ABSTRACT
The New Zealand fairy tern/tara iti (Sternula nereis davisae; NZFT) is New Zealand’s most endangered bird, with approximately 40 individuals remaining. Due to previous poor breeding success, this species is on the brink of extinction. Nest failures occur because of adverse weather, predation, and potentially nest disturbance. We observed parental behaviour of 11 nesting pairs from November 2020 to January 2021 at three sites: Mangawhai, Waipu and Pakiri. Overall, avian predator disturbance was low at all nests, and most disturbance events were by interactions between conspecifics. Responses to conspecific disturbances were lowest for the three closely nesting pairs and highest for the three solitary nests. We measured the frequency of chick feeding and found feeding rates varied considerably between nests. Defensive response rates and feeding rates varied between nests but we found no relationship between the proportion of responses to disturbance and feeding rates. We found no relationship between feeding rate and tidal state. This could be due to our small sample size and the duration of our observation period (30 min). We urge future researchers to increase observation period time. With an understanding of feeding rates and disturbance, informed decisions can be made to support the conservation of NZFT.
Waitara
Ko te Tara iti/New Zealand fairy tern (Sternula nereis davisae; NZFT), te momo manu morearea rawa atu o Aotearoa, tata ki te 40 e toe ana nga tangata takitahi me te 11 nga takirua whakatipu.
…
Introduction
Almost one-third of the world's seabird species (96 of 304 species) are classified as threatened with extinction (Birdlife International Citation2023). The number of endangered or critically endangered seabird species has increased from 12% to 17% over the last 10 years (Croxall et al. Citation2012; Birdlife International Citation2023). Even among the Laridae, a group that is generally thriving, 14% of its species are threatened with extinction (Birdlife International Citation2023). Reviews and meta-analyses by Dias et al. (Citation2019) and Grant et al. (Citation2022), respectively, have identified the key threats to seabirds as climate change, invasive predators, declines in food resources, loss of nesting habitat, and human disturbance. Smaller seabirds, such as fairy terns (Sternula nereis) that nest in accessible coastal areas, are particularly vulnerable to invasive predators (Greenwell et al. Citation2019). The threats to New Zealand seabirds do not differ to those affecting seabirds around the world; however, the evolutionary absence of terrestrial mammals and the subsequent introductions of many invasive mammalian predator species have increased the impact of invasive predators on many seabird populations in New Zealand.
The New Zealand fairy tern/tara iti (Sternula nereis davisae; NZFT) is one of three distinct and geographically isolated subspecies of fairy tern that also include the Australian fairy tern (S. n. nereis) and New Caledonian fairy tern (S. n. exsul) (Baling and Brunton Citation2022). All three subspecies are in decline, but the NZFT has the smallest single population comprised of approximately 40 birds (BirdLife International Citation2018). The population has experienced variable, but typically low, breeding success since management began in 1983 (Ferreira et al. Citation2005). In this study we recorded observations on the number and type of disturbances at nest sites and the defensive responses of breeding birds to these disturbances and on the feeding rate of chicks at different ages from November 2020 to January 2021 at three breeding sites: Mangawhai, Waipu and Pakiri.
Disturbance at nest sites can occur in various forms, including conspecifics, interspecifics, avian predators and human activities. Disturbance at breeding sites is a significant threat for many coastal, ground nesting seabirds (Syrova et al. Citation2020; Byerly et al. Citation2022). Although responses to different disturbances often vary in intensity and frequency, diverting time and energy away from activities such as chick feeding can have negative effects on breeding success. For example, avian predator disturbance was negatively associated with breeding success in least tern (S. antillarum) colony productivity. This disturbance likely contributed to decreased productivity as adults attending chicks were regularly flushed. Human disturbance had a similar impact on breeding success (Darrah Citation2020). Similarly, common tern (S. hirundo) and subspecies of fairy tern will respond to disturbances during nesting (Palestis Citation2005; Baling Citation2008). Here we define these responses as defensive behaviour (Greenwell Citation2021). However, little is known about the defensive responses to disturbance for NZFT.
One potential driver of low NZFT population growth is chick feeding rate. NZFT chicks are solely dependent on their parents for food until they fledge at around 32 days old (Parrish and Pulham Citation1995). They then often maintain post-fledging dependency (Preddey Citation2008). The energetic requirement for chick growth pre-fledging means that pairs must maintain a high feeding rate for the chicks to survive.
Measuring the chick feeding rates of NZFT is likely to help conservation managers understand the relationship between feeding rates and breeding success for this species. It also enables managers to assess the need for intervention (e.g. supplemental feeding of chicks).
Materials and methods
Study setting
Data collection was carried out at three beach sand dune breeding sites: Mangawhai (36°06′S, 174°36′E), Waipu (35°59′S, 174°29′E) and Pakiri (36°15′S, 174°44′E). Papakanui (36°26′S, 174°12′E) is also a known NZFT breeding site; however, this was not logistically possible to include as part of our study. All breeding sites are subject to intensive trapping for mammalian predators. We observed breeding pairs of NZFT with nests. All birds had previously been banded by the New Zealand Department of Conservation (DOC) with unique colour combinations of leg bands allowing visual identification of all individuals and nests. Information of final breeding success during 2020/2021 was provided by DOC.
Nest observations
Observations of NZFT nests were made continuously during 30 min sampling periods. We noted the number and age of chicks and/or eggs. Overall, we made 156 observations (18 during incubation and 138 during chick rearing). Observation periods occurred between sunrise and sunset; therefore, nocturnal observations of nest disturbances and feeding activities were not included in our study. Observations included weekdays and weekends. Observations were made under favourable weather conditions. At Mangawhai and Waipu, observers were positioned a minimum of 30 m from the nest in hides provided by the Shorebirds Trust. At the Pakiri site, observers sat on a sand dune above the nest site a minimum of 30 m away. Observers used 10 × 45 binoculars and 50 mm spotting scopes with 12–36× magnification.
NZFT behaviours were categorised using published descriptions of NZFT behaviours (Parrish and Pulman Citation1995). Behaviours were recorded using the software BORIS (Friard and Gamba Citation2016) on laptops with preloaded behavioural descriptions. The observation site and tide for each observation was noted. Active NZFT fairy tern nests were continuously observed for 30 min.
Disturbance at nest
Every 5 min the nesting area within 30 m of the nesting bird was scanned for potential sources of disturbance. This distance was selected to be the same as observer distance as any disturbance outside of it did not cause a detectable behavioural change in NZFT. Disturbances were categorised as either conspecifics, interspecifics, avian predators, or humans. Conspecifics were defined as other NZFT individuals that were not directly associated with the nest being observed (non-chick or parent birds). Interspecifics was defined as other seabird and shorebird species such as Caspian tern/taranui (Hydroprogne caspia) and variable oystercatcher/tōrea pango (Haematopus unicolor) that potentially compete with NZFT for space. Note that while instances of these species attacking the chicks and eggs of NZFT have been recorded, such events are relatively infrequent and likely driven by territorial aggression rather than predation. Avian predators were species that are known to predate chicks and eggs, such as black-backed gull/karoro (Larus dominicanus). Mammalian predators of NZFT are primarily nocturnal, hence not included in our observations or analyses. Human disturbance included, but was not limited to, dogs and walkers. For each observed disturbance a comment describing the disturbance were recorded. Nests were categorised as group or solitary. Group nests are active NZFT nest within 10 m of another. Solitary nests are active NZFT nest greater than 10 m away from another.
Here we report on defensive behavioural responses. Defensive behaviours were defined as adults chasing other birds or intruders. Instances of nest defence toward disturbances were summed for the four categories of disturbance (conspecific, interspecific, avian predator, human) and reported as the total number of disturbances recorded in that category. These totals were then divided by the total number of encounters in their corresponding category to produce a proportion of defensive response across nests.
Feeding rate
Feeding rates of NZFT chicks prior to fledging were recorded in 30 min time periods. Data were analysed from the six different nests. Overall, there were 138 observation bouts where parents had chicks and feeding could occur and a total of 181 feeding events were recorded. During each observation period, BORIS (Friard and Gamba Citation2016) was used to record each feeding event. A successful feeding event was defined as the chick successfully swallowing a fish brought back to the nest site by a parent. The resulting information was then extracted from BORIS and used to estimate the average feeding rate of chicks from each nest per 30 min period. The feeding rate data were averaged across three-day chick age periods at 0–2 days, 3–5 days, etc. We report the feeding rate per chick in the nest to enable comparison of single and two chick nests.
To test for any relationship between feeding rates and defensive response we used the feeding rate categories and pooled defensive responses (0–1, 2–3, 4+), before performing a Chi-squared test of independence. To test for the effects of tidal state (low, mid, high) on feeding rates, we first pooled the feeding rates into three categories (0, 1–2, 3+). We then performed a Chi-squared test of independence. All statistical analyses were performed using R Studio (RStudio Team Citation2020). Because our sample size was small, consisting of only three successful nests (chicks surviving >32 days) and three failed nests, and considering that the failed nests occurred early in the nestling stage, we were unable to conduct meaningful analyses to determine the relationship between feeding rates and the probability of fledgling success.
Results
Summary of the 2020/21 breeding season
The 11 NZFT pairs breeding in 2020/21 made 17 nesting attempts from which came 29 eggs and 12 hatchlings. Three nests were successful and a total of four chicks fledged. Of the successful fledglings one came from a nest at Mangawhai (Nest ID: M442), two together from a nest in Waipu (W435), and one from a nest at Pakiri (P440). The main cause of failure otherwise was extreme weather such as high wind and rainfall, resulting in the loss of nests through flooding or abandonment. Assumed predation resulted in the loss of two eggs. One attack by an interspecific resulted in the loss of a hatchling. One instance of a chick choking, and subsequent mortality occurred.
Disturbance at nests
Counts of conspecifics per 30 min was highest at nests M430 (number of observation periods (obs.) = 8, average count/per 30mins (x̅) = 2.96), M433 (obs. = 6, x̅ = 2.95) and M437 (obs. = 11, x̅ = 3.15) (). These nests were grouped close together (approx. 5 m apart) at the Mangawhai breeding site. A fourth nest M442 had moderate conspecific presence (obs. = 59, x̅ = 1.2) (). This nest was active at the Mangawhai breeding site after the first three nests had failed. Nests W435 (obs. = 23, x̅ = 0.025) and P440 (obs. = 50, x̅ = 0.5) had considerably lower conspecific counts (). The counts of interspecifics per 30 min at nest sites varied considerably with the highest average recorded at nest P440 (x̅ = 13.89) (). All Mangawhai nests had comparably low counts of interspecifics (). Avian predator presence per 30 min was consistently low across all nesting sites (x̅ = 0.047) (). Human presence per 30 min were relatively low at all nests except P440 (x̅ = 7.94) ().
Figure 1. Left column: Average (+SE) nest disturbance count of A, conspecifics, C, interspecifics, E, avian predators and G, humans recorded within 30 m of each nest. Right column: Proportion (+SE) of defensive response by NZFT from each nest towards disturbance type, B, conspecifics, D, interspecifics, F, avian predators and H, humans over a 30 min period. Nests have been indicated by colour.
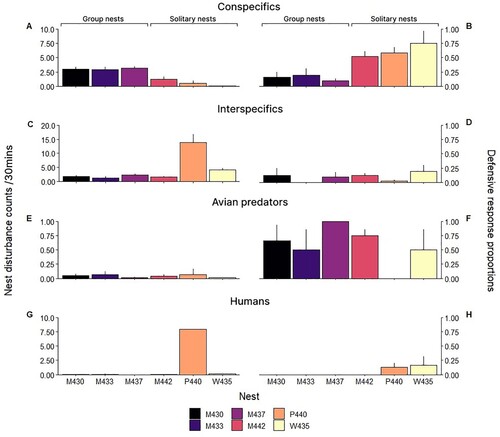
Proportion of defensive responses to an observed conspecific was highest at non-colonial nests W435, P440 and M442 (). Across all nests, the average levels of defensive response towards interspecifics were less than 20% of the time an interspecific was present (). Among the interspecific species observed, the New Zealand dotterels/tūturiwhatu (Charadrius obscurus) and variable oystercatchers were found to elicit the highest number of defensive responses. This is likely because they were the most common interspecific species across all of the nest sites. At the Waipu site the main interspecific disturbance was by welcome swallows/warou (Hirundo neoxena neoxena). Welcome swallows were also observed chasing NZFT at Mangawhai foraging sites. Defensive responses to avian predators were higher than responses to all other threats at each nest sites other than P440 (a solo parent) (). However, the sample sizes were low due to infrequent avian predator disturbance, so should be interpreted with caution. Defensive response to human disturbance was only recorded at nests P440 and W435. This also should be interpreted with caution as the sample size was generally low due to infrequent human disturbance.
Feeding rates
Feeding rates were measured for six nesting attempts, three successfully fledged chicks and three had chick mortality. Sample size varied between age and nest (). The low sample size for nests and ages should be considered when interpreting the results. Feeding rates varied considerably between nests and it was not possible to determine an overall pattern although most nests had low feeding rates when chicks were less than six days old ().
Figure 2. Left column: Nests which had chick(s) that died and did not fledge A, M430, C, M433 and E, M437. Right column: Nests which had chicks that did fledge (chicks surviving >32 days) B, M442, D, P440, F, W435. Y axis: average number of fish brought back to the nest and consumed by the chick (+SE). X axis: age (in days) of the chick, grouped into three-day periods (0–2, 3–5, 6–8, etc.), using the older age of the two chicks if more than one is present in the nest. Feeding rates per chick in the nest are reported to enable comparison of single and two chick nests. Counts were made in continuous observations over a 30 min period. All chicks that shared a nest hatched a day apart. Semi-successful and successful nests (where chick(s) fledged) are represented with a circle. Chicks that died before reaching 10 days old are represented with a triangle. Nest P440 became a solo parent nest when the chick was five days old. Nest W435 had two chicks. Nest M442 had two chicks until the younger of the two died at 17 days old. Nest M433 had two chicks that both died at 7/6 days old. Observations for nest W435 began when the chicks were 12/11 days old until the chicks fledged. Observations for nest M442 ended when the chick was 24 days old, prior to the chick's fledging. Observations for nest P440 were made until fledging. The nests are identified by colour, consistent with .
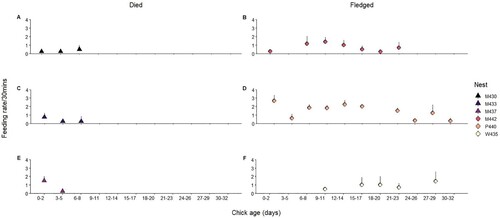
Table 1. The number of 30-minute observation periods (obs.) for six nesting attempts by breeding NZFT pairs in relation to chick age (in three-day periods).
From our analysis of the relationship between feeding rates and defensive response rates we did not find any relationship (χ2 = 4.8, df = 4, p = 0.313). We also found no significant relationship between categories of feeding rates and tide state (χ2 = 6.19, df = 4, p = 0.185).
Discussion
2020/21 Breeding season
The success of the 2020/2021 NZFT breeding season was within the range of those reported by Ferreira et al. (Citation2005) from 1991–2003, and like that of the 2021/22 season. Though for both recent seasons the number of successful fledglings was below average. This low productivity impacted our sample sizes.
Disturbance at nests
Despite comparatively high average conspecific disturbances observed at the early, closely grouped Mangawhai nests M430, M433 and M437, defensive responses to conspecifics at these nests were low compared to solitary nests (). While acknowledging that our sample sizes are unavoidably small for this critically endangered species, this result leads us to speculate that nesting NZFT may be strategically responding to different threats according to the context of the situation as reported for Australian and New Caledonian fairy terns (Baling Citation2008). Indeed, conspecifics incubating or brooding 5–10 m away from the nest appeared significantly less of a threat than non-nesting birds visiting the nesting site. Such risk assessments may be a crucial part of nest defence (Caro Citation2005) and is not unwarranted given that single male NZFT were observed pecking chicks while the parents were out foraging. In contrast, the number of avian predator disturbances that resulted in defensive responses by the NZFT were high regardless of group nesting or solitary nesting status (). Defence response towards interspecifics was low at all sites regardless of the number of encounters (). These interspecifics were often also nesting birds that appeared to be tolerated. This lack of defensive response may be due to low threat of these disturbances and little competition for nest sites within the large duneland system. Overall, we saw low human disturbance and few defensive responses to such disturbances across all nests (). In part this reflects the level of proactive management undertaken by DOC and the other community conservation volunteers at the NZFT breeding sites. These measures include signposting, fencing off areas to restrict access and nest watches. Additionally, studies on Arctic terns (S. paradisaea) found colonies in urban areas were more resilient to disturbance through necessity or acclimatisation (Syrova et al. Citation2020), this may be a factor driving low response rates to human disturbance at the Pakiri nesting site (). Human disturbance, much like interspecific disturbance at this nest, may be tolerated. Anthropomorphic change has forced birds to abandon breeding habitats such as in little terns (S. albifrons) (Medeiros et al. Citation2012); however, this species does not show the fidelity to foraging territory (and therefore breeding habitat) that is observed in NZFT (Ismar et al. Citation2014).
Understanding behavioural response to disturbance is important to conservation when combined with repercussions of defensive response to disturbance and time away from other activities. For NZFT we found feeding rates were not influenced by the level of defensive behaviour, but this may in part be due to the low overall levels of disturbance at the breeding sites resulting from management of threats and due to the sampling period we used in our study design. Should one type of disturbance incite responses from NZFT that do impact feeding behaviour, then conservation managers may need to consider possible interventions. Interventions aimed at removing humans and their activities is ongoing from areas where NZFT breed, and important evidence supports this approach. For example, Medeiros et al. (Citation2007) showed that by preventing human activities in certain areas, the impacts of human disturbance on little terns can be reduced. Where researchers noticed that avian predators would often incite defensive responses they attempted to control them (Donehower et al. Citation2007). Similarly, Morris et al. (Citation1980) found that removing nesting ring-billed gulls (L. delawarensis) led to a significant increase in the breeding success of common terns, highlighting the potential threats posed from an overcrowded habitat for terns should number of defensive responses go up as a result.
Feeding rates
Newly hatched chicks can only rely on yolk sacs for the first few days after hatching (Romanoff Citation1944; van der Wagt et al. Citation2020) and as in all seabirds, terns are semi-precocial and they quickly come to rely on their parents for food (Székely and Reynolds Citation1995; Thomas and Székely Citation2005; Preddey Citation2008). Because of the small size of the NZFT, young chicks can only consume small fish, thus constraining the food items that the parent must find for chick feeding and increasing the number of feeds required. NZFT do not always provide suitable fish for their chicks. For example, the two chicks at M442 (eight and seven days old) were observed being fed flounder (Rhombosolea sp.) that were too large to be eaten.
For the three monitored nests where chicks were successfully fledged, feeding rates for the first six days were relatively low compared to older stages (). These fluctuations, although observed for a small sample size, may be best explained by variation in chick energy associated with different growth patterns (Davies Citation1981). This difference in feeding rates will also depend on the number of chicks being raised, as seen in other tern species, but we had too few samples to detect a difference. In common terns and Arctic terns, frequency of food delivery was found to increase with each additional chick being brooded (Robinson and Hamer Citation2000). Finally, Sherfy et al. (Citation2012) found that radio-tagged least terns (Sternula antillarum) occasionally fly at night, but the amount of foraging undertaken was not known. Nighttime foraging by NZFT could be explored in future research.
Surprisingly, we found no evidence that variation in feeding rates was associated with the rates of defensive behaviour exhibited by parents in response to disturbance or with tidal state. Our 30 min observation period for sampling may have resulted in insufficient observations of feeding to conclusively determine the effect of tidal state and disturbance. Future studies that significantly increase observation periods are needed. The feeding rates at nests varied significantly, and longer observation rates may reduce the effect of this variation. For example, during our observation of nest P440, when the chick was five days old, the female parent disappeared and did not return. As a result, the feeding of the chick stopped during our sampling on that day. However, this impact was temporary, as the male parent the next day increased his time feeding the chick to compensate for the loss of his partner.
Finally, as we were unable to monitor chick growth through regular weighing, feeding rates are our best source of information to monitor chick health and level of parental care. Any early indication that a chick may not be receiving adequate food can help inform timely conservation interventions such as supplemental feeding. As only three of our monitored nests fledged chicks (W435, P440, M442), we are unable to confidently determine the expected feeding rates of an average NZFT chick. However, in line with our observations, we recommend that as part of a measure for breeding success, chicks should be monitored to ensure that feeding rates follow a pattern of increasing after the chicks reach six days old.
Conclusion
In conclusion, we observed low defensive responses to conspecifics at group nests compared to solitary nests and consistently low defence response towards interspecifics at all sites regardless of number of encounters. We observed consistently high defensive behaviour towards avian predators. Human disturbance was low and incited little defence response but must be taken into consideration. Although we did not detect a relationship between feeding rates and tidal state or defensive behaviours, expanded sampling is needed to better understand chick feeding patterns of the NZFT. It is critical that the drivers and outcomes of variations in feeding rates of chicks be better understood.
Acknowledgements
We thank The Shorebirds Trust and the Tara iti Golf Course for providing accommodation throughout the duration of our field work and, Linda Guzik, Alex Flavell-Johnson and Rob Channing, from the Tara iti Golf Course, for onsite support. This project was conducted with the support and permission of the Department of Conservation. We thank DOC Rangers Shannan Courtenay, Jacob Ball, Nikki Hartley and Amanda Hunt, and volunteers.
Disclosure statement
No potential conflict of interest was reported by the author(s).
Additional information
Funding
References
- Baling M. 2008. Conservation of the Fairy Tern (Sternula nereis spp.) via subspecies level management. Endangered Species Update. 25(3):86–93.
- Baling M, Brunton DH. 2022. Structured phylogeography and restricted gene flow among populations of Fairy Tern (Sternula nereis) across Australasia: implications for the endangered New Zealand population. Ibis. 164:800–808. https://doi -org .ezproxy.massey.ac.nz/10 .1111/ibi.13048.
- BirdLife International. 2018. Sternula nereis. The IUCN Red List of Threatened Species 2018: e.T22694691A132568135. doi:10.2305/IUCN.UK.2018-2.RLTS.T22694691A132568135.en.
- BirdLife International. 2023. Species dashboard: seabirds and laridiae. Downloaded from http://datazone.birdlife.org/ on 20/07/2023.
- Byerly PA, Zaluski S, Nellis D, Leberg PL. 2022. Effects of colony disturbance on reproductive success and nest defence behaviours in Caribbean roseate terns. Waterbirds. 44(4):463–471.
- Caro TM. 2005. Antipredator defenses in birds and mammals. Chicago: University of Chicago Press.
- Croxall J, Butchart S, Lascelles B, Stattersfield A, Sullivan B, Symes A, Taylor P. 2012. Seabird conservation status, threats and priority actions: A global assessment. Bird Conservation International. 22(1):1–34. doi:10.1017/S0959270912000020.
- Darrah AJ. 2020. Mixed evidence for effects of stewardship on least tern reproductive success in coastal Mississippi. Ornithological Applications. 122(4):1–13. doi:10.1093/condor/duaa050.
- Davies S. 1981. Development and behaviour of little tern chicks. British Birds. 74:291–298.
- Dias MP, Martin R, Pearmain EJ, Burfield IJ, Small C, Phillips RA, Croxall JP. 2019. Threats to seabirds: a global assessment. Biological Conservation. 237:525–537. doi:10.1016/j.biocon.2019.06.033.
- Donehower CE, Bird DM, Hall CS, Kress SW. 2007. Effects of gull predation and predator control on tern nesting success at eastern egg rock, Maine. Waterbirds: The International Journal of Waterbird Biology. 30(1):29–39.
- Ferreira SM, Hansen KM, Parrish GR, Pierce RJ, Pulham GA, Taylor S. 2005. Conservation of the endangered New Zealand fairy tern. Biological Conservation. 125(3):345–354. doi:10.1016/j.biocon.2005.04.015.
- Friard O, Gamba M. 2016. BORIS: A free, versatile open-source event-logging software for video/audio coding and live observations. Methods in Ecology and Evolution. 7:1325–1330. doi:10.1111/2041-210X.12584.
- Grant ML, Bond AL, Lavers JL. 2022. The influence of seabirds on their breeding, roosting, and nesting grounds: A systematic review and meta-analysis. Journal of Animal Ecology. 91(6):1266–1289. doi:10.1111/1365-2656.13699.
- Greenwell C. 2021. Inferred predation of fairy tern eggs by ruddy turnstones at Rottnest island, western Australia, an internationally significant shorebird site. Australian Field Ornithology. 38:115–117. doi:10.20938/afo38115117.
- Greenwell CN, Calver MC, Loneragan NR. 2019. Cat gets its tern: A case study of predation on a threatened coastal seabird. Animals. 9(7):445. doi:10.3390/ani9070445.
- Ismar SM, Trnski TO, Beauchamp T, Bury SJ, Wilson D, Kannemeyer R, Bellingham M, Baird K. 2014. Foraging ecology and choice of feeding habitat in the New Zealand fairy tern Sternula nereis davisae. Bird Conservation International. 24(1):72–87. doi:10.1017/S0959270913000312.
- Medeiros R, Ramos JA, Paiva VH, Almeida A, Pedro P, Antunes S. 2007. Signage reduces the impact of human disturbance on little tern nesting success in Portugal. Biological Conservation. 135(1):99–106. doi:10.1016/j.biocon.2006.10.001.
- Medeiros R, Ramos JA, Pedro P, Thomas RJ. 2012. Reproductive consequences of nest site selection by little terns breeding on sandy beaches. Waterbirds. 35(4):512–524. doi:10.1675/063.035.0402.
- Morris RD, Kirkham IR, Chardine JW. 1980. Management of a declining common tern colony. Journal of Wildlife Management. 44:241–245. doi:10.2307/3808378.
- Palestis BG. 2005. Nesting stage and nest defense by common terns. Waterbirds. 28(1):87–94. doi:10.1675/1524-4695(2005)028[0087:NSANDB]2.0.CO;2.
- Parrish GR, Pulman GA. 1995. Observations on the breeding of the New Zealand fairy tern. Tane. 35:161–173.
- Preddey M. 2008. Post-fledging parental care of a juvenile New Zealand fairy tern (Sterna nereis davisae). Notornis. 55(3):159–161.
- Robinson JA, Hamer KC. 2000. Brood size and food provisioning in common terns Sterna hirundo, and Arctic terns S. paradisaea: consequences for chick growth. Ardea. 88(1):51–60.
- Romanoff A. 1944. Avian spare yolk and its assimilation. The Auk. 61(2):235–241. doi:10.2307/4079367.
- RStudio Team. 2020. RStudio: Integrated Development for R. RStudio, PBC, Boston, MA. http://www.rstudio.com/.
- Sherfy MH, Anteau MJ, Shaffer TL, Sovada MA, Stucker JH. 2012. Foraging ecology of least terns and piping plovers nesting on Central Platte River sandpits and sandbars. US Department of the Interior, US Geological Survey.
- Syrova M, Hromadkova T, Pavel V, Vesely P. 2020. Responses of nesting Arctic terns (Sterna paradisaea) to disturbance by humans. Polar Biology. 43:399–407. doi:10.1007/s00300-020-02641-2.
- Székely T, Reynolds JD. 1995. Evolutionary transitions in parental care in shorebirds. Proceedings: Biological Sciences. 262(1363):57–64. doi:10.1098/rspb.1995.0176.
- Thomas GH, Székely T. 2005. Evolutionary pathways in shorebird breeding systems: sexual conflict, parental care, and chick development. Evolution. 59(10):2222–2230.
- van der Wagt I, de Jong IC, Mitchell MA, Molenaar R, van den Brand H. 2020. A review on yolk sac utilization in poultry. Poultry Science. 99(4):2162–2175. doi:10.1016/j.psj.2019.11.041.