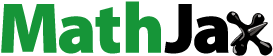
ABSTRACT
Marine strandlines provide habitat for a variety of littoral and terrestrial invertebrates, including arachnids. In this study we recorded spiders in strandlines at 35 sites on Banks Peninsula, New Zealand. On average, only 1.5 named species were recorded per thirty-minute hand search, and many sample visits (30%) recorded no spiders. Species accumulation curves suggested further species could be recorded with further sampling, but these may be tourist species rather than strandline residents. Thirty-eight species were recorded in total, with the introduced theridiid Steatoda capensis being the most common (30 records) and widespread (17 locations). The New Zealand endemic species Otagoa nova (25 records) (Toxopidae), Anoteropsis litoralis (15) and Anoteropsis hilaris (15) (Lycosidae), and the introduced Tenuiphantes tenuis (24) (Linyphiidae), were also common. Spiders were more frequent in strandlines on boulder beaches compared with shingle beaches, although there was no statistical differences in the numbers of species recorded on the different beach types.
Introduction
Marine strandlines, ‘driftlines’ or ‘wrack beds’ consist of accumulations of loose seaweed and other debris washed up on shore. Strandlines can remain in place for substantial periods and provide a resource for a range of arthropod detritivores, which in turn provide prey for a variety of predators and parasitoids (Hodge and Vink Citation2006; Hodge and Early Citation2016). Previous studies have examined how strandline invertebrates differ among different shore types, and investigated patterns in the spatial distribution of invertebrates within and between wrack beds (Phillips and Arthur Citation1994; Hodge and Jessop Citation1996; Hodge and Williams Citation2009). The temporal aspects of the strandline invertebrate community, such as the sequence of species colonisation and major seasonal trends in arthropod abundance and diversity, have also been investigated (Inglis Citation1989; Hodge and Arthur Citation1997; Hodge and Williams Citation2007, Citation2009; Yamazaki Citation2012).
It has been known for some time that spiders occur in marine strandlines: Backlund (Citation1945) and Egglishaw (Citation1965) listed the spiders found in large wrack beds in Scandinavia and NE England respectively, and Duffey (Citation1968, Citation2004) described a high diversity of species collected in drift lines in front of sand dunes in Scotland and Wales. Hodge and Vink (Citation2006) reported seven species of spider collected from strandlines around the coast of Kent in south east England.
Forster and Forster (Citation1999) described a number of spider species associated with New Zealand shorelines. Some species, such as Otagoa nova Forster, Citation1970, are specifically found above the high tide line and shelter under debris. Similarly, the katipō (Latrodectus katipo Powell, 1871), although more generally associated with coastal dunes, can be found under marine debris and driftwood on the upper shore (Griffiths Citation2001). In studies specifically examining strandlines in New Zealand, however, no spiders have been explicitly named: Inglis (Citation1989) did not report any spiders in his collections at New Brighton beach near Christchurch, and Dufour et al. (Citation2012) listed only an ‘arachnid’ from their samples of strandline invertebrates obtained in Otago.
The aim of this investigation was to produce a preliminary list of spider species associated with marine strandlines on New Zealand beaches. Here we describe the species recorded at 35 different locations around Banks Peninsula in Canterbury. The data obtained from the survey allowed us to compare the spiders collected on strandlines occurring on sandy beaches with those on shingle and stony shorelines, and assess what sampling effort may be required to gain good coverage of the spider assemblage occurring in this transient habitat.
Methods
Sampling and specimen identification
In this study strandlines were defined as accumulations of any size consisting of marine algae, eel grasses, and other organic debris (terrestrial plant material, grasses, straw, broken sticks etc.) that had been deposited or shaped by the high tide to produce a distinguishable line or major clumps of material. The searching of large pieces of driftwood and logs was deliberately avoided.
A total of 153 sample visits were made between January 2012 and February 2015 to 35 distinct locations on Banks Peninsula, from Sumner in the north to Kaitorete Spit in the south. Beaches were classified in terms of substrate: large stones and ‘boulders’, shingle/gravel, sand/mud. Sampling locations, classification of beach substrate and the number of visits made to each locality are listed in .
Table 1. Summary of sample sites, sample number, beach classification (Sa – sandy; Sh – shingle; Bo – boulders; Mu – mud), number of sample visits that recorded spiders and the total number of species recorded at each site.
The use of hand searching has been recommended for collecting spiders in strandlines (Duffey Citation1968; Hodge and Vink Citation2006). Therefore, in this study spiders were collected by searching through strandline material for approximately 30 min on each site visit, with specimens collected using a battery operated aspirator. Most of these collections were performed solely by the first author (SH); on some occasions when a field assistant was also present, the searches were of 15 min duration and therefore represented a total of 30 min collecting activity. On some occasions, especially when the strandline consisted of highly fragmented loose material, hand searching was supplemented by sieving material through a plastic colander onto a white plastic tray.
Specimens were stored in 80% ethanol, with the exception of katipō, which, as a protected species (Sirvid et al. Citation2012), were released at the site of capture. As only information on the presence/absence of a species was required, the presence of some of the larger conspicuous species (e.g. katipō, Nyssus coloripes Walckenaer, 1805, Anoteropsis litoralis Vink, Citation2002) was recorded in the field without the need of specimen collection. Similarly, collecting of large numbers of small specimens from the same location on the same date, and which were considered highly likely to be the same species, was also avoided. Identification of specimens was performed by reference to: Forster (Citation1970); Forster and Wilton (Citation1973); Blest (Citation1979); Forster (Citation1979); Court and Forster (Citation1988); Żabka (Citation1988); Vink (Citation2002); Paquin et al. (Citation2010); Marinov et al. (Citation2014); and Patoleta and Żabka (Citation2017). Voucher specimens have been retained at Canterbury Museum, Christchurch.
Data analysis
The relationship between the total species richness at each site and number of sampling visits was assessed using Spearman’s rank correlation. Differences in species richness among the three different beach substrates were assessed using a generalised linear model (GLM) with species richness (per site) designated as Poisson data, using a logarithmic link function and including sample visits to each site as a covariate. The probability of recording any spiders (including juveniles) at sites with different substrates was compared using a GLM defining the number of positive records at each site as a binomial variate, with the number of sample visits as the denominator and using a logit link function. The probability of recording the five most commonly occurring species (Steatoda capensis Hann, Citation1990; O. nova , Tenuiphantes tenuis (Blackwall, 1852), Anoteropsis hilaris (L. Koch, 1877) and A. litoralis) on each substrate was assessed using similar GLMs. For all GLMs the dispersion parameter was estimated during the analysis procedure. Genstat v16 software (VSN International Ltd, UK) was used for all of the above analyses.
To determine how species number accumulated as a function of sampling effort, sample interpolation (Species Diversity and Richness v4.1.2, Pisces Conservation Ltd, UK) was performed, based on 1000 iterations of a random resampling process without replacement. The interpolation procedure was performed for all data and for each of the three beach categories separately. Although sampling effort was not equal at each location, the species interpolation analysis was also performed using the distinct sites as the sample units to assess how the size or ‘grain’ of the sample might influence patterns in species accumulation.
Results
Fauna
Thirty-eight species of spider, belonging to 12 families, were recorded in the survey (). Seventeen of these species (45%) were only observed on one occasion over all 153 sampling visits. Steatoda capensis was the most frequent species, being recorded on 30 occasions, followed by O. nova (25), T. tenuis (24), A. hilaris (15) and A. litoralis (15). Four other species–Cryptachaea blattea (Urquhart, 1886), Diplocephalus cristatus (Blackwall, 1833), Hypoblemum albovittatum (Keyserling, 1882), and Erigone wiltoni Locket, 1973–were recorded on 10 or more occasions (). Of the 38 species collected, 19 are considered endemic to New Zealand, five species are native, and the remaining 14 species are introduced. We have designated the Hemicloea specimen as ‘introduced’, as this is an Australian genus that contains at least two unidentified species that are likely to have become naturalised in New Zealand ().
Table 2. Summary of spider species collected in a survey of strandlines at 35 Banks Peninsula sites (see ). New Zealand status: En-endemic, Na – native, In – introduced.
As expected, the survey recorded many species that are considered shoreline specialists. O. nova, a species that occurs above the high tide mark, is typically found under debris or stones and was recorded at 13 locations in this survey (Forster Citation1970). Marpissa marina (Goyen, 1892) is another endemic seashore dweller and is considered fairly common in Otago and Canterbury (Żabka and Pollard Citation2002). In our survey this species was only recorded on beaches dominated by larger stones and boulders. Anoteropsis litoralis is a lycosid that tends to occur on sandy beaches (and is well-camouflaged for this purpose), whereas another lycosid, Anoteropsis arenivaga (Dalmas, 1917), is typically found along dry river beds, lake edges and beaches, where its blue-grey colour provides excellent camouflage against grey stones and rocks. Anoteropsis arenivaga was only found under seaweed on the boulder beaches at Kaitorete Spit and Birdlings Flat: the other lycosids recorded in the survey, A. hilaris and A. litoralis, were not observed at either of these sites ().
Table 3. Summary of spider species recorded in strandlines at 35 sites on Banks Peninsula. X = species recorded at that site on at least one occasion. See for site codes.
Katipō were found at four locations, three of which involved strandlines along the fringes of sand dunes (Okains Bay, Le Bons Bay and Kaitorete Spit) where the strandlines tended to consist of large sheets of dry kelp. Katipō are typically found along sandy beaches, and among sand dunes with marram, pingao, spinifex grasses, although it is not unusual to find katipō associated with driftwood, debris, and refuse lying above the high tide mark (Griffiths Citation2001). The use of artificial cover objects to study katipō attests to their propensity for sheltering or building webs under beach ‘debris’ (e.g. Smith et al. Citation2014). As indicated in this survey, the use of strandlines by katipō appears not to be universal. Substantial populations of katipō occur at Kaitorete Spit, although the species was only recorded under dried seaweed on one of two sampling visits. Similarly, Hodge and Vink (Citation2017) previously reported that after over 380 searches of the strandlines at the sand dunes at New Brighton no katipō were found, even though the species was abundant in the adjacent sand dune grasses. The katipō specimens recorded at Duvauchelle were sub-adult and likely in the process of looking for a suitable site for web building.
Many of species collected are habitat generalists and are widespread around New Zealand. For example, Eriophora pustulosa (Walckenaer, 1841) is almost certainly of Australian origin but is found throughout New Zealand, including some subantractic islands. Similarly, Badumna longinqua (L. Koch, 1867), the Australian grey house spider, tends to occur in modified habitats and is also now widespread in New Zealand. Tenuiphantes tenuis is another species introduced to New Zealand that can be very common in pasture, parks and gardens, as well as native grassland habitats. Tenuiphantes tenuis was recorded on 24 occasions, at 16 different locations, and on all three types of beach ( and ). This species has been observed on the coast and under beach detritus in previous studies in the UK (Egglishaw Citation1965; Duffey Citation1968; Hogg Citation1995), and was the commonest spider collected in strandlines by Hodge and Vink (Citation2006) in the south of England. Araeoncus humilis (Blackwall, 1841) is another introduced linyphiid that was fairly widespread in this study (8 sites; and ). This species is associated with leaf litter and straw in its home range, and has also been reported to occur in coastal ‘seaweed litter’ (Harvey et al. Citation2002).
The endemic lycosid, A. hilaris, occurred at 12 locations, and represents another widespread species that occurs across New Zealand, often associated with gardens and farmland (Hodge and Vink Citation2000). The salticids Trite auricoma (Urquhart, 1886) and H. albovittatum are also considered common and widespread, and tend to occur under fallen leaves or among litter (Żabka and Pollard Citation2002). A number of the salticid specimens we collected were juvenile, or were adults that could not be identified to species. It is probable, therefore, that the actual number of salticid species utilising strandlines in this region will actually be higher than reported here.
Two theridiids, S. capensis and C. blattea, were widely collected in this survey, being recorded at 17 and 9 sites respectively. Steatoda capensis is an introduction from South Africa, and often found along beaches, where it is suspected of displacing katipō (Hann Citation1990). Cryptachaea blattea is a cosmopolitan species found in long grasses, low shrubs, and leaf and twig litter in beaches, regenerating scrub and young forest, where it builds a small web (Vink et al. Citation2009).
Sampling effort, species accumulation and comparison of shore types
The number of species recorded on any one sampling visit ranged from zero to a maximum of seven (at Takamatua Bay on 26th January 2014) (). Overall, 94 samples (61%) contained specimens identifiable to species level, with a further 13 samples containing only juvenile spiders. This resulted in 46 of the 153 searches (30%) producing no spiders at all, and 59 (39%) producing no specimens that could be identified to species (). Because of the large proportion of samples with no spiders, a mean of only 1.46 species were recorded per site visit, and only 22 (14%) of samples contained four or more species (). The relationship between the species recorded and sampling effort was not straightforward: seven species were recorded at Port Levy after only three visits, whereas the small beach at Corsair Bay produced only two species after nine visits (). This pattern resulted in only a moderate correlation between the number of species recorded at each site and the number of sampling visits made (rS = 0.360; N = 35; P = 0.034).
Figure 1. Frequency plot illustrating the number of named spider species collected in 153 thirty-minute hand-searches of marine strandlines at 35 sites on Banks Peninsula, New Zealand.
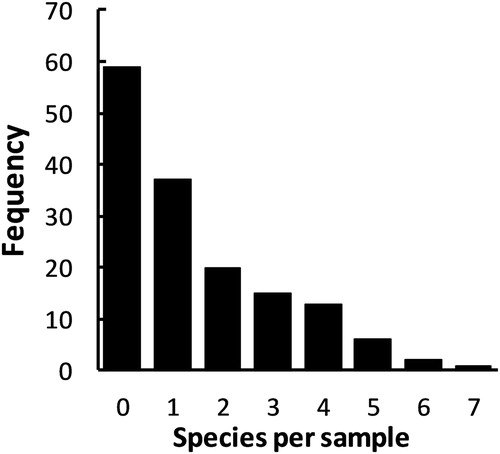
The species accumulation curves using individual collecting visits or distinct locations as the sample unit did not reach a plateau, which suggests that the list of species recorded in this habitat (in this geographic area) is still incomplete (). When using individual sampling visits as the sample unit, species accumulated much more slowly on the shingle beaches compared with sand and boulder beaches ((a)). This is an artefact of the different probabilities of finding identifiable spiders on the different beach types: identifiable spiders were recorded in 81.6% of samples from strandlines occurring on boulder beaches, and 66.7% of samples from sandy beaches but only 47.1% of samples from shingle beaches. The discrepancy among shore types in terms of species accumulation patterns was not apparent when using sites as the sampling unit ((b)).
Figure 2. Sample interpolation species accumulation curves for spiders occurring in marine strandlines on Banks Peninsula, New Zealand using (A) individual 30-minute hand searches or (B) distinct sites as the sampling unit.
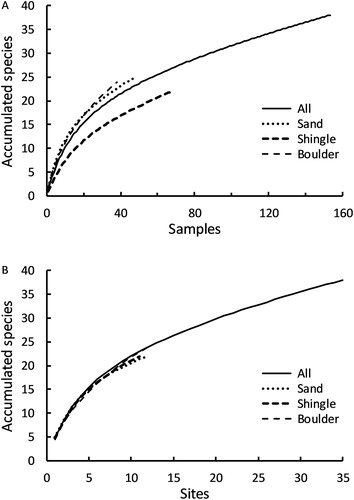
Overall, the relative scarcity of species among samples, and the large proportion of samples where no spiders were recorded, implies that any future surveys of strandline spiders would require large numbers of sample visits, including visits to different areas and different beach types, before an asymptote in accumulated species number would occur. Additionally, the species accumulation curves for individual searches and sites were well explained by power curve equations of the form:where a and b are constants, and 0 < b < 1. This relationship is equivalent to a non-decreasing form of the species-area relationship (Scheiner Citation2003; Ugland et al. Citation2003), and suggests that new species records will be recorded with additional sampling, albeit at an increasingly lower rate as sampling continues. The equations produced (both with r2 > 0.995) were:
The results of the GLM analysis suggested that the species richness in strandlines did not differ among the different classes of beach substrate (), which is supported by the overall species counts for each shore type: 22 spider species were recorded at the 12 sandy sites, 22 species at the 11 shingle sites and 24 species at the 12 boulder beach sites (). The probability of finding any spiders during a sampling visit was, however, higher on boulder beaches compared to shingle beaches (). Of the commoner species, O. nova and T. tenuis exhibited a tendency to be found most often on boulder beaches. Conversely, A. litoralis was over ten times more likely to be found on sandy beaches compared to boulder and shingle beaches. Steatoda capensis and A. hilaris did not exhibit any statistically significant biases towards a particular shore type.
Table 4. GLM predicted means (± se) of spiders in strandlines occurring on different forms of beaches on Banks Peninsula. (P-value obtained from GLMs; see Methods for details of analysis).
Discussion
This survey recorded thirty-eight species of spider occurring in marine strandlines, and provides an initial checklist of species that can be extended by future research. The spider assemblage was variable in terms of biogeographic status of the species collected and their ecology, in that there appeared no particular bias towards endemic, native or introduced species, and the collections included some species considered shore specialists and some that are habitat generalists. In terms of behaviour, we recorded both web building species and cursorial hunters, and in terms of size there were relatively large species such as Lycosidae and species that are only a few millimetres in length such as the Hahniidae.
Spiders are associated with other ephemeral decomposing resources, such as rotting fruit, because these resources attract high densities of acceptable detritivorous prey (e.g. Hodge and Vink Citation2007). It was recently demonstrated that, under laboratory conditions, spiders collected in this study (A. hilaris, C. blattea, N. coloripes and Steotoda capensis) could capture and consume species of flies that are commonly found in strandlines in this region (Hodge et al. Citation2017). By integrating themselves into the strandline trophic network, and then becoming prey to other species higher up the food chain, spiders therefore represent a link in the transfer of nutrients of marine origin into the terrestrial system.
It is conceded that many of the spiders we recorded may have been using the strandline as a daytime sheltering site rather than using it as a foraging site, and some species are probably ‘vagrants’ or ‘tourists’ rather than habitual members of the strandline assemblage. The power equations that described the species accumulation curves, whether derived from surveying an expanding area or taking additional samples over time, can indicate a high degree of species turnover in the assemblage, or that the habitat is prone to the occurrence of vagrant (Abbott Citation1983). In our system, the upper-shore strandlines we sampled were often bordered by sand dunes, agricultural land, scrub and road verges, and it can be readily envisaged that spiders could stray into strandlines from these proximal terrestrial habitats. When performing analysis of ecological community structure and dynamics, there is sometimes debate over whether vagrant species should be included in data set, as their low numbers and transience implies they may play only a ecological minor role (Taylor et al. Citation2018). In the case of strandline spiders, however, even though the actual species involved may differ temporally and spatially, vagrants as a group can constitute a major proportion of the assemblage and their impacts in terms of interactions with other spiders and as part of trophic networks may still be considerable. Ultimately, further work is required to ascertain which of the species we recorded consistently occur in the strandline habitat and/ or determine which species have some ecological function within it.
There were no spiders recorded in 30% of samples, and after working on strandline invertebrates for some time it becomes apparent that these zero counts can be caused by a number of different mechanisms, including a lack of strandline, the age of strandline, and an inadequate sampling regime. Additionally, zero records can occur due to ecological processes such as actual absence of the species, effective rarity of the species, or seasonal patterns resulting in species absences when the sampling visit is made. Future, more systematic, studies of strandline invertebrates where highly zero-inflated count or presence–absence data are obtained might look more closely at the different mechanisms that can produce zero counts and utilise more appropriate analytical techniques such as zero-inflated data distributions or ‘hurdle’ models (e.g. Martin et al. Citation2005). In some cases, zero counts could be avoided by increasing sampling effort, although, in our case, extending the search time when there is no suitable strandline present would obviously be superfluous (Hodge and Vink Citation2007). Consideration of the tidal cycle prior to making sampling visits, in order to estimate how recently any wrack material would have been deposited and thus how long it has likely remained free from submersion, could also help in reducing the occurrence of ‘empty’ samples.
There was difficulty in unequivocally classifying beach type in some cases, especially where different substrates occurred within short distances, and where multiple ‘sub-sites’ were explored within one major area (e.g. Quail island). Although there were no differences in spider species richness among the three substrate types, spiders were more likely to occur in samples taken from strandlines on boulder beaches than on sandy and shingle beaches. Also, some individual shore species (e.g. O. nova) were more likely to occur on boulder beaches, and others more likely to occur on sandy beaches (e.g. A. litoralis). These findings are likely a reflection of the ecology and habitat use of these species and require further study to assess their consistency and repeatability in different geographic regions. Forgie et al. (Citation2013), in a survey of beaches around New Zealand, reported that spiders were less abundant on disturbed gravel beaches when compared with other more stable substrates, and frequency of disturbance is also likely to play a role in the occupation of strandlines by spiders, although how this process might relate to beach substrate is still unclear.
Strandlines can be an important component of coastal ecosystems, enhancing local biodiversity and providing a habitat for specialised species or those that are nationally rare (Whitehouse Citation2005). In this survey nineteen endemic species were recorded, including the endangered katipō, which was recorded at four locations. There is sometimes pressure on civic authorities to remove strandlines from amenity beaches where accumulations of seaweed are considered unsightly (Llewellyn and Shackley Citation1996). Beach cleaning or ‘grooming’ appears not to be common in this area of New Zealand, and during this survey was observed at only one site (Sumner) on one sampling visit. Instead, strandline invertebrate communities in New Zealand appear more threatened by harvesting of beach-cast seaweed for commercial use, and by the use of beaches by recreational vehicles (Stephenson Citation1999; Zemke-White et al. Citation2005). In Australia, recent research has shown that artificially augmenting strandlines on the upper shore can increase the density of invertebrate prey to promote conservation of shore birds (Schlacher et al. Citation2017).
This study has provided an initial list of 38 species of spider occurring in marine strandlines in New Zealand. In terms of the wider arachnid assemblage, species of Opiliones (harvestmen), Psuedoscorpiones and Acari (mites) were also recorded in the survey, and the ecology of these taxa occurring in New Zealand strandlines warrants further investigation. Although individual sites were only visited a small number of times, we feel the 153 visits for the Banks Peninsula as a whole should be considered adequate for an initial survey. However, further sampling visits to Banks Peninsula, especially to new locations, are still likely to produce records of additional spider species. Expanding the search area to beaches in North and South Canterbury, or investigating strandlines on more distant areas of New Zealand’s coast, is required to gain further insight into which species are regular occupants of strandlines and which are vagrants. Finally, more detailed study of the behaviour of the spiders found within this habitat is required in order to elucidate their ecological roles, their interactions with other species and their importance in the various trophic relationships that occur within the strandline arena.
Acknowledgments
The authors thank Mick Whittle and Mike Bowie for help with field work, and the property owners along the Banks Peninsula Track and Decanter Bay that granted beach access.
Disclosure statement
No potential conflict of interest was reported by the authors.
Additional information
Funding
References
- Abbott I. 1983. The meaning of z in species/area regressions and the study of species turnover in island biogeography. Oikos. 41:385–390. doi: 10.2307/3544097
- Backlund HO. 1945. The wrack fauna of Sweden and Finland. Opusculum Entomologica Supplementum. 5:1–236.
- Blest AD. 1979. The spiders of New Zealand. Part V. Linyphiidae-Mynogleninae. Otago Museum Bulletin. 5:95–173.
- Court DJ, Forster RR. 1988. The spiders of New Zealand. Part VI. Araneidae-Araneinae. Otago Museum Bulletin. 6:68–124.
- Duffey E. 1968. An ecological analysis of the spider fauna of sand dunes. Journal of Animal Eology. 37:641–674. doi: 10.2307/3080
- Duffey E. 2004. The efficiency of timed hand-collecting combined with a habitat classification versus pitfall trapping for studies of sand dune spider faunas. Newsletter of the British Arachnological Society. 99:2–4.
- Dufour C, Probert PK, Savage C. 2012. Macrofaunal colonisation of stranded Durvillaea antarctica on a southern New Zealand exposed sandy beach. New Zealand Journal of Marine and Freshwater Research. 46:369–383. doi: 10.1080/00288330.2012.676557
- Egglishaw HJ. 1965. Observations on the fauna of wrack beds. Transactions Society British Entomology. 16:189–216.
- Forgie SA, St. John MG, Wiser SK. 2013. Invertebrate communities and drivers of their composition on gravel beaches in New Zealand. New Zealand Journal of Ecology. 37:95–104.
- Forster R, Forster L. 1999. Spiders of New Zealand and their Worldwide Kin. Dunedin: University of Otago Press, pp270.
- Forster RR. 1970. The spiders of New Zealand. Part III. Desidae, Dictynidae, Hahniidae, Amaurobioididae, Nicodamidae. Otago Museum Bulletin. 3:1–184.
- Forster RR. 1979. The spiders of New Zealand. Part V. Cycloctenidae, Gnaphosidae, Clubionidae. Otago Museum Bulletin. 5:8–95.
- Forster RR, Wilton CL. 1973. The spiders of New Zealand. Part IV. Agelenidae, Stiphidiidae, Amphinectidae, Amaurobiidae, Neolanidae, Ctenidae, Psechridae. Otago Museum Bulletin. 4:1–309.
- Griffiths JW. 2001. Web site characteristics, dispersal and species status of New Zealand’s katipō spiders, Latrodectus katipo and L. atritus [PhD thesis]. Lincoln: Lincoln University
- Hann SW. 1990. Evidence for the displacement of an endemic New Zealand spider, Latrodectus katipo Powell by the South African species Steatoda capensis Hann (Araneae: Theridiidae). New Zealand Journal of Zoology. 17:295–307. doi: 10.1080/03014223.1990.10422937
- Harvey PR, Nellist DR, Telfer MG. 2002. Provisional atlas of British spiders (Arachnida, Araneae), Volume 1. UK: Biological Records Centre. 191pp.
- Hodge S, Arthur W. 1997. Asymmetric interactions between species of seaweed fly. Journal of Animal Ecology. 66:743–754. doi: 10.2307/5925
- Hodge S, Early JE. 2016. Hymenoptera associated with marine strandlines at Christchurch and Banks Peninsula. New Zealand Entomologist. 39:117–128. doi: 10.1080/00779962.2016.1215034
- Hodge S, Jessop L. 1996. A note on Coleoptera found in wrack beds on the north-east coast of England. The Coleopterist. 5:7–12.
- Hodge S, Vink C. 2006. Spiders found on strandlines around the coast of Kent. Newsletter of the British Arachnological Society. 105:3–5.
- Hodge S, Vink C. 2007. Do epigeal spiders show a spatial association with patches of rotting fruit? Newsletter of the British Arachnological Society. 109:7–10.
- Hodge S, Vink CJ. 2000. An evaluation of Lycosa hilaris as a bioindicator of organophosphate insecticide contamination. New Zealand Plant Protection. 53:226–229. doi: 10.30843/nzpp.2000.53.3693
- Hodge S, Vink CJ. 2017. Evidence of absence is not proof of absence: the case of the New Brighton katipō? New Zealand Journal of Zoology. 44:14–24. doi: 10.1080/03014223.2016.1227343
- Hodge S, Vink CJ, Curtis N. 2017. Survival of strandline spiders maintained on a diet of the kelp fly Thoracochaeta ancudensis (Sphaeroceridae). The Weta. 51:36–46.
- Hodge S, Williams A. 2007. Coleoptera found in wrack beds and strandlines around the Kent coast. British Journal of Entomology & Natural History. 20:61–70.
- Hodge S, Williams A. 2009. Further records of Coleoptera from strandlines on the south coast of Kent. The Coleopterist. 18:111–119.
- Hogg M. 1995. Some shoreline spiders from Scotland. Newsletter of the British Arachnological Society. 72:10.
- Inglis G. 1989. The colonisation and degradation of stranded Macrocystis pyrifera (L.) C. Ag. by the macrofauna of a New Zealand sandy beach. Journal of Experimental Marine Biology and Ecology. 125:203–217. doi: 10.1016/0022-0981(89)90097-X
- Llewellyn PJ, Shackley SE. 1996. The effects of mechanical beach-cleaning on invertebrate populations. British Wildlife. 7:147–155.
- Marinov M, Vink CJ, Jones D, Kumarasinghe L. 2014. Lucid key to Theridiidae spiders of biosecurity importance to New Zealand. Christchurch: Ministry of Primary Industries.
- Martin TG, Wintle BA, Rhodes JR, Kuhnert PM, Field SA, Low-Choy SJ, Tyre AJ, Possingham HP. 2005. Zero tolerance ecology: improving ecological inference by modelling the source of zero observations. Ecology Letters. 8:1235–1246. doi: 10.1111/j.1461-0248.2005.00826.x
- Paquin P, Vink CJ, Duperre N. 2010. Spiders of New Zealand. Lincoln: Manaaki Whenua Press, 188pp.
- Patoleta B, Żabka M. 2017. A new species of Trite Simon, 1885 (Araneae: Salticidae) from New Zealand, with remarks on Trite relationships and radiation. PeerJ. 5:e3463. doi: 10.7717/peerj.3463
- Phillips DS, Arthur W. 1994. Observations on the distribution of seaweed fly larvae and other invertebrates within a wrack-bed. The Entomologist. 113:154–163.
- Scheiner SM. 2003. Six types of species-area curves. Global Ecology and Biogeography. 12:441–447. doi: 10.1046/j.1466-822X.2003.00061.x
- Schlacher TA, Hutton BM, Gilby BL, Porch N, Maguire GS, Maslo B, Connolly RM, Olds AD, Weston MA. 2017. Algal subsidies enhance invertebrate prey for threatened shorebirds: a novel conservation tool on ocean beaches? Estuarine, Coastal and Shelf Science. 191:28–38. doi: 10.1016/j.ecss.2017.04.004
- Sirvid PJ, Vink CJ, Wakelin MD, Fitzgerald BM, Hitchmough RA, Stringer IAN. 2012. The conservation status of New Zealand Araneae. New Zealand Entomologist. 35:85–90. doi: 10.1080/00779962.2012.686310
- Smith VR, Vink CJ, Nager RG, Ross J, Paterson AM. 2014. Abundance of Latrodectus katipo Powell, 1871 is affected by vegetation type and season. Journal of Insect Conservation. 18:397–405. doi: 10.1007/s10841-014-9648-2
- Stephenson G. 1999. Vehicle impacts on the biota of sandy beaches and coastal dunes: a review from a New Zealand perspective. Science for Conservation 121, NZ: Department of Conservation.
- Taylor SJN, Evans BS, White EP, Hurlbert AH. 2018. The prevalence and impact of transient species in ecological communities. Ecology. 99:1825–1835. doi: 10.1002/ecy.2398
- Ugland KI, Gray JS, Ellingsen KE. 2003. The species-accumulation curve and estimation of species richness. Journal of Animal Ecology. 72:888–897. doi: 10.1046/j.1365-2656.2003.00748.x
- Vink CJ. 2002. Lycosidae (Arachnida: Araneae). Fauna of New Zealand. 44:1–94.
- Vink CJ, Dupérré N, Paquin P, Fitzgerald BM, Sirvid PJ. 2009. The cosmopolitan spider Cryptachaea blattea (Urquhart 1886) (Araneae: Theridiidae): Redescription, including COI sequence, and new synonymy. Zootaxa. 2133:55–63. doi: 10.11646/zootaxa.2133.1.5
- Whitehouse A. 2005. Assessing the importance of strandlines for invertebrate diversity. Bulletin of the Dipterist’s Forum. 60:16.
- Yamazaki K. 2012. Seasonal changes in seaweed deposition, seaweed fly abundance, and parasitism at the pupal stage along sandy beaches in central Japan. Entomological Science. 15:28–34. doi: 10.1111/j.1479-8298.2011.00477.x
- Żabka M. 1988. Salticidae (Araneae) of Oriental, Australian and Pacific regions, III. Annales Zoologici Warsaw. 41:421–479.
- Żabka M, Pollard SD. 2002. A check-list of Salticidae (Arachnida: Araneae) of New Zealand. Records of the Canterbury Museum. 16:73–82.
- Zemke-White WL, Speed SR, McClary DJ. 2005. Beach-cast seaweed: a review. Ministry of Fisheries, New Zealand Fisheries Assessment Report 2005144.