ABSTRACT
Fenced ecosanctuaries may reduce predator presence at reserves by incorporating deterrents into pest management programmes. We quantified rodent visits to the steel hood of ecosanctuary fencing and illuminated our experimental sites to assess whether light could deter ship rats (Rattus rattus) and house mice (Mus musculus). Additionally, we assessed how mice used fencing in the presence and absence of ship rats by utilising two types of fence sections: one accessible to mice alone (along fencing within an ecosanctuary) and one accessible to mice and rats (at perimeter fence sites opposite pasture or forest habitat). We monitored sites using cameras and tracking cards within the fence hood and at tunnels at the fence base. Along the internal fence, mice were never observed within the hood but marked 40% of tunnels at the fence base. Along the perimeter fence, mice made four visits to the hood and marked 28% of tunnels at the fence base. Rats travelled exclusively within the fence hood (n = 42). Light did not reduce rat sightings but adjacent habitat affected their presence (forest > pasture; p ≤ 0.01). Positioning future ecosanctuary fencing alongside pasture and maintaining open corridors opposite fences at current ecosanctuaries may reduce rat presence.
Introduction
Ecosanctuaries provide many conservation benefits to vulnerable island and mainland ecosystems (Pech and Maitland Citation2016; Innes et al. Citation2019). Critical components of management at pest-fenced ecosanctuaries include: (a) knowledge of the predominant pest species that threaten pest-free ecosystems; and (b) deterrents that ensure vulnerable sections of pest fencing are protected from reinvasion. In New Zealand, ‘pest-proof fences’ have been built to limit or prevent reinvasion after mammal pest eradication (Day and MacGibbon Citation2007; Connolly et al. Citation2009); such fences have also been built elsewhere (e.g. Australia – Long and Robley Citation2004; Hawaii – Young et al. Citation2013). Fence construction and initial pest eradication represent a significant financial investment and sanctuaries must remain protected, especially after receiving translocated threatened taxa (Scofield et al. Citation2011; Innes et al. Citation2012; Scofield and Cullen Citation2012). However, New Zealand’s existing pest-fences have characteristics that expose predator-free sanctuaries to reinvasion by introduced mammals (Burns et al. Citation2012). For example, fencing across peninsula sanctuaries does not prevent pests walking or swimming around the fence ends, and trees falling on fences create reinvasion opportunities for mammalian pests at forested ecosanctuaries (Burns et al. Citation2012). Furthermore, the probability of incursion by at least one mammalian pest within 24 h at a fence breach is estimated as 99% in summer and 85% in winter (Connolly et al. Citation2009).
Rodents are the most frequent mammalian predators present at sanctuary fencing (29.3% of all pest sightings). Mice (Mus musculus) often travel along the base of ecosanctuary fencing and ship rats (Rattus rattus) regularly utilise the gutter of the fence hood as a safe thoroughfare (Connolly et al. Citation2009). Rodents’ recurrent visits to pest fencing and prolific use of the fence hood may increase the probability that rats and mice will locate breaches at the fence base or structural damage within the hood, and lead to a greater chance of invasion. Reinvasion by rats (both R. rattus and R. norvegicus) threaten populations of forest passerines (Innes et al. Citation1999; Armstrong et al. Citation2006), herpetofauna (Towns and Daugherty Citation1994) and invertebrates (Olson et al. Citation2006; Watts et al. Citation2011), and the seed banks of endemic vegetation (García Citation2002). Similarly, when mice reinvade sanctuaries, they are released from predation and competition and their foraging patterns can cause ecological damage (Watts et al. Citation2011, Citation2017).
Using deterrents at vulnerable sections of sanctuary fencing may allow conservation managers to minimise rodent reinvasion at ecosanctuaries. For example, applying predation cues can induce avoidance behaviour (Schakner and Blumstein Citation2013; Krijger et al. Citation2017), and manipulating rodents’ perception of predation risk could offer a novel technique for protecting the endemic fauna within ecosanctuaries (Farnworth et al. Citation2016). Prey animals, including rodents, utilise direct and indirect predation cues to assess risk and inform their foraging decisions (Orrock et al. Citation2004; Apfelbach et al. Citation2005; Fanson et al. Citation2008). Direct cues suggest the immediate presence of a predator, while indirect cues inform prey of the probability of encountering a predator and convey information regarding general predation risk (Thorson et al. Citation1998; Nersesian et al. Citation2012).
Illumination is considered an indirect predation cue, and nocturnal rodents often decrease movement (Dice Citation1945; Metzgar Citation1967) or avoid activity under moonlight (Kramer and Birney Citation2001; Shapira et al. Citation2013) because increased ambient lighting improves predators’ hunting success (Clarke Citation1983; Prugh and Golden Citation2014). Wild ship rats and non-commensal wild house mice also modify their behaviour under artificial illumination (Farnworth et al. Citation2016; Farnworth et al. Citation2019), indicating that sanctuary managers could exploit the tendency of rodents to avoid illuminated areas by utilising light as a deterrent at ecosanctuaries.
The purpose of this study was to: (i) document the dominant rodent species utilising the hood of pest fencing at Maungatautari Ecological Island, New Zealand; and (ii) assess whether illuminating sections of fencing could reduce rodent visitation rates. Typically, pest fences in New Zealand are constructed from marine grade (‘316’) stainless steel mesh (6 × 25 mm) to prevent mammals travelling or burrowing into ecosanctuaries. Additionally, a folded steel hood is attached to the top of the fence with a rolled gutter extending outwards – an important feature that prohibits entry by climbing mammals (Day and MacGibbon Citation2007). A decade has elapsed since the aluminium rolled hood of pest proof fencing was first identified as a hotspot of ship rat activity (see Connolly et al. Citation2009 for details), and an assessment of rodent presence at pest fencing seemed prudent for forthcoming pest management decisions, especially considering New Zealand’s ‘Predator Free 2050’ campaign (Russell et al. Citation2015). While mice have never been sighted within the hood of pest-proof fencing (Connolly et al. Citation2009), they may travel along the hood if rat populations are eradicated or even controlled to low levels. In these instances, populations of mice may increase (Caut et al. Citation2007) and they may utilise pest fencing more regularly. We therefore recorded rodent visitation rates at pest fencing along two different types of enclosures: one where the fence was accessible only to mice (along an internally fenced ‘cell’ within Maungatautari where all mammalian pests but mice were eradicated - henceforth referred to as the ‘Southern Enclosure fence’) and one where the fence was accessible to both mice and rats (along the perimeter fence of Maungatautari, where minimal pest control occurs - henceforth referred to as the ‘external fence’). Because ship rats behave aggressively towards mice (Bridgman et al. Citation2013) and exclude them from food sources (Ruscoe and Murphy Citation2005), we predicted that ship rats would be the most prevalent species within the hood of the external fence but that mice would utilise the hood of the Southern Enclosure fence where rats were absent. Furthermore, we applied artificial light to sites along the Southern Enclosure fence and the external fence to assess whether both ship rats and mice would avoid illuminated sections of fencing.
Methods
Study site
Maungatautari (38°03’S, 175°33’E) is an eroded volcanic cone situated in the central Waikato region of the North Island of New Zealand (Clarkson Citation2002). In 2004, the Maungatautari Ecological Island Trust built two enclosures (a 35 ha ‘Northern Enclosure’ and a 65 ha ‘Southern Enclosure’; ) and eradicated mammalian pests, including mice, from within them as pilot studies to assess feasibility for the main mountain eradication (Speedy et al. Citation2007). In 2006, a 47-km fence (XcluderTM Pest Proof Fencing Ltd; Rotorua, New Zealand) was built to surround the remaining 3363-ha forest area (), and all introduced mammals within the reserve were eradicated by trapping and poisoning (Speedy et al. Citation2007). Since the eradication of larger introduced mammals, mice have reinvaded the main enclosure and have increased greatly in abundance on the main mountain, especially after the end of mouse-control efforts in February 2012. Although there have been some short-lived mouse reinvasions of the pilot enclosures, since April 2005 (Northern Enclosure) and July 2006 (Southern Enclosure; Speedy et al. Citation2007) they have largely remained mouse-free (Watts et al. Citation2017).
Figure 1. Boundary fence lines of Maungatautari Ecological Island indicating where rodents are present. The internal fences run between the Main Sanctuary (light grey shading; mice present) and the Northern and Southern Enclosures (black shading; mice absent). The external perimeter fence (solid black line) runs between the Main Sanctuary and pasture or external forest fragments (dark grey shading), creating a boundary between areas where only mice are present (Main Sanctuary) and where all predator species are present (pasture and forest fragments). Experimental stations are shown (distance not to scale) where a = external stations opposite pasture habitat, b = external stations opposite forest habitat, and c = internal stations opposite forest habitat.
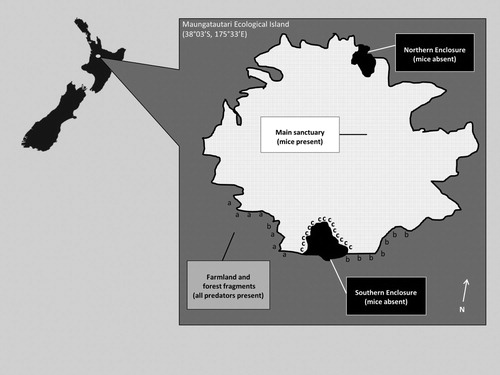
We confined our study to two 11-night periods across two months (6–16 December 2015 and 5–15 January 2016) in summer (average daily temperature 19° C; average daily humidity 73%), during the new moon phase of the lunar cycle, which allowed us to rotate treatments (see below) across our experimental stations while minimising the impact of moonlight as a confounding variable. Stations (see ‘Procedures and treatments’ for details) set up along the external fence (n = 15) were spaced a minimum of 200 m apart (based on previous research on rodents’ use of pest fencing in New Zealand; Connolly et al. Citation2009) while internal stations (n = 15) were spaced at 150 m intervals along the fencing that encircles the Southern Enclosure of Maungatautari (). As seven or eight of the 15 external stations were set up on any one night, the total distance between any two operating external stations typically exceeded 200 m, which further reduced home range overlap of ship rats, a species known to roam widely (typically 100–300 m range length in North Island podocarp-broadleaved forest; Innes Citation2005). Similarly, along the Southern Enclosure fence, seven or eight of the 15 internal stations were set up on any one night. External stations were initially set up opposite pasture as it was the dominant habitat, and earlier research (Connolly et al. Citation2009) indicated that rodent sightings did not differ between pasture and forest habitats. However, our preliminary observations revealed few rats (four rat sightings between 6 and 16 December 2015), so we moved seven of the 15 stations to sites opposite forest habitat. The internal stations along the Southern Enclosure fence were all opposite forest habitat.
Procedures and treatments
Each station was set up with an infra-red trail camera (HC600 Hyperfire Covert & PC900 Covert Pro; Reconyx, Wisconsin) that was attached with cable ties to the steel brackets that hold up the fence’s curved steel hood (). In addition to being motion-activated (providing three photos when triggered), each camera was also set to take pictures continuously at five-second intervals. Cameras were positioned to capture images of animals using the underside of the hood, and lamps were positioned c. 1 m from the camera.
Figure 2. (A) A section of XcluderTM brand pest-fencing, which surrounds Maungatautari Ecological Island, labelled to show design features that prevent mammalian predators from entering the reserve; (B) as (A), but showing the attachment of equipment that we used on the external face of the fence.
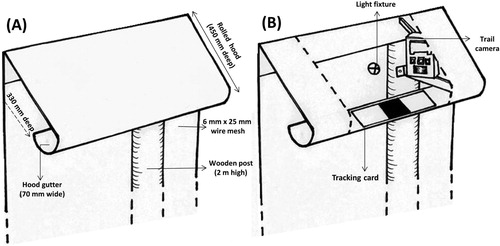
During our first period of data collection (6-16 December 2015), we detected few rats or mice, and subsequently, in our second monitoring period (5-15 January 2016), one standard tracking card (Black Trakka; Gotcha Traps, New Zealand) was placed in the hood on test nights to capture footprints of any animals undetected by cameras. Tracking cards were cut lengthways and fitted into the gutter of the hood at each station (B). We also placed a tracking tunnel (Black Trakka; Gotcha Traps, New Zealand) at the base of the fence underneath our stations to record rodent presence on the ground. Tracking cards from the fence hood were replaced daily, while cards at the base of the fence were collected after an average of six days. Tracking cards and tunnels were not baited to avoid luring rodents to our sites.
Each station was systematically allocated one of the three treatments at a time: light (an illuminated lighting fixture); dark (an unilluminated lighting fixture); and baseline (dark with no lighting fixture present to control for any effect of equipment). Artificial illumination was supplied by an LED lamp (1.6 W, 100 lumen, 5700 K cool white light; SY-CL02; Senyoo, China). The lamp had a magnetic base which allowed it to be attached vertically to the underside of the metal fence hood (A & B). The lamp had four modes of brightness (2; 50; 75; and 100%) and the second mode (50%) was selected for our experiment to ensure the lamp would have enough battery capacity to run overnight without significant dimming. We measured illuminance (starting at 1000 lux as measured by a digital light metre [Iso-Tech 1335; RS Components, United Kingdom]) over a ten-hour period in the lab and found light intensity decreased linearly by an average of 37 lux per hour. At Maungatautari, the lamp was fixed to a marked location on the underside of the hood that was 8 cm away from the lip of the curved underside (B). At this distance, light was projected at an intensity of 1000 lux along the closest 10 cm of the gutter of the hood but attenuated with distance to: 500 lux at 20 cm away from the lamp; 80 lux at 40 cm away; and 40 lux at 60 cm away. Light intensity was minimal at the base of the fence (0.8 lux). Stations were assigned a treatment for three nights. At the end of three nights, a break of at least four days was taken while the remaining seven or eight stations were set up for their allocated treatment. This continued until each station had three nights with each of the three treatments. Treatments began each night at 2000 h (30–40 mins before sunset) and lasted approximately 12 h. Trail camera batteries and memory cards were replaced every day. Photos were removed from memory cards and examined on a high-resolution (1920 × 1880 p) computer screen (Optiplex 9010; Dell, China). All stations were accessed daily using a vehicle track that runs parallel to the pest-fence.
Analyses
Our analyses excluded data from five nights (two for internal stations; three for external stations) where the battery of lighting fixtures failed or the camera angle produced a poor view. All statistical analyses were conducted using Statistica (Statistica 12; StatSoft, United States) and GenStat (GenStat 18; VSN International Ltd, Hemel Hempstead, United Kingdom).
External stations
We reviewed images from the cameras and counted the number of visits that rodents made to stations within the hood of the fence. Any part of an animal, tail included, that was seen in a camera field of view was classed as a visit. Rat visits were very sparse (i.e. the maximum number of visits to a station in one night was three) and visits were separated by several hours. We were therefore confident that each visit could be counted as independent. Rat visits were amalgamated across the three-day (or less if set-up errors occurred – see above) treatment period into station by treatment combinations, and analysed with generalised linear mixed models that used daily ‘visit rate’ values (with the number of nights as the rate offset) and Poisson residuals. Analyses tested for fixed ‘treatment’, ‘habitat’ and ‘month’ effects, and recognised ‘station’ and ‘treatment-period’ as random effects. Statistical significance was determined from likelihood ratio (LR) tests. Simple descriptive statistics were reported for mice, as few visits were made to external stations. The number of marked tracking cards from the hood of the fence was compared to the number of unmarked cards across treatments using Pearson’s chi square test (adjusted for unequal sample sizes – Baseline [n = 18], Light [n = 22], Dark [n = 22]). Treatment effects were not examined for tracking cards at the base of the fence because light attenuated rapidly (0.8 lux at the fence base), though the presence of mice at the fence base was compared between internal and external stations using Pearson’s chi square statistic to compare differences in tracking rates.
Internal stations
Simple descriptive statistics are reported for mice as we did not record any observations of mice within the hood at internal stations. Tracking cards at the base of the fence were not analysed for treatment effects due to significant light attenuation (see above).
Results
External stations
Forty-two observations of ship rats (11 at baseline stations; 15 at illuminated stations; 16 at dark stations) and four observations of mice (3 at baseline stations; 1 at a dark station) were recorded within the hood of the external perimeter fence over 131 sampling nights (). Treatment did not affect ship rat sightings (LR = 0.33, df = 2, p = 0.85) but habitat (forest > pasture: LR = 10.38, df = 1, p < 0.01) and month (January > December: LR = 5.24, df = 1, p = 0.02) significantly influenced the number of observations (). Including ‘station’ and ‘treatment-period’ as random effects within the model showed ‘station’ was not significant (LR = 1.03, p = 0.31) but ‘treatment-period’ was significant (LR = 2.89, p = 0.05), indicating that treatment effects were larger during some periods (i.e. groups of three-night treatment exposures) than others. Observations of mice were too low to determine any treatment effect.
Table 1. Total number of rodent visits detected at external stations by device type (trail camera vs. tracking cards) and location (hood of fence vs. base of fence) and across habitats (forest vs. pasture) for baseline, illuminated and dark treatments.
Thirty-five percent (22 out of 62) of all tracking cards placed in the hood were tracked by rats (10 out of 18 at baseline stations, 5 out of 22 at illuminated stations and 7 out of 22 at dark stations). We found no significant difference in the tracking rates of rats across treatments (X2 = 4.87, df = 2, p = 0.09) and no tracking tunnels placed at the base of the external perimeter fence were tracked by rats (0 out of 18; ). There were five instances where tracking cards positioned in the hood were marked but no camera observations were recorded. Additionally, there were eight instances where cameras captured images of ship rats but the tracking cards remained unmarked. Tracking by mice was higher on cards placed at the base of the perimeter fence (28%; 5 out of 18 cards tracked) than on cards placed within the hood (2%; 1 out of 62 cards). Mice were only sighted at stations that were opposite pasture habitat, where ship rats were significantly less abundant. Four of the five tunnels tracked by mice at the base of the fence were also opposite pasture habitat.
Internal fencing
Neither the cameras nor the tracking cards within the hood recorded mouse activity along the Southern Enclosure fence across 132 sampling nights; however, six out of 15 (40%) tracking tunnels placed at the base of the fence were tracked by mice. Two of the tunnels were only tracked once, while the remaining four were tracked repeatedly. Tunnels placed at the base of the Southern Enclosure fence were not tracked at a higher rate than tunnels at the perimeter fence base (X2 = 0.55, df = 1, p = 0.46).
Discussion
Predation cues to deter rodents
Light
Our hypothesis that rodents would avoid illumination to reduce their predation risk was not supported. While Farnworth et al. (Citation2019) found that captive wild ship rats significantly reduced visitation rates (by 53%), exploration (by 70%) and movement (40% slower) under illumination, in the current study the number of ship rats recorded at lit stations did not differ from unlit stations.
However, the size of the illuminated area that rodents must travel through may influence their perception of risk and their foraging behaviour. For example, light only extended across a small area within the hood of Maungatautari’s pest-fencing (0.028 m2), compared to Farnworth et al.’s (Citation2019) much larger 2.3 m2 illuminated area. At Maungatautari, rats could rapidly (under 5 sec) travel through the lit section of the hood, and such a brief exposure may not have elevated rodents’ perception of predation risk enough to deter their movement. Furthermore, exiting the hood to avoid light would expose rats to predators which frequently patrol the fence perimeter. For example, Connolly et al. (Citation2009) reported 116 sightings of cats in summer and 80 in winter at Maungatautari. Ship rats may have remained within the fence hood because exposure to light (an indirect predation cue) was less risky than encountering predators (a direct predation cue) at the base of the fence. Remaining within the hood may also offer improved foraging opportunities for ship rats. Invertebrates, such as weta (Auckland tree weta, Hemideina thoracica, and cave weta, Rhaphidophoridae spp.), are an abundant source of food within the fence gutter at Maungatautari (Farnworth et al. Citation2018).
Light may still reduce the use of pest fencing by house mice, as suggested by Farnworth et al. (Citation2016), but with only four observations of mice using the gutter throughout our study period (all in the absence of light), we are unable to provide strong evidence that light deters mice.
Habitat
In December, when all 15 stations were opposite pasture, we recorded little rat activity. Consequently, in January, we shifted seven stations to sites opposite forest habitat where rats were more abundant than at the eight stations left at their original sites opposite pasture (January sightings at stations opposite pasture = 9; January sightings at stations opposite forest = 29). The unanticipated changes to our study allowed us to analyse how the habitat type opposite pest fencing influences rodent presence within the hood. While earlier research indicated that the habitat opposite pest fencing did not influence fence use by rats and mice (Connolly et al. Citation2009), our results produced a novel finding: stations opposite forest habitat recorded significantly more rodent visits than those opposite pasture (visits at forest sites = 29; visits at pasture sites = 13).
It is widely accepted that forest habitats support abundant food sources for ship rats (Cox et al. Citation2000; Innes Citation2005) and enable a broader range of nesting sites (Cox et al. Citation2000). At our sites, ship rat abundance may have been greater at forest sites due to the availability of resources. However microhabitat structure is also considered an indirect predation cue, that prey animals use to determine risk (Thorson et al. Citation1998). Other rodent species perceive bare, open habitat (e.g. pasture) to be riskier than complex habitat (i.e. forest) (Morris and Davidson Citation2000), as dense vegetation reduces visual detection by predators and physically obstructs predator attacks (Jensen et al. Citation2003; Fanson et al. Citation2008). Therefore ship rats may exploit the protection offered by canopy cover and travel along sections of fencing opposite forest habitat more often than fencing adjacent to pasture habitat if they perceive less risk.
Rat presence near reserves may be minimised if future sanctuaries are designed to incorporate pest fencing along pasture or open habitat, and if managers of existing ecosanctuaries maintain open corridors opposite fences that surround sanctuaries. Pest management (i.e. traps or bait stations) within patches of forest adjacent to fenced sanctuaries may also minimise exploitation of the hood by local rat populations. The greater use of forest sites, where rats were most common, probably explains the increase in rat sightings in January, and we therefore believe the modifications we had to make to our sampling protocol explain the effect of month on rat presence within the fence hood (four visits in December versus 38 visits in January).
Interspecific interactions at pest fencing
We expected that ship rat competition and predation would exclude mice from external stations (Bridgman et al. Citation2013; Watts et al. Citation2017), but we captured four visits by mice on our trail cameras and collected one card tracked by mice from the hood of perimeter fencing. Detecting mice within the hood was surprising, as an earlier study by Connolly et al. (Citation2009) at Maungatautari indicated they travelled exclusively at the base of the fence. However, rat sightings were very low (total observations = 42) in our study compared to Connolly et al. (total observations = 187), despite our greater sampling effort (i.e. 131 vs. 28 sampling nights) and sampling during the same season (i.e. summer).
Fewer rats, especially opposite pasture habitat, may have allowed mice to increase their activity in areas they are usually restricted from accessing (Innes et al. Citation1995; Bridgman et al. Citation2013). However, we never observed mice within the hood of Maungatautari’s Southern Enclosure fence, despite the absence of rats there. Although mice are agile, they may only utilise the hood once they reach a certain size if entry and exit from the hood is physically challenging. Compared to ship rats (120–160 g; Innes Citation2005), house mice are much smaller (17–26 g; Ruscoe and Murphy Citation2005) and their size may impair their ability to climb along the fence into the hood. Although prior sampling in vegetation at Maungatautari found evidence of mice at heights that exceeded that of the fence (Kelly Citation2016; Watts et al. Citation2017; Innes et al. Citation2018), they tracked a larger percentage (93%) of devices on the ground, indicating a preference for travelling on the forest floor (Kelly Citation2016). Mice may only climb the fence to avoid predators that rely heavily on sound to detect prey (Buesching et al. Citation2008), as utilising the fence prevents them disturbing leaf litter, soil or twigs on the ground that could attract attention.
While we rarely observed mice travelling within the gutter of Maungatautari’s pest-fences, we found that mice regularly moved along the fence base: mice were present in tunnels below our stations within the sanctuary’s internal fencing and along external perimeter fencing. Additionally, mice tracked at 50% (12 out of 24) of baited tunnels placed at the base of the Southern Enclosure fence by Maungatautari staff in the first week (4 December 2015–11 December 2015; at the beginning of our study) and 33% (eight out of 24) of tunnels in the second week (11 December 2015–18 December 2015) of their monitoring period (A. Tairi, personal communication, 20 April 2016). Thus, despite being good climbers (Ruscoe and Murphy Citation2005; Innes et al. Citation2018), the risk of reinvasion by mice may be greater from structural damage occurring at the base of the fence, especially when considering other research showing that 86.6% of mice sighted entered a simulated fence breach at ground level (Connolly et al. Citation2009).
Travelling close to the fence base may allow mice to use it as a navigation tool during their nightly forays to locate shelter, mates or food. Wild rodents are innately thigmotactic (Stryjek and Modlinska Citation2013) and mice are naturally guided by upright structures (Horev et al. Citation2007). Remaining close to upright structures may reduce predation risk by minimising any shadows cast during movement, reducing available angles for predators to approach, or increasing the probability of locating crevices to use as a refuge (Jensen et al. Citation2003).
Recommendations for management and future research
The repeated use of the hood by ship rats in both this study and earlier research (i.e. Connolly et al. Citation2009) suggests that fencing erected for future pest management schemes should consider a different design profile to reduce rodent traffic. However, conservation managers may still benefit from the existing fencing by setting traps or poison on the lip on the inside of the hood (Innes et al. Citation2011).
We did not find strong avoidance responses among ship rats at illuminated sections of pest fencing; however, we support further field trials, as our small sample size and simple measure of avoidance may have failed to detect a more complicated response to light. Extending the area and length of the illuminated runway sections may also improve deterrence. Research into deterrents that keep sanctuaries free from rodents remains valuable, as rats and mice may place increased pressure on pest-fences after experiencing meso-predator release as New Zealand moves towards the 2050 vision of a ‘Predator Free New Zealand’ (Russell et al. Citation2015).
Concurring with other research (e.g. Cooper et al. Citation2017), we also noted that monitoring devices did not capture all rodent visits to our sites. There were five instances where tracking cards in the hood were marked but no camera observations were recorded. Our trail cameras were triggered by motion but also programmed to take pictures every five seconds with an infra-red flash. Both the infra-red flash and noise produced by Reconyx HC600 cameras falls within the detectable ranges of some nocturnal animals (Meek et al. Citation2014); these disturbances might cause rats to move stealthily, potentially explaining the missing image captures. Tracking cards were also imperfect as there were eight instances where cameras recorded images of ship rats that did not cross tracking cards placed in the hood. Certainly, future researchers should consider using a range of detection devices to maximise detection rates and information gained.
Acknowledgements
We wish to thank Maungatautari Ecological Island for their support, and we particularly acknowledge the Maungatautari volunteers who gave assistance with data collection. We also thank Neil Fitzgerald (Manaaki Whenua–Landcare Research) for help identifying rodent species and David Duganzich for advice regarding statistical analyses. We thank anonymous reviewers, Chris Jones, Peter Banks and Theresa Jones for suggestions that improved earlier versions of this manuscript and Andrea Graves for editing services. The Waikato University Animal Ethics Committee was consulted regarding all aspects of animal welfare in this study.
Disclosure statement
No potential conflict of interest was reported by the authors.
ORCID
Bridgette Farnworth http://orcid.org/0000-0002-2417-8968
Additional information
Funding
References
- Apfelbach R, Blanchard C, Blanchard RJ, Hayes RA, McGregor IS. 2005. The effects of predator odors in mammalian prey species: a review of field and laboratory studies. Neurosci Biobehav Rev. 29:1123–1144. doi: https://doi.org/10.1016/j.neubiorev.2005.05.005
- Armstrong DP, Raeburn EH, Lewis RM, Ravine D. 2006. Estimating the viability of a reintroduced New Zealand robin population as a function of predator control. J Wildl Manage. 70(4):1020–1027. doi: https://doi.org/10.2193/0022-541X(2006)70[1020:ETVOAR]2.0.CO;2
- Bridgman LJ, Innes J, Gillies C, Fitzgerald NB, Miller S, King CM. 2013. Do ship rats display predatory behaviour towards house mice? Anim Behav. 86(2):257–268. doi: https://doi.org/10.1016/j.anbehav.2013.05.013
- Buesching CD, Newman C, Twell R, Macdonald DW. 2008. Reasons for arboreality in wood mice Apodemus sylvaticus and bank voles Myodes glareolus. Mammalian Biology - Zeitschrift für Säugetierkunde. 73(4):318–324. doi: https://doi.org/10.1016/j.mambio.2007.09.009
- Burns B, Innes J, Day T. 2012. The use and potential of pest-proof fencing for ecosystem restoration and fauna conservation in New Zealand. In: Somers MJ, Hayward MW, editors. Fencing for conservation: restriction of evolutionary potential or a riposte to threatening processes? New York: Springer; p. 65–90.
- Caut S, Casanovas JG, Virgos E, Lozano J, Witmer GW, Courchamp F. 2007. Rats dying for mice: modelling the competitor release effect. Austral Ecol. 32(8):858–868. doi: https://doi.org/10.1111/j.1442-9993.2007.01770.x
- Clarke JA. 1983. Moonlight's influence on predator-prey interactions between short-eared owls (Asio flammeus) and deermice (Peromyscus maniculatus). Behav Ecol Sociobiol. 13(3):205–209. doi: https://doi.org/10.1007/BF00299924
- Clarkson B. 2002. Upland forests and special open habitats. In: Clarkson B, Merrett M, Downs T, editors. Botany of the Waikato. Hamilton, New Zealand: Waikato Botanical Society; p. 82–91.
- Connolly TA, Day TD, King CM. 2009. Estimating the potential for reinvasion by mammalian pests through pest-exclusion fencing. Wildl Res. 36(5):410–421. doi: https://doi.org/10.1071/WR09021
- Cooper A, Kelly CL, King CM, Miller SD, Patty B. 2017. Do rats mind getting their feet dirty? Observing the behaviour of ship rats (Rattus rattus) towards footprint tracking tunnels. N Z J Zool. 45(1):61–72. doi: https://doi.org/10.1080/03014223.2017.1361455
- Cox MPG, Dickman CR, Cox WG. 2000. Use of habitat by the black rat (Rattus rattus) at North Head, New South Wales: an observational and experimental study. Austral Ecol. 25(4):375–385. doi: https://doi.org/10.1046/j.1442-9993.2000.01050.x
- Day T, MacGibbon R. 2007. Multiple species exclusion fencing and technology for mainland sites. In: Witmer G, Pitt W, Fagerstone K, editors. Managing vertebrate invasive species symposium. National Wildlife Research Centre. Fort Collins: USDA/APHIS/WS; p. 418–433.
- Dice LR. 1945. Minimum intensities of illumination under which owls can find dead prey by sight. The American Naturalist. 79(784):385–416. doi: https://doi.org/10.1086/281276
- Fanson BG, Fanson KV, Brown JS. 2008. Foraging behaviour of two rodent species inhabiting a kopje (rocky outcrop) in Tsavo West National Park, Kenya. Afr Zool. 43(2):184–191. doi: https://doi.org/10.1080/15627020.2008.11657235
- Farnworth B, Innes J, Kelly C, Littler R, Waas JR. 2018. Photons and foraging: artificial light at night generates avoidance behaviour in male, but not female, New Zealand weta. Environ Pollut. 236:82–90. doi: https://doi.org/10.1016/j.envpol.2018.01.039
- Farnworth B, Innes J, Waas JR. 2016. Converting predation cues into conservation tools: the effect of light on mouse foraging behaviour. PLoS One. 11(1):e0145432. doi: https://doi.org/10.1371/journal.pone.0145432
- Farnworth B, Meitern R, Innes J, Waas JR. 2019. Increasing predation risk with light reduces speed, exploration and visit duration of invasive ship rats (Rattus rattus). Scientific Reports. 9(1):3739. doi: https://doi.org/10.1038/s41598-019-39711-3
- García J. 2002. Interaction between introduced rats and a frugivore bird-plant system in a relict island forest. J Nat Hist. 36(10):1247–1258. doi: https://doi.org/10.1080/00222930110039594
- Horev G, Benjamini Y, Sakov A, Golani I. 2007. Estimating wall guidance and attraction in mouse free locomotor behavior. Genes. Brain and Behav. 6(1):30–41.
- Innes J. 2005. Ship Rat. In: King CM, editor. The handbook of New Zealand Mammals. 2nd ed. New York: Oxford University Press; p. 187–203.
- Innes J, Fitzgerald N, Binny R, Byrom AE, Pech R, Watts C, Gillies C, Maitland M, Campbell-Hunt C, Burns B. 2019. New Zealand ecosanctuaries: types, attributes and outcomes. J R Soc N Z. doi:10.1080/03036758.03032019.01620297.
- Innes J, Hay R, Flux I, Bradfield P, Speed H, Jansen P. 1999. Successful recovery of North Island kokako Callaeas cinerea wilsoni populations, by adaptive management. Biol Conserv. 87(2):201–214. doi: https://doi.org/10.1016/S0006-3207(98)00053-6
- Innes J, Kelly C, Fitzgerald N, Warnock M, Waas J. 2018. Detection of wild house mice and other small mammals up trees and on the ground in New Zealand native forest. N Z J Zool. 45(3):227–237. doi: https://doi.org/10.1080/03014223.2018.1461660
- Innes J, Lee WG, Burns B, Campbell-Hunt C, Watts C, Phipps H, Stephens T. 2012. Role of predator-proof fences in restoring New Zealand's biodiversity: a response to Scofield et al. (2011). N Z J Ecol. 36(2):232–238.
- Innes J, Warburton B, Williams D, Speed H, Bradfield P. 1995. Large scale poisoning of ship rats (Rattus rattus) in indigenous forests of the North Island, New Zealand. N Z J Ecol. 19(1):5–17.
- Innes J, Watts C, Fitzgerald N, Thornburrow D, Burns B, Mackay J, Speedy C. 2011. Behaviour of invader ship rats experimentally released behind a pest-proof fence, Maungatautari, New Zealand. In: Veitch C, Clout M, Towns D, editors. Island invasives: eradication and management. Gland: IUCN; p. 437–440.
- Jensen SP, Gray SJ, Hurst JL. 2003. How does habitat structure affect activity and use of space among house mice? Anim Behav. 66:239–250. doi: https://doi.org/10.1006/anbe.2003.2184
- Kelly C. 2016. House mouse (Mus musculus) use of vertical space and impact on bird nesting success [Masters Unpublished thesis]. University of Waikato.
- Kramer KM, Birney EC. 2001. Effect of light intensity on activity patterns of patagonian leaf-eared mice, Phyllotis xanthopygus. J Mammal. 82(2):535–544. doi: https://doi.org/10.1644/1545-1542(2001)082<0535:EOLIOA>2.0.CO;2
- Krijger IM, Belmain SR, Singleton GR, Groot Koerkamp PWG, Meerburg BG. 2017. The need to implement the landscape of fear within rodent pest management strategies. Pest Manage Sci. 73(12):2397–2402. doi: https://doi.org/10.1002/ps.4626
- Long K, Robley A. 2004. Cost effective feral animal exclusion fencing for areas of high conservation value in Australia. Melbourne: Department of Sustainability and Environment.
- Meek PD, Ballard GA, Fleming PJS, Schaefer M, Williams W, Falzon G. 2014. Camera traps can be heard and seen by animals. Plos One. 9:10. doi: https://doi.org/10.1371/journal.pone.0110832
- Metzgar LH. 1967. An experimental comparison of screech owl predation on resident and transient white-footed mice (Peromyscus leucopus). J Mammal. 48(3):387–391. doi: https://doi.org/10.2307/1377771
- Morris DW, Davidson DL. 2000. Optimally foraging mice match patch use with habitat differences in fitness. Ecology. 81(8):2061–2066. doi: https://doi.org/10.1890/0012-9658(2000)081[2061:OFMMPU]2.0.CO;2
- Nersesian CL, Banks PB, McArthur C. 2012. Behavioural responses to indirect and direct predator cues by a mammalian herbivore, the common brushtail possum. Behav Ecol Sociobiol. 66(1):47–55. doi: https://doi.org/10.1007/s00265-011-1250-y
- Olson D, Farley L, Naisilisili W, Raikabula A, Prasad O, Atherton J, Morley C. 2006. Remote forest refugia for Fijian wildlife. Conserv Biol. 20(2):568–572. doi: https://doi.org/10.1111/j.1523-1739.2006.00412.x
- Orrock JL, Danielson BJ, Brinkerhoff RJ. 2004. Rodent foraging is affected by indirect, but not by direct, cues of predation risk. Behav Ecol. 15(3):433–437. doi: https://doi.org/10.1093/beheco/arh031
- Pech R, Maitland M. 2016. Conservation of native fauna in highly invaded systems: managing mammalian predators in New Zealand. Restor Ecol. 24(6):816–820. doi: https://doi.org/10.1111/rec.12376
- Prugh LR, Golden CD. 2014. Does moonlight increase predation risk? Meta-analysis reveals divergent responses of nocturnal mammals to lunar cycles. J Anim Ecol. 83:504–514. doi: https://doi.org/10.1111/1365-2656.12148
- Ruscoe WA, Murphy E. 2005. House mouse. In: King CM, editor. The handbook of New Zealand mammals. 2nd ed. Melbourne: Oxford University Press; p. 204–221.
- Russell JC, Innes JG, Brown PH, Byrom AE. 2015. Predator-free New Zealand: conservation country. Bioscience. 65(5):520–525. doi: https://doi.org/10.1093/biosci/biv012
- Schakner ZA, Blumstein DT. 2013. Behavioral biology of marine mammal deterrents: a review and prospectus. Biol Conserv. 167:380–389. doi: https://doi.org/10.1016/j.biocon.2013.08.024
- Scofield RP, Cullen R. 2012. Fenced sanctuaries need critical evaluation: a reply to Innes et al. (2012). N Z J Ecol. 36(2):239–242.
- Scofield RP, Cullen R, Wang M. 2011. Are predator-proof fences the answer to New Zealand's terrestrial faunal biodiversity crisis?. N Z J Ecol. 35(3):312–317.
- Shapira I, Walker E, Brunton DH, Raubenheimer D. 2013. Responses to direct versus indirect cues of predation and competition in naive invasive mice: implications for management. N Z J Ecol. 37(1):33–40.
- Speedy C, Day T, Innes J. 2007. Pest eradication technology - the critical partner to pest exclusion technology: the Maungatautari experience. In: Witmer G, Pitt W, Fagerstone K, editors. Managing vertebrate invasive species: proceedings of an international symposium. Fort Collins: USDA/APHIS/WS: National Wildlife Research Centre; p. 115–126.
- Stryjek R, Modlinska K. 2013. A thigmotaxis-based method of recapturing and transporting small mammals in the laboratory. Lab Animal. 42:321–324. doi: https://doi.org/10.1038/laban.328
- Thorson JM, Morgan RA, Brown JS, Norman JE. 1998. Direct and indirect cues of predatory risk and patch use by fox squirrels and thirteen-lined ground squirrels. Behav Ecol. 9(2):151–157. doi: https://doi.org/10.1093/beheco/9.2.151
- Towns DR, Daugherty CH. 1994. Patterns of range contractions and extinctions in the New Zealand herpetofauna following human colonisation. N Z J Zool. 21(4):325–339. doi: https://doi.org/10.1080/03014223.1994.9518003
- Watts C, Armstrong D, Innes J, Thornburrow D. 2011. Dramatic increases in weta (Orthoptera) following mammal eradication on Maungatautari - evidence from pitfalls and tracking tunnels. N Z J Ecol. 35(3):261–272.
- Watts C, Innes J, Wilson D, Fitzgerald N, Bartlam S, Thornburrow D, Smale M. 2017. Impacts of mice alone on biodiversity: final report of a Waikato field trial. Hamilton, New Zealand: Landcare Research Contract Report Number LC2747.
- Young LC, VanderWerf EA, Lohr MT, Miller CJ, Titmus AJ, Peters D, Wilson L. 2013. Multi-species predator eradication within a predator-proof fence at Ka‘ena Point, Hawai‘i. Biol Invasions. 15(12):2627–2638. doi: https://doi.org/10.1007/s10530-013-0479-y