Abstract
Knowing when and where animals are active improves wildlife management. We monitored variation in long-tailed bat (Chalinolobus tuberculatus) echolocation activity at two sites (one urban, one rural) in Hamilton, New Zealand, across different nights, seasons, microhabitats and environmental conditions. Activity peaked between the first and third hours after sunset. Activity was higher during spring and summer compared with winter. At the rural site, more passes were recorded at 4–6 m than at 15–30 m. More bat passes were recorded in microhabitats with water. Temperature correlated positively with activity at the rural site only. To maximize detections we recommend that bats be monitored: (1) during warmer months (spring/summer), (2) on warm nights (≥ 7°C), 3) at lower heights (≤ 6 m from the ground), and (4) in open edge and forested microhabitats with water. Recommendations should enable practitioners to focus resources so that bats are effectively detected in urban and rural habitats.
Introduction
Knowing when and where animals are active can guide decision-making and improve wildlife management by practitioners. Bats are difficult to monitor and manage due to their nocturnal and mobile nature (Fenton Citation2003). The behaviour, distribution and conservation needs of many species remain poorly understood (Mickleburgh et al. Citation2002).
Management of bat populations in human-dominated environments is disadvantaged by a lack of knowledge about how bats use these ecosystems (O’ Shea et al. Citation2003). In some urban environments species thrive. For example, big brown bats (Eptesicus fuscus) gain thermal benefits from roosting in buildings (Lausen & Barclay Citation2006). Conversely, many species are disadvantaged by direct (e.g. road mortality; Lesinski Citation2008) and indirect (e.g. reduced roosting resources; Sparks et al. Citation2005) human impacts. Some species depend heavily on urban green spaces (e.g. parklands; Gaisler et al. Citation1998). These areas may have attributes that are favourable for roosting and foraging (e.g. supporting mature trees; Hein et al. Citation2009). However, even within green-space habitats, bat diversity and activity can be lower compared with natural habitats (e.g. Avila-Flores & Fenton Citation2005) due to: reduced resource availability (e.g. roost sites and prey; Bartonička & Zukal Citation2003), social factors (e.g. differential habitat use by sexes; Safi et al. Citation2007), and anthropogenic variables (e.g. effects of artificial light; Scanlon & Petit Citation2008). To better understand how bats use modified habitats, monitoring programmes need to account for activity at multiple temporal and spatial scales (Bat Conservation Trust Citation2007). In turn, knowing when and where bats are most active should allow managers to maximize detection and capture rates, thereby focusing limited resources and improving project outcomes.
New Zealand has two extant bat species—long-tailed bats (Chalinolobus tuberculatus) and lesser short-tailed bats (Mystacina tuberculata), which form the entirety of New Zealand's native terrestrial mammal fauna. Developing field-based monitoring methods for each bat species is a high priority (Molloy Citation1995). New Zealand bats are monitored using one of two methods: (1) trapping followed by radio-tracking, and (2) monitoring echolocation activity using detectors. Trapping and radio-tracking enable identification of home ranges, roost sites and relative abundance—information not directly obtainable from detectors (MacSwiney et al. Citation2008). Detectors allow bats to be non-invasively monitored over time and across sites by transforming ultrasonic echolocation pulses (sounds > 20 kHz) into sounds audible to humans (O'Farrell & Gannon Citation1999).
Long-tailed bats are small (8–14 g) aerial insectivores that use echolocation (c.40 kHz pulses) for orientation and foraging (Parsons et al. Citation1997). They are listed as nationally vulnerable (threatened in the North Island) with population declines attributed to habitat loss and predation by pests (O'Donnell et al. Citation2010). Populations are small (tens to hundreds of individuals), fragmented, and widely dispersed, occurring in indigenous forest (O'Donnell Citation2000), plantations (Borkin & Parsons Citation2009) and agricultural landscapes (Griffiths Citation2007). However, Hamilton (North Island, New Zealand) is one of the only confirmed cities in New Zealand to support a population of bats (Le Roux & Waas Citation2012; Dekrout Citation2009), despite the Hamilton Ecological District being one of the most ecologically degraded regions in New Zealand (Clarkson & McQueen Citation2004). The use of urban landscapes by New Zealand bats is not well understood. Better understanding of bat activity in urban habitats should guide monitoring efforts in New Zealand cities. We used detectors to monitor bat activity at two exotic forest habitats, one on the urban edge and one on the rural outskirts of Hamilton. We aimed to develop practical monitoring recommendations to determine when and where to deploy detectors to maximize long-tailed bat detection. This information should optimize detection of long-tailed bats and capture rates by allowing practitioners to effectively focus resources and monitoring effort during times and at locations of peak activity.
Materials and methods
Site descriptions
Bat activity was monitored over 9 months (July 2009 to March 2010) at two sites, one on the urban edge and one on the rural outskirts of Hamilton (37°47'S, 175°17'E). Sites were: small exotic forest fragments separated by 4 km, flanked by the Waikato River—a major landscape feature, and situated within the same ecotone–the Mangakotukutuku and Mangaonua gully systems. The first site was a 1.2-ha mature oak (Quercus robur) fragment on the outskirts of the city (i.e. the ‘rural site’), supporting approximately 200 mature oak trees (> 100 years old; ring counts of felled trees) with a mean diameter at breast height of 60.1 ± 3.22 cm (10 × 10 m vegetation plots). Trees had canopy heights of ≥ 25 m. Agricultural land flanked the site to the south, east and west and the Waikato River to the north. The second site was 0.3 ha of open parkland situated adjacent to a 1-ha native broadleaf forest remnant (Hammond Bush) and medium-density residential housing on the edge of the city (i.e. the ‘urban site’). Along the edge of the park, mature exotic trees including, alder (Alnus sp.), willow (Salix cinerea), acacia (Acacia sp.), eucalypts (Eucalyptus spp.) and pine (Pinus radiata) were dominant. Monitoring was restricted to these two sites because at the time of this study they were the only sites known to support bat roosts (Dekrout Citation2009). Moreover, both sites are situated in close proximity with similar landscape features (i.e. mature exotic trees and riparian margins), facilitating comparison.
Monitoring design and equipment
The rural site was divided into four proportionally representative microhabitats of equal size (0.3 ha) based on the presence/absence of water bodies: (1) an open edge with no water bodies, (2) an open edge with an irrigation channel and pond, (3) a sheltered interior dominated by a clearing with a stream, and (4) a river edge. In each microhabitat, four trees were selected (n = 16) to establish rope and pulley systems used to hoist detectors to two height tiers per tree: 4–6 m and 25–30 m. Trees were selected based on: safety for climbing purposes, height of the tree (> 25 m to increase independent vertical monitoring), and the distance between trees in each microhabitat (> 25 m to increase independent horizontal monitoring). The vertical arrangement of detectors enabled concurrent monitoring of bat activity within the air space between the ground and mid-storey (between 0 and 20 m) and between the mid-storey and above the canopy (between 21 and 40 m). The sensitivity of detectors was at least 20 m (tested using a ‘chirp board II’ simulating 40 kHz echolocation pulses). Detectors were orientated upward (approximately 45 degrees from the horizon) following attachment to rope and pulley systems. At the urban site, this set-up was replicated in four alder trees. It was not possible to select taller trees at this site because of safety concerns for climbers, resulting in the higher tier being lower at 15–20 m. Microhabitats were not delineated at this site because the area was too small.
Ten automated heterodyne detectors were used to monitor bats (The Department of Conservation; Lloyd Citation2009). Detectors had similar sensitivities and were assumed to have an equal chance of detecting echolocation pulses. Detectors were calibrated to the same time and date settings (New Zealand Standard Time) and pre-set to start monitoring 30 minutes before sunset and continue until 30 minutes after sunrise. The five vertically arranged detector pairs were used to concurrently monitor bat activity at one tree at the urban site and four trees at the rural site (i.e. within each of the microhabitats). Detector pairs, kept the same throughout the study, were assigned to different trees (4 × 4 Latin square), and microhabitats/urban site (5 × 5 Latin square) every 3–5 days. Detectors of each pair were alternated between lower and higher tiers on each deployment. Detectors were not placed at < 4 m because of theft risks. Bat activity was monitored on as many nights as possible, irrespective of weather conditions over four seasons: winter (July and August), spring (September to November), summer (December to February) and autumn (March). Recorded passes were stored onto Secure Digital cards (2 GB, SanDisk) for inspection.
Data collection and classification
Recorded echolocation files were sorted by visual and auditory inspection of waveforms using Bat Search 1.02 Software® (The Department of Conservation, 2008, New Zealand; Lloyd Citation2009). Sound files were sorted into: (1) echolocation passes, defined as files containing two or more 40-kHz pulses emitted in sequence (Parsons et al. Citation1997), and (2) non-bat sounds (wind, rain or insect-generated noise) that were discarded. Echolocation passes were classified into two bat call categories: (1) search phase pulses with low pulse repetition rates used for commuting and locating prey (mean inter-pulse interval of c.104 ms), or (2) feeding buzzes consisting of a series of rapid pulses used to determine the range of prey before capture (mean inter-pulse durations of c.4.5 ms; Parsons et al. Citation1997). Files containing one or more feeding buzzes were classified as a single feeding event. Echolocation pulses were recorded with a date (day/month/year) and time (hour/minute/second) stamp. Time stamps of echolocation passes recorded at lower and higher heights were inspected to ensure that they did not overlap in time. On rare occasions when this occurred (< 100), the pulse with the greatest intensity was classified to avoid double counts of what was assumed to be a single bat flying past. Data for a range of environmental variables logged at hourly intervals were retrieved from the national weather station located at Hamilton International Airport, 2.5 and 6.5 km from the rural and urban sites, respectively (agent number 2112, network number C75834, MetService, National Institute for Water and Atmosphere, Ltd).
Statistical analyses
Data were kept separate for the rural and urban sites to identify site-specific trends. Our dependent variable was bat passes/detector/night, referred to here as either ‘bat passes/night’ or ‘activity’ for simplicity. To determine the effect of season on bat activity at each site we constructed Generalized Linear Mixed Models. We fitted ‘season’ as a fixed effect and ‘survey night’ (date), ‘detector pair’ and ‘sample tree’ as random effects. For the rural site, we also incorporated ‘microhabitat’ as a fixed effect. Bat passes/night were marginally over-dispersed and fitted in Generalized Linear Mixed Models using negative binomial distributions with log-link functions and aggregation parameters held at one. To determine what effect detector height had on recorded bat activity, we used non-parametric Wilcoxon Matched-Pairs tests, which assess differences between two related samples. Due to equipment failure, not every detector in each pair recorded bat activity every night, resulting in smaller sample sizes for these analyses. Generalized log-linear regression models were used in a stepwise manner to determine which continuous environmental variables or combination of variables served as ‘predictor/s’ of bat activity at each site. Models assumed a Poisson distribution and used a Newton–Raphson algorithm. Environmental variables of interest included mean nightly: (1) temperature (°C), (2) relative humidity (%), (3) precipitation (mm), (4) wind speed (m/s), (5) relative cloud amount (octa), and (6) percentage of full moon (phase). Analyses were undertaken in GenStat (14th edition; VSN International Ltd, Hemel Hempstead, UK). Means are presented with ± one standard error of means (SEM).
Results
Both sites were monitored for 2300 h (217 nights) over 9 months. Bats were detected every month at both sites and on approximately 84% of monitoring nights at the rural (183/217 nights) and urban (180/217) sites. At each site, bats were recorded during approximately 5800 min or just 4% of the total recording time. A total of 22,833 echolocation passes were recorded and classified (11,077 at the urban site from one detector pair and 11,756 at the rural site from four detector pairs). A total of 1472 (6.4%) passes were classified as feeding buzzes.
Nightly and seasonal activity
Nightly activity was highly variable, characterized by distinctive peaks and falls across continuous recording nights (). Activity peaked between the first and third hours after sunset at both sites across all seasons (). Bimodal activity patterns with an additional peak in activity 3–4 h before sunrise were observed during spring, summer and autumn. This was most evident at the rural site. Feeding buzzes were rarely recorded. However, during winter at the rural site a peak in feeding activity was evident in the early evening.
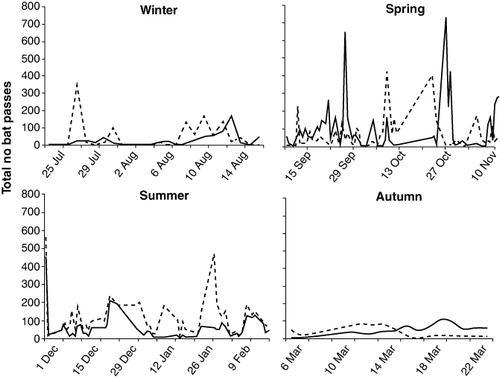
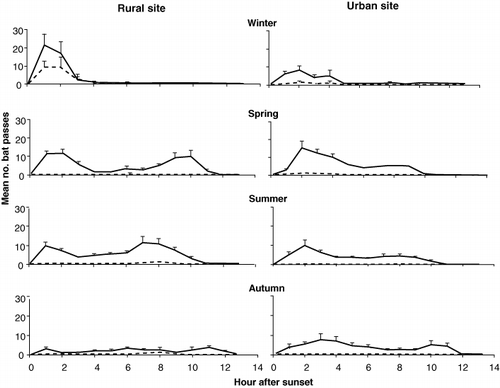
The effect of season on bat activity was significant at the rural site (Wald = 22.83, d.f. = 3, P < 0.001) and urban site (Wald = 25.34, d.f. = 3, P < 0.001). Activity was significantly higher (P < 0.001) in spring and summer compared with winter at both sites (). At the urban site, there was no significant difference between bat activity recorded in autumn and spring (P = 0.406) or summer (P = 0.068). However, at the rural site there was significantly less (P < 0.001) activity in autumn compared with spring and summer. There was no random effect of detector pair (P = 0.346) or sample tree (P = 0.231), but survey night was significant (P < 0.001). Activity peaked in September at the rural site (mean passes/night of 12.28 ± 1.65) and November at the urban site (mean passes/night of 31.27 ± 5.81). The longest consecutive monitoring period without bat detections at each site was three nights during winter.
Table 1 Mean number (± SEM) of bat passes/detector/night for each season, detector height (all seasons) and microhabitat (all seasons) at the rural and urban sites.
Detector height and microhabitat
At the rural site, significantly more bat passes/night were recorded on detectors placed at lower heights compared with higher heights (Wilcoxon = 1657,n = 171 nights with four detector pairs, P < 0.001). At the urban site, no significant difference (Wilcoxon = 3676, n = 131 nights with one detector pair, P = 0.947) in activity was detected between height tiers (; ).
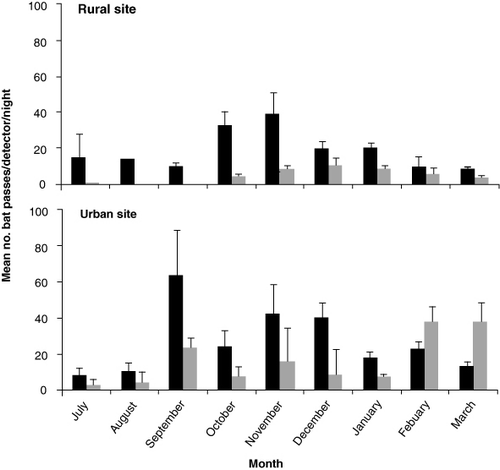
Microhabitat had a significant effect (Wald = 46.30, d.f. = 3, P < 0.001) on activity at the rural site. Significantly more (P < 0.001) bat passes/night were recorded on detectors situated in microhabitats containing water (). The number of bat passes recorded in the open edge microhabitat without a water body accounted for < 20% of passes in each season (mean of 12.24 ± 3.51% of all passes). We observed differences in the use of microhabitats by bats across seasons (). During winter, 60% of passes were recorded in the sheltered interior microhabitat. During spring, there was near equal use of all three microhabitats containing water bodies. During autumn, 60% of passes were recorded along the river edge.
Environmental predictors
At the urban site, no environmental variables were significant (P > 0.05) predictors of activity. At the rural site, mean nightly temperature was the only positive and significant predictor of activity (Wald = 407.6, d.f. = 6, P < 0.001). Activity increased from an average of 4.22 ± 1.43 passes/night at temperatures < 7 °C to 58.68 ± 6.98 passes/night at temperatures ≥ 7 °C (i.e. 93% increase in pass rates).
Discussion
Variation in long-tailed bat echolocation activity documented in the modified habitats in our study is consistent with activity patterns reported for long-tailed bats in forested ecosystems elsewhere in New Zealand (e.g. O'Donnell et al. Citation2006) and for other temperate insectivorous bat species overseas (e.g. Scanlon & Petit Citation2008). Our results emphasize the importance of accounting for both spatial and temporal changes in bat activity in future bat monitoring programmes in order to improve research and management outcomes.
Nightly and monthly activity patterns
Stereotypical peaks and falls in nightly activity over our study period () likely represent variation in foraging effort, which may be linked with changes in ephemeral prey availability and environmental conditions. Radio-tracking has shown that individuals move between habitats along the southern urban–rural interface of Hamilton using the Waikato River as a corridor (Dekrout Citation2009). Therefore, similarities in activity patterns at both sites may be due to the same individuals using these habitats.
At both sites and across all seasons activity peaked between the first and third hours after sunset (). Peaks in activity during the evening are probably explained by individuals replenishing energy levels after emergence (Kunz Citation1974). Greater availability of flying insect prey and warmer dusk temperatures may also explain evening activity peaks (e.g. Jones & Rydell Citation1994). Activity decreased during the middle of the night across all seasons. Flight can be energetically expensive and discontinuous nightly activity likely represents bursts of foraging activity interspersed with periods of roosting in which individuals rest, digest food and socialize (e.g. Speakman & Thomas Citation2003; Murray & Kurta Citation2004).
Feeding buzzes are a conclusive measure of foraging effort for insectivorous bats (Miller Citation1999). Feeding buzzes accounted for < 7% of recorded bat passes, making it difficult to identify trends in foraging sequences. The exception was during winter, when a peak in feeding buzzes at the rural site was evident. Temperate bat species, including long-tailed bats, are capable of torpor whereby individuals reduce body temperatures to conserve energy. During winter, individuals can remain inactive for several days rousing periodically to forage (Turbill Citation2008). Peaks in feeding activity during winter are likely to represent individuals foraging for brief, intense periods to maintain energy reserves. Bats were always detected by the fourth night of monitoring at both sites even during winter. We recommend a minimum of four consecutive monitoring nights to detect long-tailed bats during colder periods or at habitats with low activity.
In spring and summer, significantly more bat activity was recorded at both habitats, compared with winter. This is consistent with reports of seasonal variation in long-tailed bat activity in other parts of New Zealand (e.g. O'Donnell Citation2001), with few exceptions (e.g. Borkin & Parsons Citation2009). Increased activity during spring and summer is probably linked to increased insect availability and reduced thermoregulatory costs (Speakman & Thomas Citation2003). In spring, summer and autumn, additional peaks in foraging activity were observed before dawn. Bimodal peaks in activity have previously been observed in long-tailed bats (Griffiths Citation2007), and probably represent increased foraging effort during favourable conditions and individuals returning to roosts before sunrise. Bimodal activity patterns have also been linked with reproductive status in females, as higher energy demands associated with pregnancy and lactation require more frequent foraging (Swift Citation2009). Pronounced bimodal peaks in activity and higher pass rates during spring at the rural site may indicate nearby maternity roost(s) during this period.
Detector height and microhabitat patterns
Previous studies show that long-tailed bat activity can vary between habitat types (e.g. grasslands and forest edges; O'Donnell et al. Citation2006) but this is the first study to examine differential use of microhabitats by long-tailed bats (for an example in lesser short-tailed bats see Scrimgeour et al. Citation2013). Bat activity at both sites varied in relation to vertical airspace and horizontal microhabitats. At the rural site, significantly more passes were recorded on detectors placed at lower heights compared with greater heights. A similar, non-significant, trend was observed at the urban site (). The non-significant result at the urban site is probably the result of two factors: (1) sample trees were not as tall (< 20 m) compared with the rural site (> 25 m), which is likely to have influenced detection rates at higher tiers, and (2) the urban site is characterized by a large open space (i.e. low-clutter environment) compared with the rural site, which is densely stocked with trees (i.e. high-clutter environment). Bats may fly lower at the rural site more frequently to avoid obstacles in the canopy such as structural clutter from branches, which may limit manoeuvrability and alter echolocation signals through attenuation and pulse–echo overlaps (Kalcounis et al. Citation1999; Broders et al. Citation2004).
Seasonal variation in vertical activity was also observed. During spring, summer and autumn, more activity was recorded at greater heights compared with winter. This may be linked with increased temperatures and greater insect availability at higher elevations (Hecker & Brigham Citation1999; Collins & Jones Citation2009). Our results suggest that placing detectors at heights of 4–6 m will increase detection rates. Placing detectors at heights < 4 m from the ground may also be effective; however, we could not place detectors at these heights because of the risk of equipment being stolen in public areas. This is also an important practical consideration for urban studies.
Long-tailed bat activity was non-randomly distributed between microhabitats at the rural site. The percentage of bat passes recorded in the open edge microhabitat lacking a water body accounted for < 20% of all passes in each season (). The occurrence of water bodies in the other microhabitats probably contributed to increased activity. Insect concentrations have been shown to increase as the distance to water bodies decreases (Fukui et al. Citation2006). Insectivorous bats exploit insect concentration over natural and modified water sources (e.g. Verboom et al. Citation1999; Vindigni et al. Citation2009). During autumn, 60% of bat activity occurred along the river edge. This may be related to bats accumulating fat reserves before winter (O'Donnell Citation2005). Activity along riparian margins may also be related to individuals using this linear ‘corridor’ to move between habitats (Dekrout 2008). Given that microhabitat variation in bat activity was investigated at one site in this study, these data should be viewed as preliminary. Nevertheless, long-tailed bats are responsive to small-scale habitat features, which influence detection rates at fine spatial scales.
Environmental predictors of activity
The only predictor of long-tailed bat activity was mean nightly temperature at the rural site only. Several studies have found that changes in temperature can significantly affect bat activity (e.g. Milne et al. Citation2005). Other studies have found that bats respond to environmental variables such as wind, rain and moonlight (e.g. Rydell Citation1991). However, the effects of these variables vary between species and regions. No effect of wind, rain or moonlight on bat activity in our study is consistent with studies undertaken on bats in temperate regions (e.g. Gaisler et al. Citation1998; Scanlon & Petit Citation2008). Moreover, environmental variables may affect bat activity in indirect and complex ways (e.g. moonlight and rainfall may affect invertebrate abundance, which may influence bat activity; e.g. Hecker & Brigham Citation1999). For practical reasons (e.g. damage to equipment) practitioners may wish to avoid bat surveys on rainy/windy nights.
The site-specific influence of temperature is intriguing and lends support to speculation that male and female long-tailed bats may use different habitats along the southern urban–rural interface of Hamilton, associated with maternity roosting. Sex-specific thermal requirements may explain behavioural differences in bat activity and habitat use (e.g. Senior et al. Citation2005). Females of some bat species have exacting roost requirements because of higher thermoregulatory demands associated with reproduction, compared with fewer constraints in males (e.g. Speakman & Thomas Citation2003). In long-tailed bats, varying thermal requirements between sexes may explain differences in roost sizes, with females being more communal than males (Sedgeley Citation2001). Previous bat trapping at the urban site (summer and autumn 2004–2007) revealed a male capture bias (23 males: one female; Dekrout Citation2009). This might suggest that females prefer to use rural habitats over urban ones; however, low capture rates and the fact that no bats have been captured at the rural site despite previous attempts, makes it impossible to compare sex ratios between these two habitats. More research is needed to determine if differential use of habitats occurs in males and females and whether this is driven by maternity roost selection or other factors (e.g. anthropogenic disturbances; Le Roux & Waas Citation2012).
Monitoring recommendations
To maximize long-tailed bat detections in urban and rural habitats we recommend that bats be monitored: (1) during warmer months (spring and summer), (2) on warm nights (≥ 7 °C), (3) by placing detectors at lower heights (≤ 6 m from the ground), and (4) by placing detectors in open edge and forested habitats containing water bodies. Restricted site replication is a limitation of our study as only two habitats were intensively monitored. Bat activity is temporally and spatially heterogeneous and may vary in other modified environments. However, the intensive monitoring effort in our study provides confidence in observed activity trends, which should be useful for other studies, especially in urban contexts. Our recommendations may also be valid in habitat types where long-tailed bat activity patterns are similar (e.g. plantations; Borkin & Parsons Citation2009).
Conclusion
Monitoring recommendations developed in our study should enable practitioners to better focus bat monitoring effort during times and at locations of peak activity. This should maximize and improve: recordings of long-tailed bat echolocation pulses for bioacoustic analyses, detector-based presence/absence surveys, and capture rates.
Acknowledgements
We thank Associate Professor Stuart Parsons and Sarah King for the provision of equipment, several anonymous reviewers who improved earlier versions of this manuscript, David Duganzich for statistical advice, Dudley Bell and the WINTEC arborists for assistance with setting up rope and pulley systems, and Brian Herman for property access. This research was funded by Waikato University, Environment Waikato, The Department of Conservation, Forest and Bird Protection Society (Waikato Conservancy), Opus International Consultants, and Bat Conservation International.
References
- Avila-Flores R, Fenton MB 2005. Use of spatial features by foraging insectivorous bats in a large urban landscape. Journal of Mammalogy 86: 1193–1204. doi:10.1644/04-MAMM-A-085R1.1
- Bartonička T, Zukal J 2003. Flight activity and habitat use of four bat species in a small town revealed by bat detectors. Folia Zoologica 52: 155–166.
- Bat Conservation Trust 2007. Bat surveys: good practice guidelines. 2nd edition. London, Bat Conservation Trust.
- Borkin KM, Parsons S 2009. Long-tailed bats’ use of a Pinus radiata stand in Kinleith Forest: Recommendations for monitoring. New Zealand Journal of Forestry 53: 38–43.
- Broders HG, Findlay CS, Zheng L 2004. Effects of clutter on echolocation call structure of Myotis septentrionalis and M. lucifugus. Journal of Mammalogy 85: 273–281. doi:10.1644/BWG-102
- Clarkson BD, McQueen JC 2004. Ecological restoration in Hamilton City, North Island, New Zealand. In: 16th International Conference Society for Ecological Restoration, pp. 1–6. Victoria, Canada.
- Collins J, Jones G 2009. Differences in bat activity in relation to bat detector height: implications for bat surveys at proposed windfarm sites. Acta Chiropterologica 11: 343–350. doi:10.3161/150811009X485576
- Dekrout A 2009. Monitoring New Zealand long-tailed bats (Chalinolobus tuberculatus) in urban habitats: ecology, physiology and genetics. Unpublished PhD thesis, New Zealand, University of Auckland.
- Fenton MB 2003. Science and conservation of bats: Where to next? Wildlife Society Bulletin 31: 6–15.
- Fukui D, Murakami M, Nakano S, Aoi T 2006. Effect of emergent aquatic insects on bat foraging in a riparian forest. Journal of Animal Ecology 75: 1252–1285. doi:10.1111/j.1365-2656.2006.01146.x
- Gaisler J, Zukal J, Řehák Z, Homolka M 1998. Habitat preference and flight activity of bats in a city. The Zoological Society of London 244: 439–445. doi:10.1111/j.1469-7998.1998.tb00048.x
- Griffiths R 2007. Activity patterns of long-tailed bats (Chalinolobus tuberculatus) in a rural landscape, South Canterbury, New Zealand. New Zealand Journal of Zoology 34: 247–258. doi:10.1080/03014220709510083
- Hecker KR, Brigham RM 1999. Does moonlight change vertical stratification of activity by forest-dwelling insectivorous bats? Journal of Mammalogy 80: 1196–1201. doi:10.2307/1383170
- Hein CD, Castleberry SB, Miller KV 2009. Site-occupancy of bats in relation to forested corridors. Forest Ecology and Management 257: 1200–1207.
- Jones G, Rydell J 1994. Foraging strategy and predation risk as factors influencing emergence time in echolocating bats. Philosophical Transactions of the Royal Society of London: Biological Sciences 346: 445–455. doi:10.1098/rstb.1994.0161
- Kalcounis MC, Hobson KA, Brigham RM, Hecker KR 1999. Bat activity in the Boreal forest: importance of stand type and vertical strata. Journal of Mammalogy 80: 673–682. doi:10.2307/1383311
- Kunz TH 1974. Foraging ecology of a temperate insectivorous bat (Myotis velifer). Ecology 55: 693–711. doi:10.2307/1934408
- Lausen CL, Barclay RMR 2006. Benefits of living in a building: big brown bats (Eptesicus fuscus) in rocks versus buildings. Journal of Mammalogy 87: 362–370. doi:10.1644/05-MAMM-A-127R1.1
- Le Roux DS, Waas JR 2012. Do long-tailed bats alter their evening activity in response to aircraft noise? Acta Chiropterologica 14: 111–120 doi:10.3161/150811012X654321
- Lesinski G 2008. Linear landscape elements and bat casualties on roads – an example. Annales Zoologici Fennici 45: 277–280. doi:10.5735/086.045.0406
- Lloyd B 2009. Bat call identification manual for use with digital bat recorders and BatSearch Software. Wellington, New Zealand, The Department of Conservation.
- MacSwiney MC, Clarke FM, Racey P 2008. What you see is not what you get: the role of ultrasonic detectors in increasing inventory completeness in Neotropical bat assemblages. Journal of Applied Ecology 45: 1364–1371. doi:10.1111/j.1365-2664.2008.01531.x
- Mickleburgh SP, Hutson AM, Racey PA 2002. A review of the global conservation status of bats. Oryx 36: 206–211.
- Milne DJ, Fisher A, Rainey I, Pavey CR 2005. Temporal patterns of bats in the top end of the Northern Territory, Australia. Journal of Mammalogy 86: 909–920. doi:10.1644/1545-1542(2005)86[909:TPOBIT]2.0.CO;2
- Miller BW 1999. A method of determining relative activity of free flying bats using a new activity index for acoustic monitoring. Acta Chiropterologica 3: 93–105.
- Molloy JC 1995. Bat (Peka peka) recovery plan (Mystacina, Chalinolobus). In: Threatened Species Recovery Plan Series No. 15. Wellington, New Zealand, The Department of Conservation.
- Murray SW, Kurta A 2004. Nocturnal activity of the endangered Indiana bat (Myotis sodalis). The Zoological Society of London 262: 197–206. doi:10.1017/S0952836903004503
- O'Donnell CFJ 2000. Influence of season, habitat, temperature, and invertebrate availability on nocturnal activity of the New Zealand long-tailed bat (Chalinolobus tuberculatus). New Zealand Journal of Zoology 27: 207–221. doi:10.1080/03014223.2000.9518228
- O'Donnell CFJ 2001. Advances in New Zealand Mammalogy 1990–2000: long-tailed bat. Journal of the Royal Society of New Zealand 31: 43–57. doi:10.1080/03014223.2001.9517638
- O'Donnell CFJ 2005. New Zealand long-tailed bat. In: King CM ed. The handbook of New Zealand mammals. 2nd edition. Oxford, Oxford University Press. Pp. 98–109
- O'Donnell CFJ, Christie JE, Simpson W 2006. Habitat use and nocturnal activity of lesser short-tailed bats (Mystacina tuberculata) in comparison with long-tailed bats (Chalinolobus tuberculatus) in temperate rainforest. New Zealand Journal of Zoology 33: 113–124. doi:10.1080/03014223.2006.9518435
- O'Donnell CFJ, Christie JE, Hitchmough RA, Lloyd B, Parsons S 2010. The conservation status of New Zealand bats, 2009. New Zealand Journal of Zoology, 37: 297–311. doi:10.1080/03014223.2010.513395
- O'Farrell MJ, Gannon WL 1999. A comparison of acoustic versus capture techniques for the inventory of bats. Journal of Mammalogy 80: 24–30. doi:10.2307/1383204
- O’ Shea TJ, Bogan MA, Ellison LE 2003. Monitoring trends in bat populations of the United States and territories: status of the science and recommendations for the future. Wildlife Society Bulletin 31: 16–29.
- Parsons S, Thorpe CW, Dawson SM 1997. Echolocation calls of the long-tailed bat: a quantitative analysis of types of calls. Journal of Mammalogy 78: 964–976. doi:10.2307/1382956
- Rydell J 1991. Seasonal use of illuminated areas by foraging northern bats Epstesicus nilssoni. Holarctic Ecology 14: 203–207.
- Safi K, Köning B, Kerth G 2007. Sex differences in population genetics, home range size and habitat use of the parti-colored bat (Vespertilio murinus, Linnaeus 1758) in Switzerland and their consequences for conservation. Biological Conservation 137: 28–36. doi:10.1016/j.biocon.2007.01.011
- Scanlon A, Petit S 2008. Effects of site, time, weather and light on urban bat activity and richness: considerations for survey effort. Wildlife Research 35: 821–834. doi:10.1071/WR08035
- Scrimgeour J, Molles L, Waas JR 2013. Vertical variation in flight activity of the lesser short-tailed bat in podocarp and beech forests, Central North Island, New Zealand. New Zealand Journal of Ecology 37(2): 193–198.
- Sedgeley JA 2001. Quality of cavity microclimate as a factor influencing selection of maternity roosts by a tree-dwelling bat, Chalinolobus tuberculatus, in New Zealand. Journal of Applied Ecology 38: 425–438. doi:10.1046/j.1365-2664.2001.00607.x
- Senior P, Butlin RK, Altringham JD 2005. Sex and segregation in temperate bats. Proceedings of the Royal Society 272: 2467–2473. doi:10.1098/rspb.2005.3237
- Sparks DW, Ritzi CM, Duchamp JE, Whitaker Jr. JO 2005. Foraging habitat of the Indiana bat (Myotis sodalis) at an urban–rural interface. Journal of Mammalogy 86: 713–718. doi:10.1644/1545-1542(2005)086[0713:FHOTIB]2.0.CO;2
- Speakman JR, Thomas DW 2003. Physiological ecology and energetics of bats. In: Kunz TH, Fenton MB eds. Bat ecology. Chicago, University of Chicago Press. Pp. 430–490.
- Swift SM 2009. Activity patterns of pipistrelle bats (Pipistrellus pipistrellus) in north-east Scotland. Journal of Zoology 190: 285–295. doi:10.1111/j.1469-7998.1980.tb01428.x
- Turbill C 2008. Winter activity of Australian tree-roosting bats: influence of temperature and climatic patterns. Journal of Zoology 276: 285–290. doi:10.1111/j.1469-7998.2008.00487.x
- Verboom B, Boonman AM, Limpens HJGA 1999. Acoustic perception of landscape elements by the pond bat (Myotis dasycneme). Journal of Zoology 248: 59–66. doi:10.1111/j.1469-7998.1999.tb01022.x
- Vindigni MA, Morris AD, Miller DA, Kalcounis-Rueppell MC 2009. Use of modified water sources by bats in a managed pine landscape. Forest Ecology and Management 258: 2056–2061. doi:10.1016/j.foreco.2009.07.058