rather low, consistent with a low number of varied sites.
Figure 9 Maximum likelihood tree estimated under the HKY+I model for the COI sequences. Branch lengths are drawn proportional to the expected number of substitutions per site (see scale bar). Numbers above and adjacent to nodes are bootstrap proportions. Each individual is labelled with taxon, followed by population only in the case where the population is of uncertain identification, then by sample code and by haplotypes in bold.
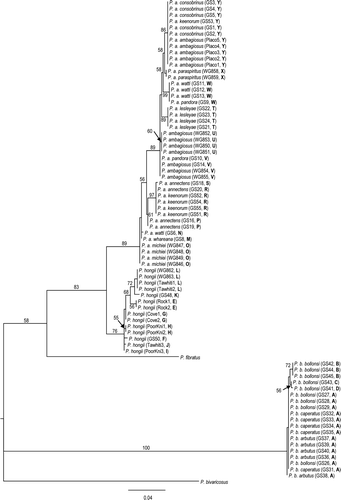
Figure 10 Maximum parsimony networks for the COI data. Large circle represent sampled haplotypes (A–Y). Short bars represent the number of mutational steps between haplotypes. Dotted arrows show the position of the root as inferred by the maximum likelihood phylogenetic analysis in .
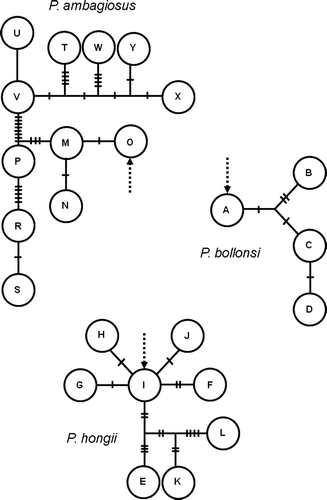
Figure 11 Bayesian tree estimated under the Mkv model for the allozyme data. Branch lengths are drawn proportional to the expected number of changes per character and numbers above nodes are estimated posterior probabilities.
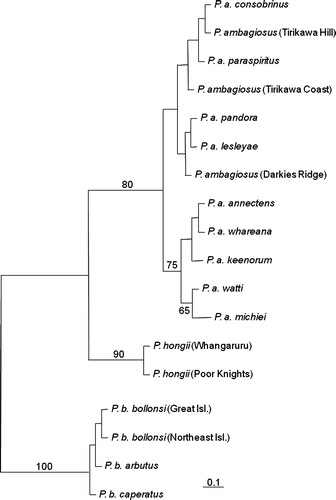
The P. ambagiosus sample was the largest and had 12 haplotypes (M–Y), the greatest number of haplotypes for all the species. Uncorrected distances within P. ambagiosus ranged from 0% to 3.12%. The root of the P. ambagiosus maximum likelihood tree was at haplotypes O, which is possessed by P. a. michiei. Within P. ambagiosus there was considerable variation that did not correspond completely with the taxonomic boundaries. The only subspecies or populations that were monophyletic and had unique haplotypes were P. a. paraspiritus, P. ambagiosus (Tirikawa Hill population), P. a. lesleyae, and P. a. michiei. The ML tree also showed an interesting phylogeographic pattern, with the haplotypes closer to the root of the P. ambagiosus sub-tree found to the east of the North Cape region, and the more derived haplotypes found progressively westwards (). There are some breaks in this pattern though, with the far eastern P. a. watti subspecies having two haplotypes (W and N) that are more commonly found towards the west.
The P. hongii dataset had 8 haplotypes (E–L) and uncorrected distances ranged from 0% to 1.62%. The P. bollonsi dataset had 4 haplotypes (A–D), which was the lowest number among all the species. The root of the P. bollonsi tree lay on haplotype A, which was the most common haplotype sampled and was possessed by all individuals sampled from Manawatawhi/Great Island. Placostylus bollonsi has three subspecies on Manawatawhi/Great Island and all sampled individuals from these subspecies had the A haplotypes. The individuals of P. b. bollonsi North East Island had a unique set of haplotypes, albeit very similar to the Manawatawhi/Great Island haplotypes. Uncorrected distances within P. bollonsi ranged from 0% to 0.63%. The root of the P. hongii tree lay on haplotype I, from the Poor Knights Islands.
The allozyme tree estimated using Bayesian inference under the Mkv model () was very similar to the distance trees from Triggs & Sherley (1993). The tree shows deep and well supported subdivisions between all three New Zealand species. Within P. ambagiosus, two major clades are evident, although only one of these receives posterior probability support over 50%.
We observed a weak correlation between mtDNA diversity and allozyme heterozygosity. Among the populations and subspecies of P. bollonsi, the North East Island had the highest allozyme polymorphism and the greatest number of haplotypes (). As for P. ambagiosus the subspecies with the greatest number of haplotypes were P. a. annectens with four haplotypes and P. a. watti with two haplotypes. However, contrary to this pattern the P. ambagiosus population from Tirikawa Coast had only one haplotype, yet 7.1% polymorphic loci, the same as P. a. watti and P. a. annectens (). P. hongii had 8 haplotypes and 21.4% polymorphic loci, both relatively high values.
Table 7 Distribution of haplotypes among taxa of New Zealand Placostylus
The results from the coalescent analysis for P. ambagiosus under the exponential growth rate model show the marginal distribution growth rate parameter includes zero (mean=–12.755, 0.95 posterior intervals=−249.178 – 216.256). Therefore, the data were unable to distinguish between a growing, shrinking or a constant population size through time.
Discussion
Taxonomy of New Zealand Placostylus species
Some well-accepted terrestrial land snail species contain large amounts of genetic variation (e.g. Thomaz et al. Citation1996; Ross Citation1999; Watanabe & Chiba Citation2001). This variation has been attributed to either a high rate of nucleotide substitution, genetic admixture, balancing selection or population structure (Thomaz et al. Citation1996), with the latter receiving support from more recent empirical studies (e.g. Watanabe & Chiba Citation2001). Placostylus differs from these examples in that genetic variation within each species is relatively low (less than 3.12% uncorrected distance). Furthermore, variation among populations and subspecies within all three Placostylus species is also very low. Most populations and subspecies do not have unique haplotypes and in fact many share haplotypes. The allozyme tree of Triggs & Sherley (1993) does show differentiation among the populations of P. ambagiosus snails but inspection of the raw data shows there are no diagnostic alleles for any of the named subspecies, and this is consistent with the mtDNA data.
Of relevance to assessing the validity of species and subspecies within Placostylus is evidence of gene flow between populations. Many of the subspecies and populations of P. ambagiosus share haplotypes, which is indicative either of ongoing gene flow or of very recent divergence. Both situations are inconsistent with dividing each species into subspecies or of separating populations of unknown status into subspecies. Rather, the genetic data here were more consistent with a single species in the Te Paki/North Cape area, P. ambagiosus. Our morphometric analyses also supported this conclusion by demonstrating that some of the variation in shell height and shape can be explained by the local environmental factors of vegetation type and moisture level. Environmental influences on shell-shape have also been previously suggested by Penniket (Citation1981), Brook & Laurenson (Citation1992) and Brook & McArdle (Citation1999). Thus the original subdivisions into subspecies using such characteristics are not indicative of underlying genetic differences. Indeed, the shape of land snail shells has long been suspected and even on occasion observed to vary in response to local environmental conditions (e.g. Solem Citation1959; Goodfriend Citation1986; Wilber & Owen 1964; Welter-Schultes Citation2000). Combined morphometric and either laboratory rearing or genetic studies showed in different species that shell-shape may indeed vary largely in response to environment or largely be determined genetically or that both may be important (e.g. Gould & Woodruff Citation1974; Estebenet & Matin 2003, Anderson et al. Citation2007, Madec & Bellido Citation2007). In the case of P. ambagiosus and P. hongi, which have shells of similar size and shape, the specific differentiation is supported by diagnostic allozyme alleles (Triggs & Sherley 1993) and the unique COI haplotypes even though the shells of these species are of similar size and shape. Furthermore, given the allopatric nature of these two species, they are clearly on different evolutionary trajectories. On the basis of these data we make the following taxonomic changes to New Zealand species of Placostylus:
Placostylus (Maoristylus) ambagiosus Suter, 1906
Placostylus hongii ambagiosus: Suter, 1906, p. 253.
Placostylus (Maoristylus) ambagiosus ambagiosus: Powell, 1947, p. 182
Placostylus (Maoristylus) ambagiosus annectens: Powell, 1938, p. 148, syn. nov.
Placostylus (Maoristylus) ambagiosus consobrinus: Powell, 1938, p. 149, syn. nov.
Placostylus (Maoristylus) ambagiosus hancoxi: Powell, 1951b, p. 136, syn. nov.
Placostylus (Maoristylus) ambagiosus keenorum: Powell, 1947, p. 186, syn. nov.
Placostylus (Maoristylus) ambagiosus lesleyae: Powell, 1947, p. 184, syn. nov.
Placostylus (Maoristylus) ambagiosus michiei: Powell, 1951b, p. 134, syn. nov.
Placostylus (Maoristylus) ambagiosus pandora: Powell, 1951b, p. 137, syn. nov.
Placostylus (Maoristylus) ambagiosus paraspiritus: Powell, 1951b, p. 137, syn. nov.
Placostylus (Maoristylus) ambagiosus watti: Powell, 1947, p. 187, syn. nov.
Placostylus (Maoristylus) ambagiosus whareana: Powell, 1951, p. 135, syn. nov.
Placostylus (Maoristylus) ambagiosus gardneri: Powell, 1951b, p. 135, syn. nov.
Placostylus (Maoristylus) ambagiosus hinemoa: Powell, 1947, p. 182, syn. nov.
Placostylus (Maoristylus) ambagiosus worthyi: Powell, 1947, p. 183, syn. nov.
Placostylus (Maoristylus) ambagiosus priscus: Powell, 1938, p. 149, syn. nov.
Placostylus (Maoristylus) ambagiosus spiritus: Powell, 1947, p. 185, syn. nov.
Placostylus (Basileostylus) bollonsi Suter, 1908
Placostylus bollonsi Suter, 1908, p. 341
Placostylus (Basileostylus) bollonsi: Haas, 1935, p. 189
Placostylus (Basileostylus) bollonsi bollonsi: Powell, 1948, p. 288.
Placostylus (Basileostylus) bollonsi caperatus: Powell, 1948, p. 289, syn. nov.
Placostylus (Basileostylus) bollonsi arbutus: Powell, 1948, p. 289, syn. nov.
This revised classification leaves three Placostylus species within New Zealand, P. ambagiosus, P. hongii and P. bollonsi and no subspecies. We have synonymised the P. ambagiosus subspecies known only from fossil material, even though no phylogenetic data are present. The recent Holocene age of these fossils (Powell Citation1979) suggests it is unlikely speciation or subspeciation has taken place. Furthermore, we have shown that the characters used to justify subspecies status are unreliable. We also note the names Placostylus hongii hongii and Placostylus annectens watti reported in Trewick et al. (Citation2009) are invalid. These names probably refer to Placostylus hongii and Placostylus ambagiosus watti, respectively.
Biogeography and phylogeography
The DNA sequence data are uninformative with regard to the long term population size in P. ambagiosus. This result may seem paradoxical given the recent decline in population size as suggested by the more extensive distribution as indicated by sub-fossil data. However, because scattered populations exist over the Te Paki/North Cape area it is possible the reduction in population size has yet to reduce haplotype diversity to a significant extent. Alternatively, longer sequence lengths or greater sampling within populations may provide stronger signal.
The phylogeography of the Northland region is poorly understood. Spencer et al. (Citation2006) performed phylogeographic analysis on several genera of landsnails from the rhytitid subfamily Paryphantinae that are distributed within Northland region. One of these taxa, Amborhytida duplicata, is distributed at several locations in the Te Paki/North Cape area. These authors observed an east-west split (see their B) that is almost identical to the split observed in our allozyme tree from P. ambagiosus. Although, we have no good evidence to suggest how fast the COI sequences are evolving in Placostylus, and so are unable to place reliable date estimates on the splits, the phylogeographic splits we see may be the result of island formation during the Pliocene and Pleistocene, as was suggested by Spencer et al. (Citation2006). Buckley et al. (Citation2010) presented mitochondrial DNA sequence data from 10 individuals of an undescribed species of stick insect from the genus Clitarchus Stål distributed throughout the Te Paki/North Cape area, but no clear phylogeographic pattern was discernable, in contrast to that of P. ambagiosus.
Conservation considerations
The lack of taxonomic distinction among populations within each of the three species suggests a revision of the desired conservation outcomes is in order. Although we recognise only a single species in each of the three geographic areas in which New Zealand Placostylus currently occur—Te Paki/North Cape (P. ambagiosus), eastern Northland and offshore islands (P. hongii), and the Manawatawhi/Three Kings Islands (P. bollonsi)—they are comprised mostly of small, scattered populations. We strongly recommend that multiple populations of each species are maintained in order to capture genetic diversity and promote the long-term survival of each species. Within P. ambagiosus there is a general east to west pattern of genetic differentiation shown both by the allozymes and mitochondrial DNA results. Thus we recommend that several populations across this east-west gradient are preserved so as to preserve the genetic variation within P. ambagiosus, which is largely geographic in nature.
The extant populations are largely clustered into several geographic groupings, some of which are non monophyletic. The first grouping contains the populations from Cape Maria van Diemen and Motuopao Island. The second contains populations in the Tirikawa/Pandora area. The third cluster of populations runs along the coast from the eastern end of Spirits Bay to Te Huka. The fourth cluster contains the populations from North Cape, and the fifth cluster contains the isolated population from Whareana. Active conservation management of at least one population from each of these clusters would ensure the full range of genetic variation is preserved. The sharing of mitochondrial haplotypes among populations and the lack of taxonomic distinction suggest translocations could be appropriate if required to ensure the survival of individual populations or to initiate new populations in areas of suitable or restored habitat.
The Manawatawhi/Three Kings endemic P. bollonsi is the most phylogenetically distinct of the three New Zealand species (Ponder et al. Citation2003) and therefore deserves the highest conservation attention, relative to the mainland species. This species also displays a high amount of genetic variation in both mtDNA and the nuclear genome relative to P. hongii but not as much as in P. ambagiosus. The presence of unique haplotypes on North East Island relative to Manawatawhi/Great Island suggests these populations have been separated for some time and did not arise by being transported between islands by Māori people within the last 2000 years as suggested by Powell (Citation1948, Citation1951) and Climo (Citation1973). We have no evidence of how the population on West Island originated because we were unable to sample that population. The land area of the Manawatawhi/Three Kings has changed considerably and repeatedly over the last 2.5 million years due to Pleistocene sea level shifts (Brook & Thrasher Citation1991). At the Last Glacial Maximum, and indeed during many earlier glacial advances, all the islands were connected. The changing topography of the islands will have forced this species through several bottlenecks, with accompanying changes in genetic variation. The current levels of genetic variation may well erode further due to genetic drift in isolated populations on different islands in the Manawatawhi/Three Kings archipelago and this loss of variation may reduce the potential for this species to adapt to future environmental changes. From a genetic point of view, it is therefore imperative that current population sizes are maintained to ensure the adaptability of this species is preserved. Indications are that all the original populations are increasing in number as the vegetation recovers following the removal of goats (Brook & Laurenson Citation1992).
In contrast, there is minimal genetic differentiation between the mainland and the Poor Knights Islands populations of P. hongii. This is shown both by the allozyme data of Triggs & Shirley (1993) and by the mtDNA data presented here. Although Powell (Citation1938, Citation1979) argued for translocation by Māori to the Poor Knights, Brook & McArdle (Citation1999) argued that the distribution was natural due to the presence of P. hongii on uninhabited rock stacks. Given the presence of unique haplotypes on Gut Rock, Fanal Island and the Poor Knights Islands, we favour the hypothesis of Brook & McArdle (Citation1999) but further genetic sampling is required to confirm this. An implication of this hypothesis is that translocations among islands may not be appropriate, given they represent isolated and independent populations.
Acknowledgements
This research was funded by the Foundation for Research, Science and Technology through the Sustaining and Restoring Biodiversity OBI and DOC investigation No. 2386. The authors would like to thank Robert Hoare for advice on nomenclature and Chris Edkins for Figs 1, 2 and 5–8. Gary Houliston, Cor Vink and two anonymous reviewers provided comment that improved the manuscript. Department of Conservation staff assisted with permits and sampling.
References
- Anderson , TK , Weaver , KF and Guralnick , RP . 2007 . Variation in adult shell morphology and life-history traits in the land snail Oreohelix cooperi in relation to biotic and abiotic factors . Journal of Molluscan Studies , 73 : 129 – 137 .
- Barker , GM . 2005 . The character of the New Zealand land snail fauna and communities: some evolutionary and ecological perspectives . Records of the Western Australian Museum Supplement , 68 : 53 – 102 .
- Brescia , FM , Pöllabauer , CM , Potter , MA and Robertson , AW . 2008 . A review of the ecology and conservation of Placostylus (Mollusca: Gastropoda: Bulimulidae) in New Caledonia . Molluscan Research , 28 : 111 – 122 .
- Brook , FJ . 1999 . Changes in the landsnail fauna of Lady Alice Island, northeastern New Zealand . Journal of the Royal Society of New Zealand , 29 : 135 – 157 .
- Brook FJ 2002 . Uncommon and rare landsnails in the Northland region of New Zealand, and an assessment of conservation management priorities . Department of Conservation , Whangarei, , New Zealand
- Brook FJ 2003 . Conservation status of the giant endemic landsnail Placostylus bollonsi on Three Kings Islands . DOC Science Internal Series 140 , Wellington, , New Zealand .
- Brook FJ , Thrasher GP 1991 . Cretaceous and Cenozoic geology of Northernmost New Zealand . New Zealand Geological Survey . Record 41.
- Brook , FJ and Laurenson , CM . 1992 . Ecology and morphological variation in Placostylus bollonsi (Gastropoda: Bulimulidae) at Three Kings Islands, New Zealand . Records of Auckland Institute and Museum , 29 : 135 – 166 .
- Brook , FJ and McArdle , BH . 1999 . Morphological variation, biogeography and local extinction of Placostylus hongii (Gastropoda: Bulimulidae) of northern New Zealand . Journal of the Royal Society of New Zealand , 29 : 407 – 434 .
- Brook F , Whaley P 2008 . Note on visit to South West Island, Three Kings Islands, 10 April 2008 . Unpublished report, Department of Consevation, Whangarei. 2 p.
- Buckley , TR and Bradler , S . 2010 . Tepakiphasma ngatikuri, a new genus and species of stick insect (Phasmatodea) from the Far North of New Zealand . New Zealand Entomologist , 33 : 118 – 126 .
- Buckley , TR , Marske , K and Attanayake , D . 2010 . Phylogeography and ecological niche modelling of the New Zealand stick insect Clitarchus hookeri (White) support survival in multiple coastal refugia . Journal of Biogeography , 37 : 682 – 695 .
- Chapple , DG , Patterson , GB , Bell , T and Daugherty , CH . 2008 . Taxonomic revision of the New Zealand copper skink (Cyclodina aenea: Squamata: Scincidae) species complex, with descriptions of two new species . Journal of Herpetology , 42 : 437 – 452 .
- Choat , JH and Schiel , DR . 1980 . Population structure of Placostylus hongii (Gastropoda: Paryphantidae) on the Poor Knights Islands . New Zealand Journal of Zoology , 7 : 199 – 205 .
- Clement , M , Posada , D and Crandall , KA . 2000 . TCS: a computer program to estimate gene genealogies . Molecular Ecology , 9 : 1657 – 1660 .
- Climo , FM . 1973 . The systematics, biology and zoogeography of the land snail fauna of Great Island, Three Kings Group, New Zealand . Journal of the Royal Society of New Zealand , 3 : 565 – 587 .
- de Lange , PJ , Heenan , PB and Dawson , MI . 2003 . A new species of Leucopogon (Ericaceae) from the Surville Cliffs, North Cape, New Zealand . New Zealand Journal of Botany , 41 : 13 – 21 .
- Druce , AP , Bartlett , JK and Gardner , RO . 1979 . Indigenous vascular plants of the serpentine area of Surville Cliffs and adjacent cliff tops, northwest of North Cape, New Zealand . Tane , 25 : 187 – 206 .
- Drummond , AJ and Rambaut , A . 2007 . BEAST: Bayesian evolutionary analysis by sampling trees . BMC Evolutionary Biology , 7 : 214
- Estebenet , AL and Martin , PR . 2003 . Shell interpopulation variation and its origin in Pomacea canaliculata (Gastropoda: Ampullariidae) from southern pampas, Argentina . Journal of Molluscan Studies , 60 : 301 – 310 .
- Folmer , O , Black , M , Hoeh , W , Lutz , R and Vrijenhoek , R . 1994 . DNA primers for amplification of mitochondrial cytochrome c oxidase subunit I from diverse metazoan invertebrates . Molecular Marine Biology and Biotechnology , 3 : 294 – 299 .
- Goodacre , SL and Wade , CM . 2001 . Molecular evolutionary relationships between partulid land snails of the Pacific . Proceedings of the Royal Society of London B. , 268 : 1 – 7 .
- Goodfriend , GA . 1986 . Variation in land-snail shell form and size and its causes: a review . Systematic Zoology , 35 : 204 – 223 .
- Gould , SJ and Woodruff , DS . 1974 . Genetics and morphometrics of Cerion at Pongo Carpet: a new systematic approach to this enigmatic landsnail . Systematic Zoology , 23 : 518 – 536 .
- Goulstone , JF , Mayhill , PC and Parrish , GR . 1993 . An illustrated guide to the land Mollusca of the Te Paki Ecological Region, New Zealand . Tane , 34 : 1 – 32 .
- Hayward , BW and Brook , FJ . 1981 . Exploitation and redistribution of flax snail (Placostylus) by the prehistoric Maori . New Zealand Journal of Ecology , 4 : 33 – 36 .
- Heller , J . 2001 . “ Life history strategies ” . In The biology of terrestrial molluscs , Edited by: Barker , GM . 413 – 445 . Wallingford, CABI Publishing. Pp .
- Hemp , P and Bertness , MD . 1984 . Snail shape and growth rates: evidence for plastic shell allometry in Littorina littorina . Proceedings of the National Academy of Science USA , 81 : 811 – 813 .
- Hitchmough R , Bull L , Cromarty P 2007 . New Zealand threat classification system lists 2005 . Department of Conservation , Wellington
- Holland , BS and Hadfield , MG . 2002 . Islands within an island: phylogeography and conservation genetics of the endangered Hawaiian tree snail Achatinella mustelina . Molecular Ecology , 11 : 365 – 375 .
- Larochelle A , Larivière MC 2005 . Harpalini (Insecta: Coleoptera: Carabidae: Harpalinae) . Fauna of New Zealand , vol. 53 . Manaaki Whenua Press , Lincoln
- Lewis , PO . 2001 . A likelihood approach to estimating phylogeny from discrete morphological character data . Systematic Biology , 50 : 913 – 925 .
- Madec , L and Bellido , A . 2007 . Spatial variation of shell morphometrics in the subantarctic land snail Notodiscus hookeri from Crozet and Kerguelen Islands . Polar Biology , 30 : 1571 – 1578 .
- McCune B , Grace JB 2002 . Analysis of ecological communities . Gleneden Beach, OR, MjM Software Design.
- Marshall , BA and Barker , GM . 2007 . A revision of New Zealand landsnails of the genus Cytora Kobelt & Möllendorf, 1897 (Mollusca: Gastropoda: Pupinidae) . Tuhinga , 18 : 49 – 113 .
- Minin , V , Abdo , Z , Joyce , P and Sullivan , J . 2003 . Performance-based selection of likelihood models for phylogeny estimation . Systematic Biology , 52 : 674 – 683 .
- Ogle CC 1979 . Critical status of Placostylus and Paryphanta land snails in the Far North. Faunal Survey Unit Report No. 14 New Zealand Wildlife Service , Wellington
- Parrish R , Sherley G , Aviss M 1995 , Giant land snail recovery plan Placostylus spp., Paryphanta sp. Threatened Species Recovery Plan Series No. 13 . Department of Conservation , Wellington
- Penniket ASW 1981 . Population studies of land snails of the genus Placostylus in the North of New Zealand . Unpublished MSc thesis, University of Auckland, Auckland.
- Ponder , WF , Colgan , DJ , Gleeson , DM and Sherley , GH . 2003 . Relationships of Placostylus from Lord Howe Island: an investigation using the mitochondrial cytochrome c oxidase 1 gene . Molluscan Research , 23 : 159 – 178 .
- Powell , AWB . 1935 . Land Mollusca of the Three Kings Islands, New Zealand . Proceedings of the Malacological Society of London , 21 : 243 – 248 .
- Powell , AWB . 1938 . The Paryphantidae of New Zealand no. IV. and the genus Placostylus in New Zealand . Records of the Auckland Institute and Museum , 2 : 133 – 150 .
- Powell , AWB . 1947 . Distribution of Placostylus land snails in northernmost New Zealand . Records of the Auckland Institute and Museum , 3 : 173 – 189 .
- Powell , AWB . 1948 . Land mollusca of the Three Kings . Records of the Auckland Institute and Museum , 3 : 273 – 290 .
- Powell , AWB . 1951 . On further colonies of Plascostylus land snails from northernmost New Zealand . Records of the Auckland Institute and Museum , 4 : 134 – 140 .
- Powell AWB 1979 . New Zealand Mollusca: marine land and freshwater shells . Collins , Auckland
- Ramsay , GW and Gardner , NW . 1977 . Endangered and rare New Zealand invertebrate species . Weta , 1 : 3 – 6 .
- Ronquist , F and Huelsenbeck , JP . 2003 . MrBayes 3: Bayesian phylogenetic inference under mixed models . Bioinformatics , 19 : 1572 – 1574 .
- Ross , TK . 1999 . Phylogeography and conservation genetics of the Iowa Pleistocene snail . Molecular Ecology , 8 : 1363 – 1373 .
- Rozas , J , Sanchez-DelBarrio , JC , Messenguer , X and Rozas , R . 2003 . DNAsp: DNA polymorphism analyses by the coalescent and other methods . Bioinformatics , 19 : 2496 – 2497 .
- Sambrook J , Fritsch EF , Maniatis T 1989 . Molecular cloning: a laboratory manual , 2nd edition Cold Spring Harbor Laboratory Press , New York
- Sherley GH 1990 . Transferal of Placostylus ambagiosus paraspiritus from Cape Maria van Deimen 16 and 17 May 1999 with comments on the condition of the colony . Unpublished report. Department of Conservation, Wellington.
- Sherley , G . 1996 . Morphological variation in the shells of Placostylus species (Gastropoda: Bulimulidae) in New Zealand and implications for their conservation . New Zealand Journal of Zoology , 23 : 73 – 82 .
- Solem , A . 1959 . Systematics and Zoogeography of the land and fresh-water Mollusca of the New Hebrides . Fieldiana: Zoology , 43 : 1 – 359 .
- Spencer , HG , Brook , FJ and Kennedy , M . 2006 . Phylogeography of kauri snails and their allies from Northland, New Zealand (Mollusca: Gastropoda: Rhytididae: Paryphantinae) . Molecular Phylogenetics and Evolution , 38 : 835 – 842 .
- Stringer IAN , Grant EA 2007 . Captive rearing and biology of the endangered giant land snails Placostylus ambagiosus and P. hongii (Pulmonata: Bulimulidae) DOC Research & Development Series 279. Department of Conservation , Wellington
- Stringer , IAN , Parrish , GR and Sherley , GH . 2004 . Population structure, growth and longevity of Placostylus hongii (Pulmonata: Bulimulidae) on Tawhiti Rahi Island, Poor Knights Islands, New Zealand . Pacific Conservation Biology , 9 : 241 – 247 .
- Suter , H . 1908 . A new Placostylus from New Zealand . Transactions and Proceedings of the New Zealand Institute , 40 : 34 – 343 .
- Swofford DL 2002 PAUP*. Phylogenetic analysis using parsimony (* and other methods) . Version 4 . Sunderland , MA, Sinauer Associates .
- Thomaz , D , Guiller , A and Clarke , B . 1996 . Extreme divergence of mitochondrial DNA within species of pulmonate land snails . Proceedings of the Royal Society of London B , 263 : 363 – 368 .
- Townsend , JH , Slapcinsky , J , Krysko , KL , Donlan , EM and Golden , EA . 2005 . Predation of a tree snail Drymaeus multilineatus (Gastropoda: Bulimulidae) by Iguana iguana (Reptilia: Iguanidae) on Key Biscayne, Florida . Southeastern Naturalist , 4 : 361 – 364 .
- Trewick S , Brescia F , Jordan C 2009 . Diversity and phylogeny of New Caledonian Placostylus land snails; evidence from mitochondrial DNA . In : Grandcolas P Zoologia Neocaledonica 7. Biodiversity studies in New Caledonia. Mémoires du Muséum national d'Histoire naturelle , 198 . Pp 421 – 436 .
- Triggs , SJ and Sherley , GH . 1993 . Allozyme genetic diversity in Placostylus land snails and implications for conservation . New Zealand Journal of Zoology , 20 : 19 – 33 .
- Watanabe , Y and Chiba , S . 2001 . High within-population mitochondrial DNA variation due to microvicariance, population mixing in the land snail Euhadra quasita (Pulmonata: Bradybaenidae) . Molecular Evolution , 10 : 2635 – 2645 .
- Welter-Schultes , FW . 2000 . The pattern of geographical and altitudinal variation in the land snail Albinaria idaea from Crete (Gastropoda: Clausiliidae) . Biological Journal of the Linnean Society , 71 : 237 – 250 .
- Wilbur , KM and Owen , G . 1964 . “ Growth ” . In Physiology of Mollusca , Edited by: Wilbur , KM and Yonge , CM . Vol. 1 , 211 – 242 . New York : Academic Press. .
- Winterbourn , MJ . 2009 . A new genus and species of Leptophlebiidae (Ephemeroptera) from northern New Zealand . New Zealand Journal of Zoology , 36 : 423 – 430 .