ABSTRACT
The abundance and distribution of aquatic insects may be controlled by factors at any stage of the life cycle. To understand the potential role of factors affecting egg and adult stages and oviposition, basic information on egg characteristics and oviposition behaviour is required. However, published information on New Zealand Ephemeroptera, Plecoptera and Trichoptera (EPT) is scattered and very limited. This review compiles current knowledge of New Zealand EPT egg characteristics and oviposition behaviour using published information and unpublished observations. Where direct observations are lacking, information on closely related species overseas, and inferences based on female morphology, are included. Eggs show a variety of physical characteristics that may influence egg retention, transport and distribution. Oviposition behaviour is highly selective among some taxa, but appears generalist among others, though the latter may still require specific cues. Identifying and providing for adult, egg and oviposition requirements may be fundamental to re-establishing EPT populations in restored aquatic habitats.
Introduction
Understanding the processes that regulate population densities and distributions is challenging for species with complex life cycles (Lancaster et al. Citation2010a). For most aquatic insects, different life stages inhabit different environments and are exposed to different sources of mortality. Understanding the pressures on one life stage, therefore, may not provide any information regarding pressures on another stage. To determine the points at which population regulation occurs, we need to understand events and their effects occurring at all life stages, as well as how population fluctuations in early life stages affect later ones (Lancaster et al. Citation2010a). This information will greatly improve our understanding of the processes that drive patterns of abundance and distribution (Heino and Peckarksy Citation2014).
Although models of population dynamics in marine and lentic freshwater invertebrates have shown the importance of processes at various life stages (Reich and Downes Citation2003b and references therein; Lancaster et al. Citation2010a), stream ecologists have typically assumed that, for lotic insects, population densities are set by the instream habitat needs of larvae (review in Lancaster and Downes Citation2013). However, a number of aquatic insect taxa are known to be highly selective in their choice of oviposition habitat (Reich and Downes Citation2003a; Lancaster and Downes Citation2013), behaviour that implies strong selection pressure resulting from either variable mortality among ovipositing females or differential survivorship among egg or neonate larvae in different places (Lancaster et al. Citation2010b). Although the ultimate consequences of such selectivity are poorly known (Lancaster et al. Citation2010b), the existence of selective behaviour implies that populations are influenced by events during either the adult or egg stage.
The abundance and distribution of aquatic insect populations may be affected by the physical characteristics of eggs and egg masses as well as by oviposition behaviour. Egg size and number can affect the fitness of ovipositing adults and their offspring (Reich Citation2004). Egg size, density, coating and attachment structures also determine the way eggs are retained or transported within the stream, affecting distribution patterns of neonate larvae (Lancaster and Downes Citation2013). After oviposition, eggs are exposed to a variety of risks, including dislodgement, predation, desiccation and disease; therefore, the way the eggs are packaged, and the development time of embryos, may influence rates of hatching success (Reich Citation2004; Lancaster and Downes Citation2013). In addition, egg morphology correlates with female genital morphology, which often relates in predictable ways to oviposition behaviour and habitat. Understanding the basic characteristics of eggs, therefore, can provide valuable information on a species’ fecundity, and the spatial, temporal, physiological and evolutionary constraints on potential recruits.
Understanding the controls on population dynamics of aquatic insects has recently gained an important application in stream management. Research has begun to show that in many stream restoration projects, self-sustaining populations of aquatic insects are failing to re-establish (Parkyn et al. Citation2003; Palmer et al. Citation2010; Louhi et al. Citation2011; Stranko et al. Citation2012; Giling et al. Citation2016) for reasons that are not well understood (Feld et al. Citation2011). The influence of the terrestrial adult stage as an important coloniser of new habitats and a potential determinant of larval abundance and distribution is now widely acknowledged (Reich Citation2004 and references therein). For species that oviposit on specific substrates, lack of such substrates may, in some situations, limit egg supply and population densities (Lancaster et al. Citation2010a; Heino and Peckarsky Citation2014), potentially limiting recolonisation of restored reaches (Purcell et al. Citation2002; Blakely et al. Citation2006). Yet very few stream restoration projects explicitly provide for egg or adult life stages, or for insect oviposition. Greater understanding of the habitat requirements for insect colonisation and recruitment may reveal that lack of suitable habitat is a critical factor underlying the lack of recolonisation in many stream restoration projects, and that greater success can be achieved by providing such habitat.
Despite the importance of basic information on egg characteristics and oviposition behaviour, there is a paucity of accessible information for aquatic insects globally, more so in the southern hemisphere (Reich Citation2004), and particularly in New Zealand. Even among population studies that try to integrate multiple life history stages, information on eggs is lacking for most macroinvertebrate species (Reich Citation2004). Oviposition is rarely observed in aquatic insects as it often occurs at night or early morning, and observations either remain unpublished or are published in widely scattered fragments and in obscure locations. Female genitalia can sometimes be used to infer oviposition behaviour, but female genitalia are often ignored as they are not used as much as male genitalia for species-level identification (e.g. Holzenthal et al. Citation2015).
This review presents a synopsis of the egg characteristics and oviposition habitats and behaviour of the aquatic insect orders Ephemeroptera, Plecoptera and Trichoptera in New Zealand, which represent about 400 endemic species. Our aim is to provide information that can be used to generate hypotheses about insect population dynamics involving interactions among different life stages, and also to enable the detection, identification and quantification of insect eggs in the field for testing hypotheses. We recognise that estimating egg abundances and distribution from field sampling is extremely challenging for those species that scatter their eggs across the water surface. However, future sampling technologies may enable this, and with more detailed information on size, density, stickiness and morphology, hydraulic models may be employed to predict distributions.
The information in this review has been compiled from local and international literature, and unpublished theses, as well as personal observations and unpublished data. Major knowledge gaps remain, and to begin filling these, egg characteristics and oviposition habitats and behaviour have been inferred from related non-New Zealand taxa in some cases. In other cases, morphological traits expressed by some female aquatic insects, such as limb modification (for swimming) or structure of terminal abdominal segments (e.g. an ovipositor), have been used to infer oviposition behaviour, habitat preference, egg or egg mass appearance, or method of egg delivery.
Discussion
Ephemeroptera
Fifty-four species of Ephemeroptera or mayflies are known from eight families in New Zealand (Hitchings et al. Citation2015; Hitchings and Hitchings Citation2016) with many families having ancestral Gondwanan connections (Peters and Campbell Citation1991; Towns and Peters Citation1996). Mayfly eggs are oval to elongate or fusiform (spindle-like) and the chorion (shell) exhibits species-specific patterning and reticulation (Towns and Peters Citation1996). Eggs of some species have spring-coiled, fibre-like structures that unravel on contact with water to act as an attachment or anchor devices (Brittain Citation1982; Lancaster and Downes Citation2013).
In terms of oviposition, mayflies are classified into bombers, dippers, floaters, landers and splashers (Encalada and Peckarsky Citation2007). New Zealand mayflies have not been classified according to this scheme but observations of oviposition in New Zealand are limited, even for Leptophlebiidae, the most species-rich family, perhaps because oviposition often occurs at dawn or after sunset (Wisely Citation1961; Norrie Citation1969). However, based on observations by Phillips (Citation1930a, Citation1930b), Wisely (Citation1961), Mclean (Citation1967) and Chisholm (Citation1984) it would appear that most New Zealand species are ‘dippers’ while only one species is confirmed as a ‘bomber’ and another a ‘floater’. Dipping entails the female dipping her abdomen into the water as she skims over the water surface, releasing small batches of eggs each time (Brittain Citation1982; Encalada and Peckarsky Citation2007). Bombing involves the female releasing the entire extruded egg mass in a one-off event either from the air or on contact with water (Encalada and Peckarsky Citation2007). Underwater oviposition in mayflies (i.e. ‘landers’, sensu Encalada and Peckarsky Citation2007) is unusual and known only from the northern hemisphere Baetidae (Peckarsky et al. Citation2000), and therefore unlikely to occur in New Zealand species. The presence of an ovipositor in two leptophlebiids species introduces a third oviposition category, ‘surface penetrators’, for New Zealand mayflies.
Ameletopsidae
The eggs of Ameletopsis perscitus Eaton, 1899, the only New Zealand representative of this family, are small and oval (0.21 × 0.15 mm) (Phillips Citation1930a). Coiled attachment structures are absent from the egg but the large mesh reticulation present on the chorion is adhesive, enabling egg attachment to submerged structures (Koss and Edmunds Citation1974 and SEM photos within). No information is available on fecundity of A. perscitus, but Mirawara, an Australian genus, is known to produce up to 8000 eggs (Peters and Campbell Citation1991). Nothing is known of the oviposition habitat or behaviour of A. perscitus, but Mirawara is known to oviposit during the day, depositing small batches of eggs on the water surface at the tails end of pools (Riek Citation1970; Peters and Campbell Citation1991).
Coloburiscidae
Eggs and egg masses of the ubiquitous Coloburiscus humeralis Walker, 1853, the only representative of this family in New Zealand, are possibly the best studied among the New Zealand mayflies (see Phillips Citation1930a; Wisely Citation1961, Citation1965; McLean Citation1967; Norrie Citation1969). Female C. humeralis are active at both dawn and dusk, carrying a large bright orange egg mass () of 3000–6000 eggs (Wisely Citation1961). Eggs are released in a single oviposition event (‘bombing’) as the female flies over a riffle touching the water with her egg mass (Wisely Citation1961). Oviposition by splashing in New Zealand mayflies is so far known only for C. humeralis. The egg mass remains intact as it sinks, but no indication was given by Wisely (Citation1961) or Mclean (Citation1967) as to how long the egg mass remains intact before it disintegrates and the individual ellipsoid eggs (0.20–0.25 × 0.10–0.15 mm; Wisely Citation1961) are dispersed. Terminal pads attached to three or four loosely coiled spring-like filaments at the poles of the egg become sticky once wet (Koss and Edmunds Citation1974 and SEM photos within; Wisely Citation1961), and are likely to aid in the retention of the egg between the interstitial spaces in riffles where the nymphs are primarily found (Wisely Citation1961; Mclean Citation1967).
Ichthybotidae
This small endemic family of burrowing mayflies is represented by Ichthybotus, New Zealand’s largest mayfly, containing two species (Hitchings et al. Citation2015). Ichthybotus eggs are large and oval (0.37 × 0.19 mm) with a thick, mostly smooth chorion (6–7 µm) that lacks attachment threads (Phillips Citation1930a; Koss and Edmunds Citation1974; Chisholm Citation1984). Egg attachment to the substrate is by an adhesive layer mostly concentrated at the egg poles (Koss and Edmunds Citation1974 and SEM photos within).
Female Ichthybotus hudsoni (McLachlan, 1894) oviposit at dusk by either ‘crash landing’ on to the water surface or gently alighting (‘floater’, sensu Encalada and Peckarsky Citation2007), releasing almost all eggs 30 seconds after landing (Chisholm Citation1984). Unfortunately, no information is available on the fecundity of I. hudsoni.
Due to the lack of attachment structures on I. hudsoni eggs, Chisholm (Citation1984) calculated an egg was capable of travelling up to 20 m before settling on the stream bed. Oviposition habitat is therefore likely to occur in slow flowing shallow water in the vicinity of the preferred nymphal habitat of accumulated fine gravels, sand and detritus. Furthermore, released eggs are likely to become sticky once wet, particularly at the poles, and eventually covered with sand grains and detritus. This may help retain the egg in favourable habitat and provide camouflage from predators. Very small I. hudsoni nymphs are present almost throughout the year despite adults being active only in summer, suggesting egg diapause occurs (Chisholm Citation1984).
Leptophlebiidae
Leptophlebiidae is the most species-rich mayfly family in New Zealand with nearly 40 species recognised (Hitchings et al. Citation2015). Leptophlebiidae eggs are mostly oval to fusiform (spindle-like) with the chorion (egg shell) showing species-specific patterns and sculpturing that aid in adhesion and retention of the egg (Koss and Edmunds Citation1974). In New Zealand species, the chorion can range from scale-like attachment structures to slightly raised circular, stellate or paired attachment structures, and in some species the eggs are strongly ridged (Towns Citation1983; Towns and Peters Citation1996). The eggs of Neozephlebia scita Walker, 1853, one of the most widespread species, are unique within New Zealand Leptophlebiidae due to extremely long, irregularly coiled, thread-like structures present at the egg poles (Phillips Citation1930b; Towns Citation1983; Hitchings et al. Citation2015). A polar concentration of attachment threads are thought to anchor the egg in a position of least resistance to flow (Koss and Edmunds Citation1974), and this may explain the ability of N. scita to colonise an extremely wide range of habitats including pools and riffles in streams from sea-level to almost 1000 m (Towns Citation1983; Towns and Peters Citation1996).
Almost all Leptophlebiidae are considered dippers, releasing a few eggs each time the female’s abdomen touches the water surface (Riek Citation1970; Brittain Citation1982; Peters and Campbell Citation1991). Dipping has been observed at the side of pools for the New Zealand species Acanthophlebia cruentata (Hudson, Citation1904) (e.g. McLean Citation1967) and over a run section of a large river by Austroclima sepia (Phillips, 1930) (B. J. Smith, pers. obs.). Additionally, imagines of A. sepia, Austronella planulata Towns, Citation1983 and Deleatidium sp., each with an extruded egg mass, have been collected from sticky sheets suspended just above the water of a small stream (National Institute of Water and Atmospheric Research [NIWA], unpubl. data) suggesting dipping also occurs in these genera. Leptophlebiidae fecundity is highly variable among species, for example Deleatidium vernale Phillips, 1930 can produce 600–800 eggs, whereas around 2000 eggs have been reported for Zephlebia versicolor (Eaton, 1899) (Phillips Citation1930a, Citation1930b).
Most, but not all, Leptophlebiidae females have a short egg-guide on sternite seven. The egg-guide is extended to form a short ovipositor-like structure in Tepakia caligata Towns and Peters, Citation1996 and Isothraulus abditus Towns and Peters, 1979 (Towns and Peters Citation1996) with an internal telescopic structure (B. J. Smith, pers. obs.; ). An analogous structure is known from an Australian leptophlebiid (Riek Citation1970) and a small telescopic structure is present within the ovipositor of a European species (Gaino et al. Citation2009). Mayflies use telescopic ovipositors to break the water surface tension and allow oviposition to occur below the water (Riek Citation1970). The presence of a short ovipositor in Tepakia and Isothraulus suggests these species may have a more specialised or selective oviposition behaviour, such as that described above by Riek (Citation1970) or ovipositing in the cracks and crevices of emergent or instream structures. It is suggested that a third behavioural category of oviposition, ‘surface penetrators’, be assigned to New Zealand Leptophlebiidae with a telescopic ovipositor.
Oniscigasteridae
Two species within the endemic genus Oniscigaster are known from New Zealand (Hitchings et al. Citation2015). Oniscigaster have large, oval eggs (0.29 × 0.16 mm) and a female can produce between 6000–8000 eggs (Phillips Citation1930a). Oniscigaster eggs are smooth with a relatively unsculptured chorion (Phillips Citation1930a; Peters and Campbell Citation1991) and the lack of coiled attachment structures (Koss and Edmunds Citation1974 and SEM photos within) may account for McLean’s (Citation1970) observation that Oniscigaster eggs do not adhere to stones. Oniscigaster oviposition habitat or behaviour has not been observed, but the absence of egg attachment features suggest the preferred oviposition habitat could be slow flowing, very shallow water (≤ 10 mm) on fine sand and silt where small nymphs (< 10 mm) are also abundant (McLean Citation1970).
Nesameletidae
Nesameletidae is represented in New Zealand by the single genus Nesameletus with five described species (Hitchings and Staniczek Citation2003). Terminal spring-like coils of the fibre-clusters are present on Nesameletus eggs (Koss and Edmunds Citation1974 and SEM photos within; Hitchings and Staniczek Citation2003) most likely activating on contact with water to aid in the attachment of the egg to instream substrate. Nesameletus are considered dippers, releasing eggs on the water surface in areas of reduced flow (Hitchings and Staniczek Citation2003) so do not fix their eggs to the substrate via a cement-like substance as reported by Phillips (Citation1930a) and Winterbourn (Citation2003).
Rallidentidae
This endemic family is represented by a single genus, Rallidens, with two species (Staniczek and Hitchings Citation2013). The eggs of Rallidens have knob-terminated coiled attachment threads present at egg poles (Koss and Edmunds Citation1974 and SEM photos within; Staniczek and Hitchings Citation2013). These structures are thought to aid in attaching eggs to stream substrates. The nymphs of Rallidens are more commonly collected from pools as well as riffles and runs with reduced velocities (Staniczek and Hitchings Citation2013), and ovipositing females have been observed repeatedly dipping in pools and back waters with a flight pattern that ensures eggs are widely scattered (McLean Citation1967).
Siphlaenigmatidae
No direct observations have been recorded of the oviposition habitat or behaviour of Siphlaenigma janae Penniket, 1962, the only species in this endemic family. However, egg morphology provides some indication of egg dynamics after oviposition. Fibre-coiled attachment threads, thought to aid the attachment of the egg to instream substrate, uniformly cover the egg, though adhesive terminal fibre clusters or knobs, as seen in Rallidentidae, are absent (Koss and Edmunds Citation1974 and SEM photos within).
Plecoptera
The New Zealand plecopteran fauna is represented by at least 120 species in four families, Austroperlidae, Eustheniidae, Gripopterygidae and Notonemouridae, which have an almost exclusively southern hemisphere distribution (McLellan Citation2006). In regard to oviposition, the New Zealand stoneflies are the least understood among the three aquatic insect orders discussed in this review. Information consists of several descriptions of eggs and a few descriptions of oviposition for New Zealand species, discussed within accounts of southern hemisphere stoneflies. However, most information on egg characteristics and oviposition is for Australian species (see Hynes Citation1974).
Many stoneflies carry an extruded egg mass on an upturned abdomen and scatter eggs across the water surface in a single oviposition event (Hynes Citation1974), but some species have an ovipositor (see McLellan Citation1991), suggesting the female is highly selective when choosing an egg-laying habitat. Australian stoneflies are thought to oviposit at night or in the early morning (Hynes Citation1974, Citation1976), and in the absence of direct observations this is assumed to be true for the New Zealand fauna. The individual eggs of some species may be encapsulated in spumaline, a gelatinous material (Hynes Citation1974). The chemical composition of stonefly spumaline is unknown but in Trichoptera consists primarily of complex polysaccharides and glycoproteins, where the composition can vary between species (Hinton Citation1981).
Egg maturation for many stonefly species is conditional on adult feeding (e.g. Hynes Citation1974; MacNeale et al. Citation2002) and between 30%–50% of female reproductive biomass has been attributed to terrestrial feeding (Oberndorfer and Stewart Citation1977; Beer-Stiller and Zwick Citation1995). Some New Zealand female stoneflies emerge with immature ovarioles and are capable of surviving several weeks (Smith and Collier Citation2000; NIWA, unpubl. data) moving into the riparian vegetation to feed on sooty mould fungi, fungal spores, pollen and fine particulate organic matter (Smith and Collier Citation2000; Winterbourn Citation2005). Therefore, successful egg production requires suitable food resources to be available in the riparian zone, and to re-establish stonefly populations, stream restoration must provide for adult feeding as well as oviposition requirements.
Austroperlidae
Austroperlidae are present in Australia, South America and New Zealand, but only one species, Austroperla cyrene (Newman, 1845), is known from New Zealand (McLellan Citation1997). The only information on egg morphology of A. cyrene is from Thomson (Citation1934), who figured an egg in vivo but gave no other details. No descriptions of South American Austroperlidae eggs are available, and therefore the following accounts of egg characteristics and oviposition behaviour are from four Australian Austroperlidae genera but are likely to apply or overlap with those of A. cyrene. The eggs of Australian Austroperlidae are white, spherical to oval and encased in either a thick and non-sticky or thin and sticky spumaline (gelatinous) coating (Rait Citation1937; Hynes Citation1976). Ovipositors are absent from all Austroperlidae. Some species lay non-sticky eggs as a single batch at the water’s edge and these disperse on contact with water (Rait Citation1937). The eggs of other Australian species have a sticky spumaline coating and have been found adhered to rocks. The spumaline dissolves in a few days releasing the eggs, as reported for other stoneflies (Hynes Citation1974).
Adult A. cyrene are known to feed on wood (Thomson Citation1934), and although females can live up to 69 days on a weak sucrose solution they do not produce eggs (B. J. Smith, unpubl. data). Australian Austroperlidae feed on fungi-infested wood before producing eggs (Hynes Citation1974), and a specific diet may also be required for egg production in A. cyrene. Given that Austroperlidae adults consume decaying wood and A. cyrene nymphs have an affinity with wood (e.g. Anderson Citation1982; Collier and Smith Citation2003), females are likely to oviposit on emergent wood or near to instream woody resources. Since adult feeding is obligatory for ovary development and egg production in Austroperlidae, stream restoration projects will need to provide food resources for these and other long-lived stonefly species.
Eustheniidae
The large, typically green Stenoperla adult is one of New Zealand’s most easily recognised stoneflies and the only genus of this family present in New Zealand. Four species are known (McLellan Citation1996) but only for the ubiquitous Stenoperla prasina (Newman, 1845) has the life history and egg been described in any detail (Helson Citation1935).
Up to 75 shiny, dark brown, heavily sclerotised eggs (0.6 × 0.3 mm) are produced by S. prasina, each encapsulated in a thick spumaline coating that dissolves after 24 hours (Helson Citation1935). Spumaline may assist attachment to a substrate or protect the egg as it sinks and settles on the stream bed (Hynes Citation1974). A spumaline coating has not been confirmed for the remaining three, more closely related, species.
Female Stenoperla lack an ovipositor, and an extruded egg mass is carried subterminally on the ventral surface of an up-turned abdomen (Helson Citation1935). Stenoperla prasina is crepuscular (Helson Citation1935) and, typically, eggs are scattered at the water surface and attach to submerged objects (Pendergrast and Cowley Citation1966). Tillyard (pers. corres. to Rait Citation1937) noted that S. prasina used an emergent rock to enter the water to lay a smooth compact mass of pear-shaped eggs. However, given the eggs of Stenoperla are smooth, circular (bi-convex disc) and lens-shaped when viewed laterally (Helson Citation1935; Zwick Citation1979; McLellan Citation1996), the accuracy of Tillyard’s observation is questionable. The eggs of other Eustheniidae taxa, such as Cosmioperla from Australia and Neuroperla from Argentina and Chile also have a similar general appearance to Stenoperla (McLellan Citation1996; Stark et al. Citation2009).
Mature eggs have been recorded from late instar nymphs of the closely related Australian Cosmioperla, allowing females to oviposit within 3 days of emerging (Hynes Citation1974; Zwick Citation1979). Eggs are variably present in mature nymphs of S. prasina () and S. maclellani Zwick, Citation1979 (Helson Citation1934; Zwick Citation1979) suggesting these species also can oviposit shortly after emergence.
Adult Stenoperla have functioning mouthparts and S. prasina and S. maclellani have been collected with full guts (Zwick Citation1979) indicating adults may move into the riparian zone to feed. Stenoperla prasina can live up to 38 days in the laboratory on a weak sucrose solution, but without producing eggs (B. J. Smith, unpubl. data). Most Australian eusthenids are known to consume fungal-infected wood (Hynes Citation1974) and since some New Zealand Eustheniidae females emerge with an immature reproductive system, a specialist diet may be required for ovariole development and egg production. Adult feeding may be less of a requirement for species with eggs already present in mature nymphs.
Gripopterygidae
Gripopterygidae is the most species-rich of the New Zealand stonefly fauna, represented by 12 genera containing over 70 species (McLellan Citation2006). The eggs of only five New Zealand Gripopterygidae species have been mentioned or briefly described: Zelandobius pilosus Death, Citation1990 (Furcillatus group) (Death Citation1990); Zelandoperla agnetis McLellan, Citation1967 (Stark et al. Citation2009); Megaleptoperla grandis (Hudson, 1913) (Michaelis Citation1984); and the two alpine species Holcoperla angularis Wisely, Citation1953 (Wisely Citation1953; Stark et al. Citation2009) and Apteryoperla monticola (Wisely, Citation1953) (Wisely Citation1953). Eggs of Australian Gripopterygidae have been described in considerably more detail. They are variously shaped, including spherical, flat, pie, cone and bun shaped with the chorion of some species displaying elaborate sculpturing (Hynes Citation1974). Considerable variation of the chorion, from relatively simple, unadorned surface to elaborate structure with prominent follicle cell impressions and equatorial rings, are also present in Neotropical Gripopterygidae; however, the chorion of Z. agnetis is smooth with micropyles present as minute pores without additional modification (Stark et al. Citation2009).
Hynes (Citation1974) claimed sticky or spumaline-covered eggs (which may aid attachment to substrates) are absent from Gripopterygidae. However, the small (0.3 mm) tuberculate-sculptured, spherical eggs of Z. pilosus, which are laid singly or as a coherent mass (up to 156 eggs), have been observed stuck to a petri dish with a ‘jelly-like substance’ (Death Citation1990). A basal cap or collar-like structure analogous to attachment structures seen in some northern hemisphere stoneflies (e.g. Lancaster and Downes Citation2013, fig. Citation11.Citation4) are visible on the egg figured by Death (Citation1990) and may assist adhesion to the spumaline. Characteristics of Z. pilosus eggs and egg masses may or may not be similar to other species within the Furcillatus group, as attachment structures were absent from the weakly bi-convex, disc-shape egg (0.24 × 0.14 mm) dissected from a mature Z. furcillatus Tillyard, 1923 (B. J. Smith, unpubl. data).
Prolonged retention of eggs leading to advanced embryonic development is widely documented in northern hemisphere Capniidae and Leuctridae stoneflies (Caires et al. Citation2016), but considered a rare phenomenon in southern hemisphere stoneflies. However, advanced egg development in gripopterygids has been documented for three species, one species each from South American (Zwick Citation1973), Tasmania (Zwick Citation1973; Hynes Citation1974) and New Zealand (Michaelis Citation1984). Advanced egg development occurs in the last instar nymph of both Megaleptoperla species (McLellan Citation1977), but only the eggs of M. diminuta Kimmins, 1938 have been described. The adult female of M. diminuta produces white, oval eggs (0.42 mm long), each with a well-developed first instar nymph with distinguishable eyespots (Michaelis Citation1984). Unfortunately, no further details of M. diminuta eggs were provided by Michaelis (Citation1984). In addition to M. diminuta, advanced embryonic development has been observed for a second New Zealand gripopterygid, Zelandoperla decorata Tillyard, 1923, by one of the authors (B. J. Smith, pers. obs.). A single female was collected at the water edge ovipositing a slightly oval white egg (0.26 × 0.34 mm) with developed eyes (). Whether advanced oviparity is obligate or facultative in this species remains to be determined. Adults need to be long-lived for advanced egg development to occur (Michaelis Citation1984), and terrestrial feeding may be necessary for longevity and egg production (e.g. Beer-Stiller and Zwick Citation1995; Smith and Collier Citation2000; Winterbourn Citation2005).
Figure 4. Zelandoperla decorata (Plecoptera: Gripopterygidae) extruding egg (arrow); insert showing well-developed egg with visible eyespots (arrow).
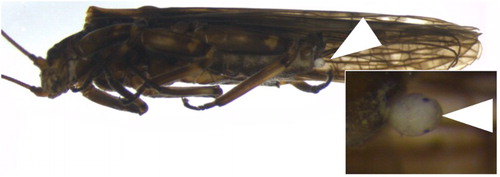
Wisely (Citation1953) described the eggs of the two alpine stoneflies, H. angularis and A. monticola, as circular but lens-shaped laterally (0.20 × 0.36 mm). Apteryoperla monticola eggs are white with a thin chorion and lack a basal plate or adhesive knobs (Wisely Citation1953). The eggs of H. angularis are transparent (Wisely Citation1953) and SEM microphotography also suggests a thin chorion with no collar, and a rough granular surface that lacks micropyles (Stark et al. Citation2009). A spumaline coating was not confirmed for the egg of either species by Wisely (Citation1953).
Female Gripopterygidae normally carry a single mass of extruded eggs attached to the underside of upturned terminal abdomen segments (Riek Citation1970; Hynes Citation1974). Some eggs may be released singly for some New Zealand species as noted for Z. decorata (this review) and Z. pilosus, as reported by Death (Citation1990). However, more typically, oviposition probably occurs shortly after the egg mass has been extruded when the female’s abdomen contacts the water surface, releasing eggs as a single batch (Riek Citation1970; Hynes Citation1974). Gripopterygidae eggs have been collected individually from the stream bed, suggesting the eggs either disperse on contact with water or shortly after the egg mass sinks and disintegrates (Hynes Citation1974). Unlike most gripopterygids, the alpine H. angularis retains eggs internally within an abdominal pouch formed by a modification of sternite nine (Wisely Citation1953). The oviposition behaviour of M. diminuta is not known but eggs appear to be held by a curved rim on the inside of the large sternite eight (Michaelis Citation1984, fig. Citation1). This could suggest mature eggs are extruded as a batch prior to oviposition on instream vegetation, the preferred habitat of the nymph (McLellan Citation1975).
The nymphs of Acroperla trivacuata (Tillyard, 1923), Nesoperla fulvescens Tillyard, 1923 and Taraperla howesi (Tillyard, 1923) have mostly severed their aquatic connections, with all but the earliest instars showing a high tendency towards terrestrialism (McLellan Citation1998; Winterbourn Citation2010). Early instar nymphs of T. howesi are associated with pool habitats dominated by sand and silt (McLellan Citation1998; Winterbourn Citation1966, Citation2010) and an adult female was collected from a pool by Winterbourn (Citation2010). Therefore, pools are the most likely oviposition habitat for T. howesi. The oviposition habitat for A. trivacuata is unknown, but 80% of the two smallest nymphal size classes are found mid-stream (Winterbourn Citation1966). While this could suggest this is the preferred oviposition habitat, disparity between oviposition and neonate habitat have been reported for some aquatic taxa (Lancaster et al. Citation2010a).
The following observations and egg measurements of A. trivacuata and N. fulvescens are from eggs dissected from mature females by one of the authors (B. J. Smith, pers. obs.). Eggs of A. trivacuata are spherical (0.22 mm diameter) and lack attachment structures (e.g. basal cap or collar) or show advanced development indicating ovoviviparity. Acroperla trivacuata eggs laid in summer do not hatch until autumn (McLellan Citation1998) indicating either an extremely long incubation time or egg diapause. Eggs dissected from mature N. fulvescens are sub-spherical (0.26 mm diameter) and also lack attachment structures. Nesoperla fulvescens eggs do not show advanced development to indicate ovoviviparity. Gravid females of A. trivacuata and N. fulvescens have been collected with food items in the gut (), which may also suggest feeding is required for longevity and egg production, as noted for other adult stonefly species (e.g. Hynes Citation1974; Caires et al. Citation2016). The habitats for oviposition and early instar nymphs of N. fulvescens are unknown (Winterbourn Citation2010).
New Zealand Gripopterygidae associated with harsh environments such as high altitudes (e.g. Vesicaperla) and sub-Antarctic islands (e.g. Aucklandobius) are often wingless (apterous), and their mostly terrestrial nymphs are found in damp, humid conditions under stones and amongst decaying vegetation (e.g. Wisely Citation1953; Illies Citation1963; McLellan Citation2003, Citation2006). Vesicaperla are unique within the Gripopterygidae in that females of three of the seven known species have rigid ovipositors that range from small and rudimentary (V. celmisia McLellan, Citation2003 and V. trilinear McLellan, Citation2003) to well-defined and extending beyond the end of the abdomen (V. substirpes McLellan, Citation1967) (McLellan Citation1967, Citation2003). The presence of ovipositors and the distribution of nymphs and adults suggest females are likely to exhibit highly specialised or selective oviposition behaviour. Nymphs and adults of V. celmisia and V. trilinear are associated with Haast’s mountain daisy (Celmisia haasti Hook. f.) and oviposition is likely to occur within or around this alpine plant. Decaying vegetation is the preferred habitat for V. substirpes (McLellan Citation1967) and the long ovipositor may be used in part to insert eggs deep within decomposing vegetation, perhaps to facilitate incubation or avoid opportunistic predation. The preferred nymphal habitat for the remaining Vesicaperla species (which lack ovipositors) is alpine leaf litter and roadside ferns (Blechnum novae-zealandiae Chambers and Farrant), and, as for the other species, oviposition is likely to occur in areas of high humidity within leaf litter near the nymphal habitat.
Notonemouridae
New Zealand Notonemouridae comprises seven genera and 29 species (McLellan Citation2006). The eggs and oviposition of New Zealand Notonemouridae are not described, and only in Australia have eggs of some Notonemouridae species been studied in detail. The following observations and measurements are from eggs dissected from mature females by one of the authors (B. J. Smith, pers. obs.). The eggs of Spaniocerca zelandica Tillyard, 1923 (0.22 × 0.14 mm), S. zwicki McLellan, Citation1991 (0.16 × 0.14 mm), Omanuperla bruningi McLellan, 1972 (0.20 × 0.16 mm) and Notonemoura latipennis Tillyard, 1923 (0.20 × 0.16 mm) are small and (often irregularly) oval, and the egg contents completely fill the egg. Eggs of Spaniocercoides watti McLellan, 1984 are small, shiny and irregularly oval (0.2 × 0.16 mm) and the egg contents are partially detached from the chorion. The eggs of Halticoperla viridans McLellan and Winterbourn, 1968 are irregularly oval (0.24 × 0.20 mm) and coated with what appears to be an outer spumaline layer, although this could not be confirmed.
All New Zealand Notonemouridae, with the exception of Otehiwi sagattarius McLellan, Citation2003, are characterised by a modification of the sub-genital plate to form an ovipositor. Oviposition has not been observed for New Zealand Notonemouridae but oviposition strategies may be inferred based on nymphal habitats, ovipositor morphology and observations of Australian species by Hynes (Citation1974). Notonemouridae ovipositors can range from short and pointed (some Spaniocerca), long and straight with chitinous reinforcing strips (Notonemoura; ) to curved and fully sclerotised (Halticoperla; ) (see McLellan Citation1991, Citation2000). Females most likely use their specialised egg-laying structures to oviposit in the cracks and crevices of wood or rocks at the water’s edge. Ovipositor morphology has been correlated with specific egg-laying habitat in some caddisflies (Spänhoff and Alecke Citation2004) and this is also likely to be true for Plecoptera where differences in oviposition microhabitat may reduce competition between related species (Zwick Citation2015). Notonemouridae nymphs are often associated with thin films of water, bogs, seepages and springs, while other species are hyporheic and some can live out of the water under damp rocks (McLellan Citation1991, Citation2000). It is likely that the adults oviposit near the nymphal habitats.
Figure 6. Notonemoura latipennis (Plecoptera: Notonemouridae). A, Female with ovipositor (arrow); B, magnified view of ovipositor with eggs visible (arrow).
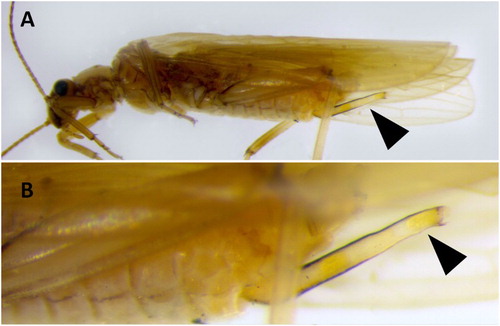
Hynes (Citation1974) reported oviposition for four Australian species within Austrocerca, Austrocercella and Notonemoura (the latter genus being shared with New Zealand). Using an ovipositor, female Austrocercella and Notonemoura lay a single spumaline-coated egg within wet or damp cracks and crevices in bark at the water’s edge where post-oviposition swelling of the spumaline locks the egg in place (Hynes Citation1974). New Zealand species with ovipositors are also likely to exhibit similar egg-laying behaviour, exploiting similar oviposition habits. Females that lay single eggs may minimise predation risk by either laying eggs in multiple habitats or ovipositing over several nights or a combination of both. Winterbourn’s (Citation2005) collection of S. zelandica and Cristaperla fimbriata (Winterbourn, 1965) with abdomens partially full of eggs, suggesting multiple oviposition events, provides some evidence of such a strategy.
Ovovivipary, apparently rare in southern hemisphere stoneflies, has been documented for Austrocerca tasmanica (Tillyard, 1924) (Hynes Citation1974), and in this case it may be linked with the lack of an ovipositor in the female. Female A. tasmanica lay a non-spumaline coated batch of mature eggs (nymphs with developed eyes), but unlike Australian notonemourids with ovipositors, A. tasmanica eggs are not laid in crevices (Hynes Citation1974), and thus are exposed to predation. Whereas 2 to 4 weeks incubation is required for some Australian Notonemouridae eggs laid via an ovipositor (Hynes and Hynes Citation1975), hatching may occur shortly after oviposition by A. tasmanica due to the advanced developmental stage of the eggs. Ovoviviparity therefore may reduce predation risk.
Trichoptera
Trichoptera or caddisflies are the most diverse of the New Zealand aquatic insect orders with approximately 250 species known (Smith Citation2014). They produce some of the most recognisable aquatic insect eggs and have a diverse array of egg delivery methods with the terminal segments of some species modified into a protrusible oviscapt, an ovipositor-like structure (Holzenthal et al. Citation2007). Egg mass morphology in caddisflies reflects the classification of the order proposed by Frania and Wiggins (Citation1997) following the larval morphology in sub-orders Annulipalpia, Integripalpia and Spicipalpia. However, a more recent phylogenetic study implies only Annulipalpia and Integripalpia are considered monophyletic clades, with Spicipalpia considered a clade within the latter (Holzenthal et al. Citation2007).
Annulipalpia comprise caddisflies whose larvae are fixed retreat-makers and net-spinners and in New Zealand includes Ecnomidae, Hydropsychidae, Philopotamidae and Polycentropodidae. The eggs of Hydropsychidae, Polycentropodidae and Philopotamidae are laid as a single batch, while the eggs of New Zealand Ecnomidae are likely to be laid in cracks and crevices since the terminal segments of the females form an oviscapt. Spumaline, a protective, jelly-like substance of complex polysaccharides and glycoproteins (Hinton Citation1981), is either absent or forms a thin coating over the egg mass. Some Annulipalpia females have the ability to dive and swim to oviposit on underwater structures due to a modification of the middle and hind legs and the presence of swimming setae (Holzenthal et al. Citation2015).
Prior to the reclassification by Holzenthal et al. (Citation2007), Integripalpia included only caddisflies with case-making larvae. In New Zealand, these include Calocidae, Chathamiidae, Conoesucidae, Helicophidae, Helicopsychidae, Kokiriidae, Leptoceridae, Oeconesidae and Philorheithridae. Eggs are characteristically contained with a spumaline matrix carried internally or extruded and carried externally by the female. Chathamiidae and Kokiriidae are perhaps the exception in that the females have an oviscapt, and one leptocerid species is viviparous (after Caires et al. Citation2016), giving birth to first instar larvae. Females of case-making larvae typically oviposit by entering the water using an emergent structure or release spherical egg masses at the water surface.
In New Zealand, Integripalpia families belonging to the former sub-order Spicipalpia include the species-rich Hydrobiosidae (with predatory free-living larvae) and Hydroptilidae that produce a silken protective case in the final instar. Hydrobiosidae egg masses are typically laid as a single batch with a protective spumaline coating. Nothing is known about the eggs or oviposition of New Zealand Hydroptilidae. Many species of Hydrobiosidae use emergent rocks as a landing pad to enter the water and oviposit underneath rocks (Storey et al. Citation2017).
The following descriptions of caddisfly egg masses, female morphology and egg-laying behaviour are grouped according to families within the sub-orders, Annulipalpia, Integripalpia (excluding Spicipalpia) and ‘Spicipalpia’.
Annulipalpia
Ecnomidae
Two monospecific genera, Ecnomina zelandica Wise, Citation1958 and Zelandoptila moselyi Tillyard, 1924, are known from New Zealand. Both species are characterised by a long slender oviscapt, but nothing is known of their eggs or oviposition behaviour.
An Australian ecnomid, Ecnomus, lays a single egg mass under water primarily on emergent wood (MacQueen and Downes Citation2015). However, unlike most other ecnomid genera, Ecnomus lacks a long oviscapt (Cartwright Citation1990, Citation2010), and therefore this egg-laying behaviour may not be shared by New Zealand ecnomids. It is more likely that New Zealand ecnomids behave like the putatively-related Psychomyiidae (absent from New Zealand) that use their long oviscapts to probe and lay mostly single, non-spumaline coated, eggs into cracks and crevices in submerged wood, the preferred larval habitat (e.g. Spänhoff et al. Citation2003; Spänhoff and Alecke Citation2004).
Both New Zealand ecnomids have fine hair-like sensilla associated with the terminal segment of their oviscapts, but Zelandoptila have additional lateral sensilla along the length of the oviscapt (Smith Citation2014). Terminal sensilla may act as tactile stimuli for egg placement while lateral setae may determine how deeply an egg is inserted in a crevice (Spänhoff et al. Citation2003).
Zelandoptila larvae are typically associated with deeper, cobble- or gravel-dominated stream and river beds with low to moderate abundance of woody debris (NIWA, unpubl. data). The middle leg tibia and tarsi of Zelandoptila are flattened, and both the middle and hind tibia () and tarsi are fringed with short setae (Smith Citation2014). Furthermore, gravid females have been collected flying close to the water and caught on emergent rocks (NIWA, unpubl. data). These features and behaviours suggest females can enter the water either by using an emergent structure or by diving and swimming to a suitable submerged oviposition habitat. Diving and swimming are known among other annulipalpian caddisflies with modified legs, such Hydropsychidae and Polycentropodidae (this review; see Storey et al. Citation2017) as well as some Australian ecnomids (e.g. MacQueen and Downes Citation2015).
Figure 8. Middle leg of female Zelandoptila moselyi (Trichoptera: Ecnomidae) with swimming hairs on the tibia and tarsi.
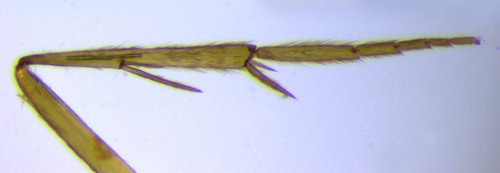
The middle leg tibia of Ecnomina are only weakly flattened and swimming setae are absent from all limbs (Smith Citation2014) indicating females probably do not dive and are not particularly active swimmers. Ecnomina larvae are known from seepages with a large amount of organic debris or slow moving, soft bottomed streams with woody debris comprising the main stable substrate (Maxted et al. Citation2003). Given their preferred larval habitat and an apparent lack of swimming ability, it is suggested that female Ecnomina may either use an emergent structure to crawl into the water as demonstrated for some Psychomyiidae (e.g. Spänhoff et al. Citation2003) or use their oviscapt to deposit single eggs into cracks and crevices in wood just below the water surface.
Hydropsychidae
Among the New Zealand Hydropsychidae, egg masses are known for Hydropsyche (‘Aoteapsyche group’ and ‘Orthopsyche group’) and Diplectrona. Egg masses of these genera are distinctively spumaline-free and rows of closely-packed individual eggs collectively form a flattened semi-fan to thumbnail-shaped disc (), each containing 83–741 eggs (Storey et al. Citation2017; B. J. Smith, unpubl. data). Individual eggs are pale yellow to red-brown depending on their developmental stage and species.
Figure 9. Egg masses of Hydropsyche spp. (Aoteapsyche group) (Trichoptera: Hydropsychidae) showing colour variation.
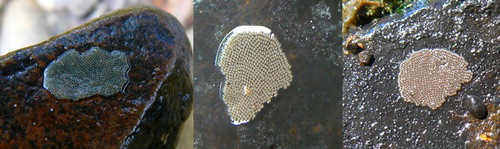
Globally, many Hydropsychidae are capable of diving into the water and swimming to submerged rocks to oviposit (Badcock Citation1952; Deutsch Citation1984, Citation1985). In New Zealand, larvae of Hydropsyche (Aoteapsyche group) inhabit small streams (with emergent rocks present or absent) to large rivers (with emergent rocks few or absent) (McFarlane Citation1976) suggesting females are sometimes required to dive and swim to oviposit. Hydropsyche (Aoteapsyche group) egg masses have been found on both emergent and submerged rocks (Storey et al. Citation2017) and the tibia and tarsi of the female’s middle legs are flattened (McFarlane Citation1976) and fringed with hairs (), indicating enhanced swimming ability. In contrast, legs of Hydropsyche (Orthopsyche group) and Diplectrona are almost rounded in cross-section (Mosely and Kimmins Citation1953; McFarlane Citation1976) and lack swimming hairs, implying that these two taxa may not dive or are poor swimmers. The egg masses of Hydropsyche (Orthopsyche group) have been found laid above the waterline but within a thin film (<3 mm deep) of water flowing over emergent rocks (), while those of Diplectrona have been found on small submerged gravels in a small stream. Hydropsyche (Orthopsyche group) and Diplectrona larvae are mostly found in a mixture of seepages and shallow streams (McFarlane Citation1976; Cowley Citation1978) where swimming or diving is probably unnecessary.
Philopotamidae
Four genera, Cryptobiosella, Hydrobiosella, Neobiosella and Xenobiosella, are recognised within New Zealand (Henderson Citation1983).
Nothing is known about the oviposition behaviour of New Zealand Philopotamidae beyond what can be inferred from female genital morphology, the larval and adult habitats, and from related non-New Zealand taxa. McFarlane (Citation1938) attributed a globular, spumaline egg mass laid a few centimetres out of the water on an over-hanging mossy stone in the quiet reach of a stream to Hydrobiosella stenocerca Tillyard, 1924. However, McFarlane’s observation does not fit within the known parameters of an annulipalpian egg mass or accounts of Australian Hydrobiosella eggs by Reich (Citation2004), and is therefore questionable. The egg mass found by McFarlane (Citation1938) is more representative of an integripalpian caddisfly, perhaps a leptocerid, but interestingly is the only known example of terrestrial oviposition by a New Zealand caddisfly.
Despite the terminal abdominal segments of female New Zealand philopotamids forming an oviscapt of variable length (e.g. Wise Citation1958; Henderson Citation1983) almost all Hydrobiosella eggs are laid on emergent rocks (Reich Citation2004), and not in cracks and crevices. Australian Hydrobiosella egg masses are laid as flattened discs (7–10 mm diameter) of 200–400 closely packed pale pink eggs (0.3–0.4 mm), superficially resembling Hydropsychidae egg masses (Reich Citation2004). Philopotamidae use emergent rocks to enter the water and oviposit (Elliott Citation1981; Reich Citation2004), most likely using these rocks as a landing pad (e.g. Storey et al. Citation2017).
Comparable terminal structures in Hydrobiosella females from Australia and New Zealand (e.g. Mosely and Kimmins Citation1953; Henderson Citation1983; Neboiss Citation1986), as well as similarities noted by Reich (Citation2004) for egg masses and oviposition behaviour of Australian Hydrobiosella and a northern hemisphere philopotamid (Philopotamus montanus [Donovan, 1813]), suggest oviposition behaviour and egg mass appearance are also likely to be similar for New Zealand species. Multiple oviposition events are known for P. montanus but it is uncertain if this behaviour also extends to New Zealand species.
Polycentropodidae
In New Zealand, Polycentropodidae is represented by six species within two genera with global distributions, Polyplectropus and Plectrocnemia (Johanson et al. Citation2012). The egg masses of Polycentropodidae generally resemble the egg mass of Hydropsychidae but with a thin spumaline layer (Reich Citation2004). The egg mass of Polyplectropus altera McFarlane, 1981 (identified by DNA) is flattened and an irregularly outlined oval disc (6.8 × 5.5 mm) containing approximately 500 closely aligned (but not touching) white eggs, laid in semi-neat rows (). This observation is largely consistent with the brief description of a Polyplectropus puerilis (McLachlan, 1868) egg mass by Michaelis (Citation1974) and those of Australian and northern hemisphere Polycentropodidae (e.g. Reich Citation2004; Lancaster et al. Citation2010a).
Oviposition has not been observed for New Zealand Polycentropodidae; however, sexual dimorphism of the middle and hind legs occur in these species and can be used to infer oviposition behaviour. New Zealand species are characterised by flattened tibia and tarsi of the middle legs and a line of fine setae forming a fringe along the margin of the middle and hind legs (B. J. Smith, pers. obs.). In other countries these modifications have been associated with diving and swimming by Polycentropodidae.
In New Zealand up to three species of Polyplectropus adults can be collected together and the width:length ratio of the tibia and tarsi of the middle legs vary among species (B. J. Smith, pers. obs.). Since species with strongly flattened legs are less likely to use emergent rocks for oviposition, and those with weaker flattened legs may oviposit in slower flow or pools (Lancaster et al. Citation2010a), this could suggest oviposition niche partitioning may occur among closely related species (i.e. a species with more flattened legs dive to oviposit on submerged rocks whereas species with moderately or weakly modified legs use emergent rocks to enter the water).
Integripalpia
Calocidae
This family is represented in New Zealand by the sole species, Pycnocentrella eruensis Mosely, 1953. The egg characteristics and oviposition habits of P. eruensis are unknown, but some inferences can be made. The morphology of the female genitalia suggest the egg mass is not extruded and carried externally in flight. However, it is likely to be encapsulated in a spumaline matrix, as this is characteristic of case-making integripalpian caddisflies, and oviposited underwater in the preferred larval habitat of small, shallow forest streams. The middle and hind legs of the female are not flattened and swimming setae are absent, indicating females probably do not dive and are poor swimmers, and are therefore probably using emergent rocks or wood to enter the water.
Chathamiidae
Perhaps the most unusual example of caddisfly oviposition is for the marine caddisfly family Chathamiidae. Five species within two genera are recognised, and females, where known, have a non-flexible, parallel-sided, smoothly sclerotised oviscapt with a pointed tip (Smith Citation2014). The presence of an oviscapt is a globally rare phenomenon within integripalpian caddisflies and known only from Chathamiidae and Kokiria miharo (Kokiriidae).
The oviscapt of Philanisus plebeius Walker, 1852 (), the most common chathamiid, is capable of penetrating the papular pore of the common intertidal cushion star Patiriella regularis (Verrill, 1867) (Asterinidae) and inserting eggs (Winterbourn and Anderson Citation1980). The eggs of P. plebeius are incubated within the coelom of the cushion star (Anderson et al. Citation1976), which may explain the absence of spumaline covering the egg (Leader Citation1976). Development of P. plebeius eggs can exceed 30 days, with first instar larvae leaving the cushion star via either the papular pore or the stomach wall (Winterbourn and Anderson Citation1980).
The middle and hind legs of Chathamiidae are not flattened and swimming setae are absent, suggesting females most likely oviposit in exposed starfish or crawl into rock pools at low tide. Oviposition in starfish may be opportunistic for P. plebeius as females have also been observed inserting their oviscapt into a rock pool (Leader Citation1976) and may also oviposit in crevices at the base of calcareous algae (Riek Citation1976). It remains to be determined whether ovipositing in starfish is obligatory for other species of Chathamiidae (Boast Citation2010).
Conoesucidae
This family, endemic to Australia and New Zealand (Johanson et al. Citation2009), is comprised of six genera and 18 described species in New Zealand (Smith Citation2014) and contains some of the most commonly recognised caddisfly species. The spherical egg mass of a New Zealand Conoesucidae (Olinga feredayi McLachlan, 1868) was first documented by Hudson (Citation1904) and later illustrated (Pycnocentrodes aureolus McLachlan, 1868) by Pendergrast and Cowley (Citation1966). The eggs of both species were later described in detail by Watson (Citation1974). The dark green or green-yellow spherical egg masses have since been observed attached to the terminal abdominal segment of all conoesucid genera except Periwinkla (B. J. Smith, pers. obs.). Because the monospecific Periwinkla childi McFarlane, Citation1973 shares the same genital morphology as all other conoesucids, it can be assumed that it carries a similar spherical egg mass.
Due to the similarity of egg masses among New Zealand Conoesucidae the following account of P. aureolus and O. feredayi are likely to be applicable to other genera. Bluntly elliptical eggs are held within a thin ribbon of spumaline tightly wound into a sphere. The egg mass of P. aureolus may contain 41–233 (mean 105) eggs and O. feredayi 107–314 (mean 222) eggs (Watson Citation1974). Egg mass size is dependent on the size of the female, number of eggs per egg mass and time since oviposition (Watson Citation1974; B. J. Smith, pers. obs.). Egg masses of P. aureolus and O. feredayi are typically 1 mm long when laid but swell to 3.5 and 5 mm, respectively, as the spumaline absorbs water and expands to produce a sticky, transparent, protective outer coating (Watson Citation1974; ). This is considerably greater than the 2.8 mm diameter reported for O. feredayi by Winterbourn (Citation1978); however, these egg masses may have been only recently deposited and may not have swollen to full size.
Figure 14. Spherical egg mass of Olinga feredayi (Trichoptera: Conoesucidae) showing a swollen spumaline outer layer with detritus attached.
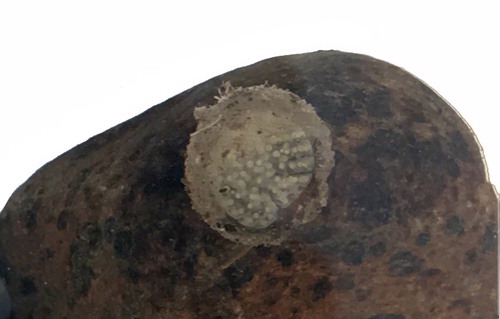
Almost all female Conoesucidae females caught in an ultra violet light trap beside a Waikato pastoral stream were carrying eggs masses attached to the terminal abdominal segments (B. J. Smith, pers. obs.; ). This suggests the egg mass is extruded and carried in flight by the female prior to oviposition. Conoesucidae females have an obliquely truncated terminal abdominal segment and two or three shiny sclerotised plates within the genital cavity that form a smooth cup-like surface for adhesion of the extruded egg mass (McFarlane Citation1966).
Figure 15. Pycnocentria evecta (Trichoptera: Conoesucidae) with spherical egg mass attached, note wings removed.
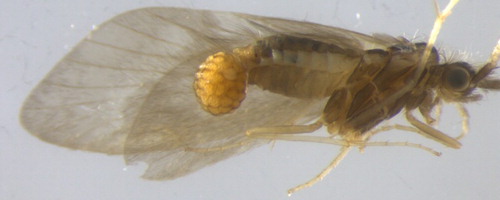
Watson (Citation1974) predicted female Conoesucidae oviposit more than once, and a captured Pycnocentrodes with an external egg mass as well as ovarioles with mature eggs (B. J. Smith, pers. obs.) provides some evidence of multiple oviposition events in this family. In addition, unmated female Olinga are capable of producing egg masses, although the viability of these egg masses has not been tested (B. J. Smith, pers. obs.). It is unknown whether parthenogenesis occurs in other Conoesucidae or New Zealand Trichoptera although Watson (Citation1974) suggested it may explain the strong female bias in some populations.
Watson (Citation1974) recorded egg masses adhered to the upstream facing surface of rocks, but a detailed survey of oviposition habitats revealed almost no conoesucid egg masses attached to rocks despite numerous adults and larvae present (Storey et al. Citation2017). Conoesucidae egg masses have been collected instead from interstitial spaces, trapped within submerged leaf packs or covered in fine detritus on the stream bed in areas of slower flow (B. J. Smith, pers. obs.).
The middle and hind legs of female Conoesucidae are not flattened and swimming setae are absent, suggesting they are unlikely to dive or swim to oviposit egg masses on the undersides of rocks, contrary to Pendergrast and Cowley (Citation1966). Conoesucidae with extruded egg masses have been trapped with sticky Tanglefoot on both emergent rocks and on plastic sheets positioned low above the water surface (NIWA, unpubl. data). This implies eggs are released at the water’s edge or as the female flies over the water surface, supporting Watson’s (Citation1974) suggestion that egg masses are likely to be brushed off the abdomen on contact with water, after which they rapidly absorb water and sink. In some Sericostomatidae, a northern hemisphere equivalent of Conoesucidae, females have been observed releasing a comparable spherical egg mass in-flight (Wood et al. Citation1982), further suggesting that in-flight egg release is likely behaviour among Conoesucidae.
Helicophidae
This small southern hemisphere family is represented in New Zealand by two genera containing six species of day-active adults (Johanson and Ward Citation2002; Henderson and Ward Citation2007). Zelolessica larvae are associated with bryophytes in small to medium-sized streams, and Alloecentrella are usually found in small forested streams and seepages (Johanson and Ward Citation2002; Henderson and Ward Citation2007). Oviposition has not been observed for this family, but it likely takes place during the day when adults are most active.
Abdominal terminal segments form a deep genital cavity in female Helicophidae and contain one to four sclerotised plates (McFarlane Citation1966), which possibly form a receptacle for holding an extruded egg mass prior to oviposition. Helicophidae adults are small (forewing 3–7 mm) and, assuming they are similar to other integripalpian Trichoptera, their egg masses most likely consist of a small batch of eggs in a spumaline matrix.
Helicopsychidae
Two genera of Helicopsychidae, Helicopsyche and Rakiura, are known from New Zealand (McFarlane Citation1973). Helicopsyche has a worldwide distribution and New Zealand has seven endemic species (Johanson Citation1999). The endemic genus Rakiura is represented by a single species, Rakiura vernale McFarlane, Citation1973.
A detailed account of Rakiura life history was provided by Michaelis (Citation1973) including egg characteristics and oviposition behaviour. Rakiura egg masses contain approximately 100 pale orange eggs laid in a ribbon of spumaline in a spiral arrangement (2.2 mm diameter). Since eggs mature during pupation and adults live only eight days and do not feed (Michaelis Citation1973), oviposition is expected to occur shortly after emergence. Adult Rakiura are predominately day-active with copulation and oviposition also occurring during the day (Michaelis Citation1973).
The egg mass of New Zealand Helicopsyche are small and spherical (B. J. Smith, pers. obs.). Females with attached egg masses have been trapped on both sticky emergent rocks and on sticky plastic sheets positioned near the water surface (NIWA, unpubl. data). This shows that the egg mass is extruded and carried prior to oviposition, as for Conoesucidae, and suggests that eggs can be released either at the water’s edge or as the female flies over the water surface.
A female Rakiura was observed swimming down 30 cm to lay an egg mass (Michaelis Citation1973), but since specimens were not available for examination it is not known whether the legs are modified for swimming. The middle and hind legs of Helicopsyche females are not flattened and swimming setae are absent from the New Zealand species examined (Helicopsyche albescens Tillyard, 1924, Helicopsyche poutini McFarlane, Citation1964 and Helicopsyche zealandica Hudson, Citation1904; B. J. Smith, pers. obs.) suggesting females are unlikely to dive or swim to oviposit. Adults have been found aggregated underneath a boulder with egg masses oviposited on a damp leaf pack at the water surface (B. J. Smith, pers. obs.; ).
Kokiriidae
Kokiria miharo McFarlane, Citation1964 is the only representative of this family in New Zealand. Female Kokiria have a wedge-shaped (lateral view), robust oviscapt comprising of three long sclerites (McFarlane Citation1964). No observations of Kokiria eggs or oviposition habitat have been made. If eggs resemble those of other integripalpian caddisflies, they are encased within a protective spumaline matrix. However, the presence of an oviscapt may suggest non-spumaline coated eggs are laid, as noted for Chathamiidae. The unique oviscapt may indicate a yet unknown specialised oviposition habitat or behaviour by Kokiria. Oviposition may occur somewhere in the preferred larval habitat of fine sediment in lake and pond margins or in the backwater pools of streams. It is not known whether the tibia and tarsi of the middle and hind legs of female Kokiria are flattened or if swimming setae are present.
Leptoceridae
The New Zealand Leptoceridae fauna is represented by 10 described species in four genera (Hudsonema, Oecetis, Triplectides and Triplectidina) (Smith Citation2014). Little is known about the egg masses and oviposition behaviour of New Zealand Leptoceridae, but inferences based on personal observation, unpublished data and international literature can add to direct observations.
Extruded spherical egg masses similar to those discussed for Conoesucidae are known for Hudsonema () and Oecetis (NIWA, unpubl. data). These Leptoceridae egg masses are similarly coated with a sticky outer coating of spumaline, thought to help adhere egg masses to rocks or collect fine detritus and sand. This may help camouflage the egg mass or retain the egg in favourable larval habitat. In addition to a sticky spumaline coating, the egg mass of a European lake dwelling Oecetis also has a trailing filament that is thought to anchor the egg to submerged macrophytes (Hanna Citation1961). The presence of a trailing filament has not been confirmed for New Zealand species.
Oviposition by Triplectides obsoletus (McLachlan, 1862) has not been observed in the field but females were observed flying close to stream banks, presumably in search of oviposition habitats (Rowley-Smith Citation1962). In the laboratory, two spumaline covered, irregularly convex egg masses (3 × 4 mm and 4 × 5 mm) were laid by a female, and contained only 7–10 eggs (each egg 0.56 × 0. 71 mm) (Rowley-Smith Citation1962). These egg masses, characteristic of integripalpian caddisflies, were laid at the edge of the water and covered by sand grains and detritus soon after oviposition. It is, however, unclear whether the small, multiple oviposition events observed by Rowley-Smith (Citation1962), and the large number of eggs retained by the female, was an artefact of laboratory conditions.
Oviposition behaviour and the egg masses of T. dolichos McFarlane, 1981 and Triplectidina are unknown, but due to similarities of female terminal structures to T. obsoletus they are also likely to have a similar egg mass.
Oecetis unicolor McLachlan, 1868 and Hudsonema amabile (McLachlan, 1868) have been collected flying close to the water surface with an extruded egg mass attached to the terminal abdominal segments (NIWA, unpubl. data), suggesting egg masses are released over the water or are detached from the abdomen on contact with water. Eggs may also be released at the water’s edge as gravid Hudsonema have been collected from emergent rocks (NIWA, unpubl. data) and Reich (Citation2004) found over 70% of Australian Leptoceridae spherical egg masses were associated with emergent rocks.
Terrestrial oviposition is known for two Australian genera (Lectrides and Leptorussa) (Towns Citation1983) and northern hemisphere Triplectides (Jackson and Sweeney Citation1995) where egg masses are laid above the water. Terrestrial oviposition has not been confirmed for any New Zealand species, but McFarlane (Citation1938) documented a globular trichopteran egg mass a few centimetres out of the water that is more similar to an integripalpian egg mass, perhaps a leptocerid, than the annulipalpian genus he attributed it (see Philopotamidae).
Flattened tibia and tarsi and associated swimming setae are absent from the New Zealand Leptoceridae (B. J. Smith, pers. obs.), suggesting adults do not dive or swim with an extruded egg mass or to fix an egg mass to a submerged structure. Furthermore, underwater oviposition may be hindered by the extremely long antennae characteristic of this family. European Oecetis have been observed repeatedly flying a short distance and resting on the water surface as their egg mass slowly sinks (Hanna Citation1961), and Ceraclea (North American) deposits its egg mass on the water surface, after which the egg mass absorbs water, swells, sinks and adheres to a submerged object (Anderson and Wallace Citation1984).
Perhaps one of the more unusual methods of oviposition in caddisflies is exhibited by Triplectides cephalotes (Walker, 1852) whereby females give birth to first instar larvae (viviparous, as defined by Caires et al. Citation2016; ). According to Lancaster and Downes (Citation2013) viviparity has not been reported for aquatic insects; however, this rare phenomenon has been known for T. cephalotes (Pendergrast and Cowley Citation1966; Michaelis Citation1984) as well as five Australian Triplectides (Morse and Neboiss Citation1982), and more recently reported in some northern hemisphere stoneflies (Caires et al. Citation2016). Triplectides cephalotes can produce up to 600 larvae which are deposited just below the water surface of lakes and ponds (Pendergrast and Cowley Citation1966).
Oeconesidae
Oeconesidae contains five genera, Oeconesus, Pseudoeconesus, Tarapsyche, Zelandopsyche and Zepsyche (Smith Citation2014). The eggs of Zelandopsyche ingens Tillyard, 1921, New Zealand’s second largest caddisfly, are encapsulated in a 13 mm diameter spumaline matrix (), and females can lay on average 370 eggs (0.7 mm diameter) (Winterbourn Citation1978; Greig and McIntosh Citation2008). The eggs of Oeconesus maori McLachlan, 1862 were reported by Anderson (Citation1982) but no further details were given. No observations of the eggs or oviposition behaviour of the remaining Oeconesidae species are known.
Figure 19. Egg mass of Zelandopsyche ingens (Trichoptera: Oeconesidae). Photo courtesy of Angus McIntosh.
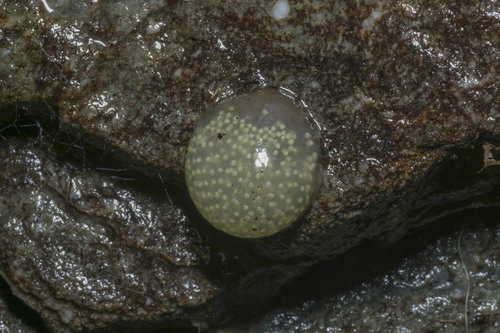
Egg masses of both O. maori and Z. ingens are laid on saturated wood (Winterbourn Citation1978; Anderson Citation1982); however, Z. ingens egg masses have also been seen on emergent rocks (A. McIntosh, University of Canterbury, pers. comm., Nov 2017). Oeconesid larvae are associated with woody debris and leaf litter, and occupy stable seepages and small forest streams with high organic content (Winterbourn and Davis Citation1976). Therefore, there is a high probability that all oeconesid egg masses are laid on emergent structures. Females of Oeconesus, Pseudoeconesus, Tarapsyche and Zelandopsyche lack modified middle or hind legs or swimming setae (B. J. Smith, pers. obs.), suggesting they do not dive or swim. Females of Zepsyche are unknown, but are likely to be similar in this regard.
Philorheithridae
In New Zealand Philorheithridae comprises a single genus, Philorheithrus, with six described species (Henderson and Ward Citation2006). Winterbourn (Citation1978) and Reich (Citation2004) both confirm Philorheithridae egg masses comprise a spherical spumaline matrix of eggs. Egg characteristics are known only for P. agilis (Hudson, Citation1904) (Winterbourn Citation1978) which has an egg mass (4.5–8 mm diameter) containing several hundred eggs (each about 0.3 mm diameter). Given the similarity of the female genitalia among the New Zealand species (Henderson and Ward Citation2006), the egg mass description given by Winterbourn (Citation1978) for P. agilis is likely to be indicative of the other New Zealand species. New Zealand Philorheithrus egg masses appear similar to the Australian genus Aphilorheithrus which has spherical egg masses up to 9 mm diameter containing up to 160 tightly packed eggs (each up to 0.5 mm diameter). Both Winterbourn (Citation1978) and Reich (Citation2004) noted Philorheithridae egg masses were covered in organic and inorganic detritus, and first instar larvae were reported by Reich (Citation2004) to use this detritus to construct a larval case.
Oviposition has not been observed for Philorheithrus but female morphological traits such as shape and configuration of the terminal abdominal segments or the modification of legs for swimming are used to infer oviposition behaviour and egg mass shape. McFarlane (Citation1966) noted the sub-terminal abdominal segments of female Philorheithrus resemble a shiny ‘strongly sclerotised pit’ ideal for holding the spherical egg mass prior to oviposition. Additionally, the genital opening does not directly open into the egg retention pit and suggests the egg mass may be transferred into the pit once eggs have been extruded (B. J. Smith, pers. obs.), supporting McFarlane’s observation that the egg mass is carried externally prior to oviposition. The middle legs of Philorheithrus are not flattened or modified for swimming (B. J. Smith, pers. obs.), and although Hudson (Citation1904) reported P. agilis diving into the water to escape predation, it is uncertain if this behaviour also extends to oviposition. Philorheithrus agilis egg masses have been found attached to the undersides of rocks and logs (Winterbourn Citation1978; Anderson Citation1982).
‘Spicipalpia’
Hydrobiosidae
Hydrobiosidae is by far New Zealand’s most species-rich family, with well over 100 species in 11 genera (Smith Citation2014), and their egg masses are the most common and recognisable among the New Zealand trichopterans.
New Zealand Hydrobiosidae egg masses are typically round to oval discs 3–17 mm wide, 3–32 mm long and covered with a thin (<2 mm) spumaline coating (Storey et al. Citation2017; A). Spumaline can be up to 5 mm thick in Australian Hydrobiosidae, providing protection against specialist egg predators (Reich Citation2004; Bovill et al. Citation2015), and the thinner layer associated with New Zealand species may be attributable to a perceived absence of native egg predators (Smith and Reid Citation2016). Hydrobiosidae eggs are usually white and laid in a straight or slightly curved row within a thin spumaline matrix, and contain 100–1200 eggs (Storey et al. Citation2017). Up to two egg masses may be laid by Psilochorema mimicum (McLachlan, 1866) (B. J. Smith, unpubl. data) and Hydrobiosis frater (McLachlan, 1868) (McFarlane Citation1938) indicating multiple oviposition events can occur.
Figure 20. Hydrobiosidae (Trichoptera) egg masses. A, Egg mass aggregation; B, Hydrobiosis umbripennis; C, Hydrobiosis soror; D, Psilochorema sp.
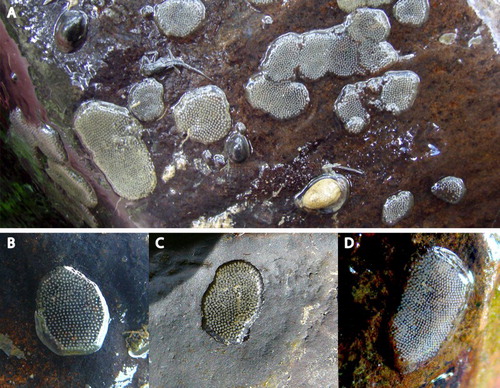
Hydrobiosidae egg masses look very similar to one another, but spacing between individual eggs and the shape of the egg mass may vary between species. Using DNA-based identifications (Storey et al. Citation2017), it is known that Hydrobiosis egg masses are generally round and eggs are laid in neat rows with individual eggs spaced at least one egg-diameter apart (B), though H. soror eggs are more tightly arranged in a semi-random order (C). Psilochorema egg masses are more elongate to rectangular with white eggs laid in neat, gently curving rows (D); Costachorema egg masses are similar to Psilochorema, but individual eggs are yellow-green to brown, although this may be dependent on the developmental stage of the egg (Storey et al. Citation2017; B. J. Smith, unpubl. data).
The middle and hind leg tibia and tarsi of Hydrobiosidae females are not flattened, implying they are unable to swim to oviposit on submerged rocks. Instead, females probably enter the water by crawling down an emergent rock and ovipositing underneath (Storey et al. Citation2017). The genitalia of female Hydrobiosidae are generally truncate, except for Tiphobiosis, in which the terminal abdominal segments form a long, curved, dorso-ventrally flattened oviscapt (). This flattened oviscapt may be beneficial for laying small eggs in cracks of rock seepages where Tiphobiosis larvae are most commonly found (Collier and Smith Citation2006). Tiphobiosis eggs are likely to be laid singly, as seen in other trichopteran taxa with a long oviscapt (e.g. Spänhoff and Alecke Citation2004).
Hydroptilidae
New Zealand Hydroptilidae comprises Oxyethira, Paroxyethira and Xuthotrichia, whose larvae are associated with streams, lakes and ponds, and seepages (Ward and Henderson Citation2004). New Zealand Hydroptilidae were reported by Leader (Citation1970) to enter the water to oviposit, but no further details were given. Hydroptilidae eggs have been described for two Australian genera associated with lotic habitats (Reich Citation2004). In both Australian genera small, pale pink eggs are tightly packed and laid in small concentric rows. Egg masses are up to 6 mm diameter and laid as flattened spumaline-free discs below the water surface (Reich Citation2004).
Reich (Citation2004) found egg masses for the two Australian genera strongly associated with emergent rocks, but in New Zealand only Oxyethira albiceps McLachlan, 1862 and Paroxyethira hintoni Leader, Citation1972 may show similar preferences due to their affiliation with running water (e.g. Leader Citation1972; Cowley Citation1978). The abdominal segments of female Xuthotrichia and at least two species of Oxyethira, and many Paroxyethira (Leader Citation1972; Wise Citation1998; Ward and Henderson Citation2004), terminate as an oviscapt-like structure suggesting a range of yet unknown oviposition habitat and behaviours. Larvae of Oxyethira ahipara Wise, Citation1998, as well as adults of Oxyethira kirikiriroa Smith, 2008 and many of the Paroxyethira adults described by Ward and Henderson (Citation2004), have been collected close to seepages, suggesting seepages may be the preferred oviposition and larval habitat for these taxa. Tibia and tarsi of the female’s middle and hind legs are not flattened; however, both male and female are covered in long setae (B. J. Smith, pers. obs.): whether these setae are used for swimming is yet to be determined.
Conclusion
Population dynamics that determine the abundance and distribution of aquatic insects may be controlled by factors at any stage of the life cycle. In order to understand the potential role of factors at egg and adult stages, and the process of oviposition that connects them, basic information on egg characteristics and oviposition behaviour is required. This review compiles available information on egg morphology and oviposition behaviour for the three aquatic insect orders Ephemeroptera, Plecoptera and Trichoptera in New Zealand. It also highlights the many knowledge gaps, in the hope of stimulating further research.
Some families in these orders show a variety of different egg characteristics and oviposition behaviours, but there are also strong commonalities that characterise families and sub-orders. We have used these commonalities to infer egg characteristics and oviposition behaviour for some taxa where no direct observations are known. These inferences provide ‘best guesses’ to temporarily fill knowledge gaps, but we hope they encourage researchers to publish direct observations.
Eggs show a variety of different sizes, shapes, coatings, stickiness and attachment structures. How these characteristics influence egg retention, transport and distribution is largely unknown, but could be explored with the use of hydraulic models. This knowledge would then open opportunities to discover the consequences of egg retention and transport for insect dispersal and colonisation dynamics. Eggs also show great variation in terms of time till hatching and whether they require adult feeding to produce and or mature, and these aspects carry implications for egg survivorship under different aquatic and terrestrial conditions.
Highly selective oviposition behaviour has been observed for some insect taxa, and for others specialised oviposition is suggested by female morphology. In contrast, many taxa appear to show generalist behaviour, for example releasing their eggs on the water surface. We would expect the more selective taxa to be more strongly affected by the presence or absence of particular habitat features in streams. However, those that release eggs on the water surface may still be responding to specific oviposition cues, such as polarised light reflected from the water surface, flow characteristics, certain chemical cues or specific temperatures (Lancaster and Downes Citation2013). Therefore, they may also be affected by human modifications to streams, such as increases in suspended sediment which reduce the polarisation of reflected light (Kriska et al. Citation2009). More research is needed to understand these cues and whether alterations by human activity may disrupt insect oviposition.
Globally, billions of dollars are invested each year in stream restoration (more than $1 billion dollars in the US alone; Bernhardt et al. Citation2005). Yet aquatic insects rarely succeed in recolonising restored reaches, and the reasons for this failure are poorly understood (Feld et al. Citation2011). As evidence points to the potential importance of egg and adult life stages, and the processes linking them with larval populations, understanding the role of eggs and oviposition behaviour in insect population dynamics is increasingly important for improving the success of stream restoration.
Acknowledgements
The authors wish to thank the NIWA Library Service (Greta Point, Wellington, New Zealand) for national and international loans, as well as Bryce McQuillan (Bryce McQuillan Photography) and Angus McIntosh (University of Canterbury, Christchurch, New Zealand) for kindly supplying Figures 1 and 19, respectively. We gratefully acknowledge the valuable comments and suggestions by reviewers Ralph Holzenthal, J. Manuel Tierno de Figueroa and an anonymous reviewer.
Disclosure statement
No potential conflict of interest was reported by the authors.
Additional information
Funding
References
- Anderson DT, Fletcher MJ, Lawson-Kerr C. 1976. A marine caddis fly, Philanisus plebeius, ovipositing in a starfish, Patiriella exigua. Search. 7:483–484.
- Anderson NH. 1982. A survey of aquatic insects associated with wood debris in New Zealand streams. Mauri Ora. 10:21–33.
- Anderson NH, Wallace JB. 1984. Habitat, life history, and behavioral adaptations of aquatic insects. In: Merritt RW, Cummins KW, editors. An introduction to the aquatic insects of North America. 2nd ed. Dubuque, IA: Kendall/Hunt Publishing Company; p. 38–58.
- Badcock RM. 1952. Observation of egg-laying under water of the aerial insect Hydropsyche angustipennies (Curtis) (Trichoptera). Nature. 170:40–41. doi: 10.1038/170040b0
- Beer-Stiller A, Zwick P. 1995. Biometric studies of some stoneflies and a mayfly (Plecoptera and Ephemeroptera). Hydrobiologia. 299:169–178. doi: 10.1007/BF00017568
- Bernhardt ES, Palmer MA, Allan JD, Alexander G, Barnas K, Brooks S, Carr J, Clayton S, Dahm C, Follstad-Shah J, et al. 2005. Synthesizing US river restoration efforts. Science. 308:636–637. doi: 10.1126/science.1109769
- Blakely TJ, Harding JS, McIntosh AR, Winterbourn MJ. 2006. Barriers to the recovery of aquatic insect communities in urban streams. Freshwater Biology. 51:1634–1645. doi: 10.1111/j.1365-2427.2006.01601.x
- Boast AP. 2010. Evolution of New Zealand’s marine caddisflies: a phylogenetic and phylogeographic assessment of the Chathamiidae (Insecta: Trichoptera). Wellington: Victoria University, New Zealand.
- Bovill WD, Downes BJ, Lancaster J. 2015. Caddisfly egg mass morphology mediates egg predation: potential costs to individuals and populations. Freshwater Biology. 60:360–372. doi: 10.1111/fwb.12497
- Brittain JE. 1982. Biology of mayflies. Annual Review of Entomology. 27:119–147. doi: 10.1146/annurev.en.27.010182.001003
- Caires AM, Chandra S, Nelson CR. 2016. Unique reproductive characteristics of Lake Tahoe’s Capnia lacustra (Plecoptera: Capniidae), a stonefly in decline. Freshwater Science. 35:1291–1299. doi: 10.1086/688485
- Cartwright DI. 1990. The Australian species of Ecnomus McLachlan (Trichoptera: Ecnomidae). Memoirs of the Museum of Victoria. 51:1–48. doi: 10.24199/j.mmv.1990.51.01
- Cartwright DI. 2010. Descriptions of two new genera and 13 new species of caddisflies from Australia. (Trichoptera: Ecnomidae). Zootaxa. 2415:1–21.
- Chisholm WP. 1984. Aspects of the biology of Ichthybotus hudsoni (McLachlan) (Ephemeroptera: Ephemeridae). Wellington (New Zealand): Victoria University.
- Collier KJ, Smith BJ. 2003. Role of wood in pumice-bed streams II. Breakdown and colonisation. Forest Ecology and Management. 177:261–276. doi: 10.1016/S0378-1127(02)00451-6
- Collier KJ, Smith BJ. 2006. Distinctive invertebrate assemblages in rockface seepages enhance lotic biodiversity in northern New Zealand. Biodiversity and Conservation. 15:3591–3616. doi: 10.1007/s10531-005-5395-8
- Cowley DR. 1978. Studies on the larvae of New Zealand Trichoptera. New Zealand Journal of Zoology. 5:639–750. doi: 10.1080/03014223.1978.10423816
- Death RG. 1990. A new species of Zelandobius (Plecoptera: Gripopterygidae: Antarctoperlinae) from New Zealand. New Zealand Natural Sciences. 17:23–28.
- Deutsch WG. 1984. Oviposition of Hydropsychidae (Trichoptera) in a large river. Canadian Journal of Zoology. 62:1988–1994. doi: 10.1139/z84-291
- Deutsch WG. 1985. Swimming modifications of adult female Hydropsychidae compared with other Trichoptera. Freshwater Invertebrate Biology. 4:35–40. doi: 10.2307/1467197
- Elliott JM. 1981. A quantitative study of the life cycle of the net-spinning caddis Philopotamus montanus (Trichoptera: Philopotamidae) in a Lake District stream. The Journal of Animal Ecology. 50:867–883. doi: 10.2307/4143
- Encalada AC, Peckarsky BL. 2007. A comparative study of the costs of alternative mayfly oviposition behaviors. Behavioral Ecology and Sociobiology. 61:1437–1448. doi: 10.1007/s00265-007-0376-4
- Feld CK, Birk S, Bradley DC, Hering D, Kail J, Marzin A, Friberg N. 2011. From natural to degraded rivers and back again: a test of restoration ecology theory and practice. Advances in Ecological Research. 44:119–209. doi: 10.1016/B978-0-12-374794-5.00003-1
- Frania HE, Wiggins GB. 1997. Analysis of morphological and behavioural evidence for the phylogeny and higher classification of Trichoptera (Insecta). Life Science Contributions of the Royal Ontario Museum. 160:1–67.
- Gaino E, Piersanti S, Rebora M. 2009. The oviposition mechanism in Habrophlebia eldae (Ephemeroptera: Leptophlebiidae). Aquatic Insects: International Journal of Freshwater Entomology. 31:515–522. doi: 10.1080/01650420902732396
- Giling DP, Mac Nally R, Thompson RM. 2016. How sensitive are invertebrates to riparian-zone replanting in stream ecosystems? Marine and Freshwater Research. 67:1500–1511. doi: 10.1071/MF14360
- Greig HS, McIntosh AR. 2008. Density reductions by predatory trout increase adult size and fecundity of surviving caddisfly larvae in a detritus-based stream food web. Freshwater Biology. 53:1579–1591. doi: 10.1111/j.1365-2427.2008.01995.x
- Hanna HM. 1961. Observations on the egg-laying of some British caddis flies and on case-building by newly hatched larvae. Proceedings of the Royal Entomological Society of London. Series A, General Entomology. 36:57–62. doi: 10.1111/j.1365-3032.1961.tb00267.x
- Heino J, Peckarsky BL. 2014. Integrating behavioral, population and large-scale approaches for understanding stream insect communities. Current Opinion in Insect Science. 2:7–13. doi: 10.1016/j.cois.2014.06.002
- Helson GAH. 1934. The bionomics and anatomy of Stenoperla prasina. Transactions and Proceedings of the Royal Society of New Zealand. 64:214–248.
- Helson GAH. 1935. The hatching and early instars of Stenoperla prasina Newman. Transactions and Proceedings of the Royal Society of New Zealand. 65:11–14.
- Henderson IM. 1983. A contribution to the systematics of New Zealand Philopotamidae (Trichoptera). New Zealand Journal of Zoology. 10:163–176. doi: 10.1080/03014223.1983.10423905
- Henderson IM, Ward JB. 2006. Four new species of the caddis genus Philorheithrus from New Zealand. Records of the Canterbury Museum. 20:21–33.
- Henderson IM, Ward JB. 2007. Three new species in the endemic New Zealand genus Alloecentrella (Trichoptera), and a re-evaluation of its family placement. Journal of Aquatic Insects: International Journal of Freshwater Entomology. 29:79–96. doi: 10.1080/01650420701381155
- Hinton HE. 1981. Biology of insect eggs 1–3. Oxford (UK): Pergamon Press.
- Hitchings TR, Hitchings TR. 2016. Two further species of Deleatidium (Deleatidium) (Ephemeroptera: Leptophlebiidae) from New Zealand. Records of the Canterbury Museum. 30:54–64.
- Hitchings TR, Hitchings TR, Shaw MD. 2015. A revision of the distribution maps and database of New Zealand mayflies (Ephemeroptera) at Canterbury Museum. Records of the Canterbury Museum. 29:5–34.
- Hitchings TR, Staniczek AH. 2003. Nesameletidae (Insecta: Ephemeroptera). Fauna of New Zealand. 46:72.
- Holzenthal RW, Blahnik RJ, Prather AL, Kjer KM. 2007. Order Trichoptera Kirby, 1813 (Insecta), caddisflies. Zootaxa. 1668:639–698.
- Holzenthal RW, Thomson RE, Ríos-Touma B. 2015. Order Trichoptera. Ecology and General Biology. 1:965–1002.
- Hudson GV. 1904. New Zealand Neuroptera. A popular introduction to the life-histories and habits of may-flies, dragon-flies, caddis- flies and allied insects inhabiting New Zealand. Including notes on their relation to angling. London: West, Newman and Co.
- Hynes HBN. 1974. Observations on the adults and eggs of Australian Plecoptera. Australian Journal of Zoology, Supplementary Series. 29:37–52.
- Hynes HBN. 1976. Biology of Plecoptera. Annual Review of Entomology. 21:135–153. doi: 10.1146/annurev.en.21.010176.001031
- Hynes HBN, Hynes ME. 1975. The life histories of many of the stoneflies of south-eastern mainland Australia. Australian Journal of Marine and Freshwater Research. 26:3–53. doi: 10.1071/MF9750113
- Illies J. 1963. The Plecoptera of the Auckland and Campbell Islands. New Zealand Journal of Zoology. 4:255–265.
- Jackson JK, Sweeney BW. 1995. Egg and larval development times for 35 species of tropical stream insects from Costa Rica. Journal of the North American Benthological Society. 14:115–130. doi: 10.2307/1467728
- Johanson KA. 1999. Revision of the New Zealand Helicopsyche (Trichoptera: Helicopsychidae). Insect Systematics & Evolution. 30:263–280. doi: 10.1163/187631200X00110
- Johanson KA, Kjer K, Malm T. 2009. Testing the monophyly of the New Zealand and Australian endemic family Conoesucidae Ross based on combined molecular and morphological data (Insecta: Trichoptera: Sericostomatoidea). Zoologica Scripta. 38:563–573. doi: 10.1111/j.1463-6409.2009.00391.x
- Johanson KA, Malm T, Espeland M, Weingartner E. 2012. Phylogeny of the Polycentropodidae (Insecta: Trichoptera) based on protein-coding genes reveal non-monophyletic genera. Molecular Phylogenetics and Evolution. 65:126–135. doi: 10.1016/j.ympev.2012.05.029
- Johanson KA, Ward JB. 2002. Re-description of the genus Zelolessica and of its two species (Insecta, Trichoptera, Helicophidae). Records of the Canterbury Museum. 16:1–17.
- Koss RW, Edmunds GF. 1974. Ephemeroptera eggs and their contribution to phylogenetic studies of the order. Zoological Journal of the Linnean Society. 55:267–349. doi: 10.1111/j.1096-3642.1974.tb01648.x
- Kriska G, Bernath B, Farkas R, Horvath G. 2009. Degrees of polarization of reflected light eliciting polarotaxis in dragonflies (Odonata), mayflies (Ephemeroptera) and tabanid flies (Tabanidae). Journal of Insect Physiology. 55:1167–1173. doi: 10.1016/j.jinsphys.2009.08.013
- Lancaster J, Downes BJ. 2013. Aquatic entomology. Oxford (UK): Oxford University Press.
- Lancaster J, Downes BJ, Arnold A. 2010a. Oviposition site selectivity of some stream-dwelling caddisflies. Hydrobiologia. 652:165–178. doi: 10.1007/s10750-010-0328-2
- Lancaster J, Downes BJ, Arnold A. 2010b. Environmental constraints on oviposition limit egg supply of a stream insect at multiple scales. Oecologia. 163:373–384. doi: 10.1007/s00442-010-1565-9
- Leader JP. 1970. Hairs of the Hydroptilidae (Trichoptera). Tane. 16:121–130.
- Leader JP. 1972. The New Zealand Hydroptilidae (Trichoptera). Journal of Entomology Series B, Taxonomy. 41:191–200. doi: 10.1111/j.1365-3113.1972.tb00047.x
- Leader JP. 1976. Marine caddisflies (Trichoptera: Philanisidae). In: Cheng L, editor. Marine insects. Amsterdam: North Holland Publishing Co; p. 291–302.
- Louhi P, Mykrä H, Paavola R, Huusko A, Vehanen T, Mäki-Petäys A, Muotka T. 2011. Twenty years of stream restoration in Finland: little response by benthic macroinvertebrate communities. Ecological Applications. 21:1950–1961. doi: 10.1890/10-0591.1
- Macneale KH, Likens GE, Peckarsky BL. 2002. Feeding strategy of an adult stonefly (Plecoptera): implications for egg production and dispersal. Verhandlungen der Internationalen Vereinigung für Theoretische und Angewandte Limnologie. 28:1140–1146.
- Macqueen A, Downes BJ. 2015. Large-scale manipulation of oviposition substrata affects egg supply to populations of some stream-dwelling caddisflies. Freshwater Biology. 60:802–812. doi: 10.1111/fwb.12535
- Maxted JR, Evans BF, Scarsbrook MR. 2003. Development of standard protocols for macroinvertebrate assessment of soft-bottomed streams in New Zealand. New Zealand Journal of Marine Freshwater Research. 37:793–807. doi: 10.1080/00288330.2003.9517209
- McFarlane AG. 1938. Life histories and biology of New Zealand Rhyacophilidae (order Trichoptera). Christchurch (New Zealand): University of Canterbury.
- McFarlane AG. 1964. A new endemic subfamily, and other additions and emendations to the Trichoptera of New Zealand (Part 5). Records of the Canterbury Museum. 8:55–79.
- McFarlane AG. 1966. New Zealand Trichoptera (Part 6). Records of the Canterbury Museum. 8:137–161.
- McFarlane AG. 1973. Five new species of Trichoptera from New Zealand. Journal of Royal Society of New Zealand. 3:23–34. doi: 10.1080/03036758.1973.10416100
- McFarlane AG. 1976. A generic revision of New Zealand Hydropsychinae (Trichoptera). Journal of the Royal Society of New Zealand. 6:23–35. doi: 10.1080/03036758.1976.10421463
- McLean JA. 1967. Studies of Ephemeroptera in the Auckland area. Tane. 13:99–105.
- McLean JA. 1970. Studies on the larvae of Oniscigaster wakefieldi (Ephemeroptera: Siphlonuridae) in Waitakere Stream, Auckland. New Zealand Journal of Marine and Freshwater Research. 4:36–45. doi: 10.1080/00288330.1970.9515325
- McLellan ID. 1967. New Gripopterygids (Plecoptera) of New Zealand. Transactions of the Royal Society of New Zealand—Zoology. 9:1–15.
- McLellan ID. 1975. The freshwater insects. In: Kuschel G, editor. Biogeography and ecology in New Zealand. The Hague: Dr W. Junk Publishers; p. 537–559.
- McLellan ID. 1977. New alpine and southern Plecoptera from New Zealand, and a new classification of the Gripopterygidae. New Zealand Journal of Zoology. 4:119–147. doi: 10.1080/03014223.1977.9517945
- McLellan ID. 1991. Notonemouridae (Insecta; Plecoptera). Fauna of New Zealand. 22:64.
- McLellan ID. 1996. A revision of Stenoperla (Plecoptera: Eustheniidae) and removal of Australian species to Cosmioperla new genus. New Zealand Journal of Zoology. 23:165–182. doi: 10.1080/03014223.1996.9518076
- McLellan ID. 1997. Austroperla cyrene Newman (Plecoptera: Austroperlidae). Journal of the Royal Society of New Zealand. 27:271–278. doi: 10.1080/03014223.1997.9517538
- McLellan ID. 1998. A revision of Acroperla (Plecoptera: Zelandoperlinae) and removal of species to Taraperla new genus. New Zealand Journal of Zoology. 25:185–203. doi: 10.1080/03014223.1998.9518149
- McLellan ID. 2000. A revision of Cristaperla (Plecoptera: Notonemouridae) and some comments on Notonemouridae and its generic groups. New Zealand Journal of Zoology. 27:233–244. doi: 10.1080/03014223.2000.9518231
- McLellan ID. 2003. Six new species and a new genus of stoneflies (Plecoptera) from New Zealand. New Zealand Journal of Zoology. 30:101–113. doi: 10.1080/03014223.2003.9518329
- McLellan ID. 2006. Endemism and biogeography of New Zealand Plecoptera (Insecta). Illiesia. 2:15–23.
- Michaelis FB. 1973. The distribution and life history of Rakiura vernale (Trichoptera: Helicopsychidae). Journal of the Royal Society of New Zealand. 3:295–304. doi: 10.1080/03036758.1973.10430607
- Michaelis FB. 1974. The ecology of Waikoropupu springs. Christchurch (New Zealand): University of Canterbury.
- Michaelis FB. 1984. The life history of Megaleptoperla diminuta (Plecoptera: Gripopterygidae) in Waikoropupu Springs, New Zealand. Annales de Limnologie. 20:69–74. doi: 10.1051/limn/1984023
- Morse JC, Neboiss A. 1982. Triplectides of Australia (Insecta: Trichoptera: Leptoceridae). Memoirs of the National Museum of Victoria. 43:61–98. doi: 10.24199/j.mmv.1982.43.05
- Mosely ME, Kimmins DE. 1953. The Trichoptera (caddis-flies) of Australia and New Zealand. London: British Museum of Natural History.
- Neboiss A. 1986. Atlas of Trichoptera of the SW Pacific-Australian region. Series Entomologica 37. Dordrecht: Dr W. Junk Publishers.
- Norrie PH. 1969. The flight activity of Ephemeroptera and Trichoptera in Waitakere stream. Auckland (New Zealand): University of Auckland.
- Oberndorfer RY, Stewart KW. 1977. The life cycle of Hydroperla crosbyi (Plecoptera: Perlodidae). The Great Basin Naturalist. 37:260–273.
- Palmer MA, Menninger HL, Bernhard E. 2010. River restoration, habitat heterogeneity and biodiversity: a failure of theory or practice? Freshwater Biology. 55:205–222. doi: 10.1111/j.1365-2427.2009.02372.x
- Parkyn SM, Davies-Colley RJ, Halliday NJ, Costley KJ, Croker GF. 2003. Planted riparian buffer zones in New Zealand: do they live up to expectations? Restoration Ecology. 11:436–447. doi: 10.1046/j.1526-100X.2003.rec0260.x
- Peckarsky BL, Taylor BW, Caudill CC. 2000. Hydrologic and behavioral constraints on oviposition of stream insects: implications for adult dispersal. Oecologia. 125:186–200. doi: 10.1007/s004420000446
- Pendergrast JG, Cowley DR. 1966. An introduction to New Zealand freshwater insects. Auckland: Collins.
- Peters WL, Campbell IC. 1991. Ephemeroptera (mayflies). In: CSIRO Division of Entomology, editor. The insects of Australia vol. 1. 2nd ed. Carlton, VIC: Melbourne University Press; p. 279–293.
- Phillips JS. 1930a. A revision of New Zealand Ephemeroptera. Part 1. Transactions of the New Zealand Institute. 61:271–334.
- Phillips JS. 1930b. A revision of New Zealand Ephemeroptera. Part 2. Transactions of the New Zealand Institute. 61:335–390.
- Purcell AH, Friedrich C, Resh VH. 2002. An assessment of a small urban stream restoration project in northern California. Restoration Ecology. 10:685–694. doi: 10.1046/j.1526-100X.2002.01049.x
- Rait L. 1937. Some observations on the immature stages of Eusthenia spectabilis (Westwood). Transactions of the Royal Society of Australia. 61:74–79.
- Reich P. 2004. Patterns of composition and abundance in macroinvertebrate egg masses from temperate Australian streams. Marine and Freshwater Research. 55:39–56. doi: 10.1071/MF03129
- Reich P, Downes BJ. 2003a. Experimental evidence for physical cues involved in oviposition site selection of lotic hydrobiosid caddis flies. Oecologia. 136:465–475. doi: 10.1007/s00442-003-1284-6
- Reich P, Downes BJ. 2003b. The distribution of aquatic invertebrate egg masses in relation to physical characteristics of oviposition sites at two Victorian upland streams. Freshwater Biology. 48:1497–1513. doi: 10.1046/j.1365-2427.2003.01101.x
- Riek EF. 1970. Ephemeroptera (mayflies). In: CSIRO, editor. The insects of Australia. Melbourne: Melbourne University Press; p. 224–240.
- Riek EF. 1976. The marine caddisfly family Chathamiidae (Trichoptera). Journal of the Australian Entomological Society. 15:405–419. doi: 10.1111/j.1440-6055.1976.tb01724.x
- Rowley-Smith DM. 1962. Studies on the biology and functional morphology of Triplectides obsoleta. Canterbury, New Zealand: University of Canterbury.
- Smith BJ. 2014. Trichoptera: a guide to the adult caddisflies from New Zealand. NIWA Information Series, No. 83. Hamilton: National Institute of water and Atmospheric Research Ltd.
- Smith BJ, Collier KJ. 2000. Interactions of adult stoneflies (Plecoptera) with riparian zones II. Diet. Aquatic Insects: International Journal of Freshwater Entomology. 22:285–296. doi: 10.1076/0165-0424(200010)22:4;1-Y;FT285
- Smith BJ, Reid DJ. 2016. Silent assassins: predation of native New Zealand trichopteran eggs by non-native freshwater gastropods. Aquatic Insects: International Journal of Freshwater Entomology. 37:293–302. doi: 10.1080/01650424.2016.1274767
- Spänhoff B, Alecke C. 2004. Ecological aspects of the external morphology and functionality of the psychomyiid female ovipositor (Insecta, Trichoptera). Zoomorphology. 123:213–220. doi: 10.1007/s00435-004-0104-9
- Spänhoff B, Alecke C, Kaschek N, Lange J, Meyer EI. 2003. Morphological characteristics of sensilla on the female ovipositor of Lype phaeopa (Psychomyiidae; Trichoptera). Journal of Insect Science. 3:12. insectscience.org/3.12. doi: 10.1093/jis/3.1.12
- Staniczek AH, Hitchings TR. 2013. A new species of Rallidens (Ephemeroptera: Rallidentidae) from New Zealand. Records of the Canterbury Museum. 27:1–9.
- Stark BP, Froehlich C, Zúñiga MC. 2009. South American stoneflies (Plecoptera). In: Adis J, Arias JR, Rueda-Delgado G, Wantzen KM, editors. Aquatic biodiversity in Latin America (ABLA), Vol. 5. Sofia: Pensoft. 154 pp.
- Storey RG, Reid DR, Smith BJ. 2017. Oviposition site selectivity of some New Zealand aquatic macroinvertebrate taxa and implications for stream restoration. New Zealand Journal of Marine and Freshwater Research. 51:165–181. doi: 10.1080/00288330.2016.1269351
- Stranko SA, Hilderbrand RH, Palmer MA. 2012. Comparing the fish and benthic macroinvertebrate diversity of restored urban streams to reference streams. Restoration Ecology. 20:747–755. doi: 10.1111/j.1526-100X.2011.00824.x
- Thomson MS. 1934. An account of the systematics, anatomy and bionomics of Austroperla cyrene Newman. Christchurch (New Zealand): University of Canterbury.
- Towns DR. 1983. Terrestrial oviposition by two species of caddisfly in South Australia (Trichoptera: Leptoceridae). Australian Journal of Entomology. 22:113–118. doi: 10.1111/j.1440-6055.1983.tb01854.x
- Towns DR, Peters WL. 1996. Leptophlebiidae (Insecta: Ephemeroptera). Fauna of New Zealand. 36:144.
- Ward JB, Henderson IM. 2004. Eleven new species of micro-caddisflies (Trichoptera: Hydroptilidae) from New Zealand. Records of the Canterbury Museum. 18:9–22.
- Watson GW. 1974. Aspects of the biology of two Sericostomatidae (Trichoptera) from the Waitakere stream. Auckland (New Zealand): University of Auckland.
- Winterbourn MJ. 1966. Studies on New Zealand stoneflies. 2. The ecology of Zelandoperla maculata (Hare) and Aucklandobius trivacuatus (Tillyard)-(Gripopterygidae). New Zealand Journal of Science. 9:312–323.
- Winterbourn MJ. 1978. The macroinvertebrate fauna of a New Zealand forest stream. New Zealand Journal of Zoology. 5:157–169. doi: 10.1080/03014223.1978.10423746
- Winterbourn MJ. 2003. Habitat segregation and nymphal life history of two Nesameletus species (Ephemeroptera: Nesameletidae) in a mountain stream. Aquatic Insects: International Journal of Freshwater Entomology. 25:41–50. doi: 10.1076/aqin.25.1.41.14028
- Winterbourn MJ. 2005. Dispersal, feeding and parasitism of adult stoneflies (Plecoptera) at a New Zealand forest stream. Aquatic Insects: International Journal of Freshwater Entomology. 27:155–166. doi: 10.1080/01650420500062840
- Winterbourn MJ. 2010. Life histories of two stoneflies (Plecoptera: Gripopterygidae) in two streams on the West Coast, New Zealand. New Zealand Natural Sciences. 35:1–8.
- Winterbourn MJ, Anderson NH. 1980. The life history of Philanisus plebeius Walker (Trichoptera: Chathamiidae), a caddisfly whose eggs were found in a starfish. Ecological Entomology. 5:293–304. doi: 10.1111/j.1365-2311.1980.tb01151.x
- Winterbourn MJ, Davis SF. 1976. Ecological role of Zelandopsyche ingens (Trichoptera: Oeconesidae) in a beech forest stream ecosystem. Australian Journal of Marine and Freshwater Research. 27:197–215. doi: 10.1071/MF9760197
- Wise KAJ. 1958. Trichoptera of New Zealand. I. A catalogue of the Auckland Museum collection with descriptions of new genera and new species. Records of the Auckland Institute and Museum. 5:49–63.
- Wise KAJ. 1998. Two new species of Oxyethira (Trichoptera: Hydroptilidae) in New Zealand. New Zealand Entomologist. 21:17–23. doi: 10.1080/00779962.1998.9722036
- Wisely B. 1953. Two wingless alpine stoneflies (Order Plecoptera) from southern New Zealand. Records of the Canterbury Museum. 6:219–231.
- Wisely HB. 1961. Studies on Ephemeroptera 1. Coloburiscus humeralis (Walker); early life history and nymph. Transactions of the Royal Society of New Zealand—Zoology. 1:249–257.
- Wisely HB. 1965. Studies on Ephemeroptera 3. (Walker); morphology and anatomy of the winged stages. New Zealand Journal of Science. 8:398–415.
- Wood JR, Resh VH, McEwan EM. 1982. Egg masses of nearctic sericostomatid caddisfly genera (Trichoptera). Annals of the Entomological Society of America. 75:430–434. doi: 10.1093/aesa/75.4.430
- Zwick P. 1973. Insecta: Plecoptera. Phylogenetisches System und Katalog. Das Tierreich 94. Berlin: Walter de Gruyter and Co.
- Zwick P. 1979. Revision of the stonefly family Eustheniidae (Plecoptera), with emphasis on the fauna of the Australian region. Aquatic Insects: International Journal of Freshwater Entomology. 1:17–50. doi: 10.1080/01650427909360975
- Zwick P. 2015. Notes on Madagascan stoneflies (Plecoptera: Notonemouridae). Zootaxa. 4059:169–180. doi: 10.11646/zootaxa.4059.1.9