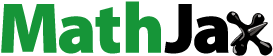
ABSTRACT
Neophilic tendencies in scavenging species living near humans may have adverse consequences for these animals. New Zealand’s kea (Nestor notabilis), an endangered parrot, potentially faces exacerbated mortality risk associated with proximity to humans. Hazards include lead building materials, introduced mammalian predators, and poison baits used to control these predators. How kea respond to these hazards can affect life expectancy. We modelled the effects of (1) proximity to humans, (2) age, (3) presence of conspecifics, and (4) blood lead levels, on the time taken to approach and contact a novel object. We also compared dietary trophic level between populations adjacent to and remote from humans using stable isotopes, and compared the age structure of these populations. Adjacent kea contacted the object faster than remote kea, as did younger individuals and those with elevated blood lead concentration, but conspecific presence did not affect approach latency. We conclude that adjacent kea exhibit enhanced neophilia, which may translate to reduced life expectancy due to interacting with anthropogenic hazards. Kea adjacent to humans were younger and foraged at a lower trophic level than remote kea, with a possible consequence of reduced productivity. Our results should be considered when setting management policy for kea habitat.
Introduction
When confronted with novelty, an individual must evaluate the conflicting forces of compulsion to investigate a potentially usable resource, and compulsion to avoid a potentially harmful hazard, such as poisoning or exposure to predators (Brown et al. Citation2013). Innate behavioural reactions to novelty include a combination of neophilia (compulsion to investigate) and neophobia (compulsion to avoid) (Berlyne Citation1950; Montgomery Citation1955). These responses enable an animal to learn what to seek, ignore, or avoid while foraging (Greenberg and Mettke-Hofmann Citation2001).
Despite appearing as a continuum, neophilia and neophobia are considered to be two independent motivational systems (Pearce and Russell Citation1971; Powell et al. Citation2003; Weisstaub et al. Citation2006) that operate concurrently (Greenberg and Mettke-Hofmann Citation2001): an animal can exhibit exploratory behaviour toward a novel object (i.e. neophilia), while remaining wary of potential hazards (i.e. neophobia). The balance between the two systems within an individual animal is influenced primarily by evolutionary ecology (including foraging behaviour), but this can be modified by experience and life stage (Sol et al. Citation2011; Greggor et al. Citation2015).
Evolution in environments with low predation pressure and a wide range of food types and feeding opportunities, as well as moderate levels of environmental stochasticity, tends to favour high neophilia, while very stable or highly stochastic environments favour low neophilia (Mettke-Hofmann et al. Citation2002; Griffin et al. Citation2017). Under extreme stability there is little to be gained from exploring an invariant environment, while in rapidly changing environments the information gained is of little predictive use (Winkler and Leisler Citation1999). For example, parrots inhabiting relatively complex environments like forest edges or islands exhibit a higher neophilia than those in more homogenous environments (Mettke-Hofmann et al. Citation2002). For these reasons, neophilia can be favoured among ecological generalists that may need to explore more to find new food types than ecological specialists (Greenberg and Mettke-Hofmann Citation2001; Mettke-Hofmann et al. Citation2002).
While neophilia may be driven by the benefits of exploration for novel resources, neophobic tendencies may be driven by the costs of exploration, leading to a degree of tension between the two. Consequently, neophilic ecological generalists also tend to have well-developed neophobic compulsions – having approached the novelty, they then treat it with caution. Only after safety-testing procedures are complete, does the novel item or food become safe and familiar. This can be influenced through social learning: if conspecifics are present, an individual may determine that the novelty is safe to approach (Greenberg Citation2003). Additionally, neophilic tendencies in ecological generalists tend to wane with age. Innovation is generally the result of juvenile neophilia, and adults can learn a new behaviour by observing the juveniles that discovered it (Greenberg Citation2003).
Our study species, the kea (Nestor notabilis), is an endangered generalist parrot endemic to the South Island of New Zealand. Unlike its forest specialist relative, the kākā (N. meridionalis), kea forage in environments ranging from sea level to the subalpine, depending on season and conditions. Kea often forage in mixed groups (Diamond and Bond Citation1991) and have a non-linear social structure (Tebbich et al. Citation1996) which may influence which individuals gain access to a novel resource (e.g. Gajdon et al. Citation2004). With an omnivorous diet, including plant components such as berries, invertebrates, and scavenged meat, kea exploit novel or seasonal food sources as they become available (O'Donnell and Dilks Citation1994; Schwing Citation2010; Young et al. Citation2012; Greer et al. Citation2015a).
Kea are known for their often-destructive exploratory behaviour with human property. Some kea are attracted to areas of human activity, such as ski fields, villages and roads, where they investigate novel objects (often containing lead) and consume lead as well as anthropogenic foods that are made available both accidentally and deliberately (Gajdon et al. Citation2006). Their innate neophilia may be a product of their life history, adapted for environments with unpredictable weather, variable food availability and, in evolutionary terms, a geologically changeable landscape that may have selected for exploratory behaviour (Martini et al. Citation2021). As occurs in scavenging bears in Europe and North America (e.g. Kavčič et al. Citation2015), kea that ‘scrounge’ for human foods may exhibit differing behavioural patterns to those living in remote areas. While scrounging kea may become a nuisance for humans or be susceptible to health issues, the primary concern in a conservation context is the accidental ingestion of lead or the predisposition to consume ‘unnatural foods’, such as poison baits (Reid et al. Citation2012; Kemp et al. Citation2019).
In kea habitat, the poison sodium fluoroacetate (1080) is aerially distributed on a landscape scale to control invasive mammalian predators that threaten New Zealand’s endemic fauna (Elliott and Kemp Citation2016). 1080 is often chosen as the most cost-effective of the available tools for protecting New Zealand’s native wildlife (Elliott and Kemp Citation2016), including kea (Kemp et al. Citation2018). Nevertheless, their neophilic attributes, combined with their ground-dwelling habits, predisposes kea to encounter and investigate baits, which they will often readily consume (although they do develop conditioned taste aversion to baits with secondary repellents lasting for over 12 months; Nichols et al. Citation2020; McLean et al. Citation2022). Neophobic attributes may prevent poisoning in many cases, but not all, and mortality increases with proximity to humans (Kemp et al. Citation2019). A study of kea survival through 1080 operations indicates that populations <20 km to human settlements are more likely to consume a lethal dose of 1080 than populations >40 km away from human settlements (Kemp et al. Citation2019). Despite ongoing attempts to add elements to baits to make them less appealing to birds (e.g. repellents, colours, flavours; Orr-Walker et al. Citation2012; Weser and Ross Citation2013; Cowan and Crowell Citation2017; Nichols et al. Citation2020), kea continue to suffer non-target mortality from ingesting 1080, and, while the overall net effect of 1080 in terms of increased reproductive success may be positive (Kemp et al. Citation2019), public objection to any deaths of individual kea remains a challenge for the agencies undertaking aerial 1080 operations.
Our aim is to test the hypotheses that scrounging human foods may alter the innate tendencies of kea which may predispose them to investigate, and subsequently consume, novel foods, potentially including poison baits (Kemp et al. Citation2019). We used a latency-to-contact experiment for this purpose. In our model, we accounted for the effects of age on latency to approach the novel object, as well as the presence or absence of conspecifics, how remote from human settlements the birds were (adjacent or remote), and circulating blood lead levels. We also used stable isotope analysis (SIA), to examine high-level differences in foraging ecology, and age structure between adjacent and remote kea populations with the aim of elucidating whether specific groups of kea may be more susceptible to ingestion of novel items, such as poison baits.
Methods
Latency to contact experiment
For the purposes of our experiments, locations were defined as <500 m from a human settlement (adjacent), and >40 km from human settlements (remote), excluding all sites in between, based on kea movements being typically (but not solely) restricted to only a few km, from what little is known (Kennedy et al. Citation2015).
A ‘latency-to-contact’ experiment was conducted to quantify behavioural response towards a novel object with four covariates (1) location (adjacent versus remote); (2) presence of conspecifics (present versus not present); (3) age class (fledgling versus juvenile/subadult (which are often difficult to distinguish) versus adult); (4) blood lead concentration (BLC; μg/dl). Kea were presented with a set-up consisting of two hessian mats (90 cm × 90 cm) placed 5 m apart and tied or pegged to the ground (). Kea prefer to land and perch on prominent rocks and ridgelines with a clear escape route, and mats were placed on these features where possible. The setup consisted of two mats and blocks rather than one to reduce interference from conspecifics (however only one individual per a group was tested). A ‘zone’ extending 2.5 m beyond the mats was marked with sticks or pebbles to provide a visible border for observers. If a kea landed within 15 m of a mat, an observer would hold up two blue plastic blocks (measuring 10 cm × 2 cm × 1 cm) and place one in the middle of each mat. The hessian mats provided a uniform background on which to place the object, and also formed part of a snare with which kea that contacted the object were caught for blood sampling for the foraging study (described below). The observer placed the blocks on the mats in such a way as to be sure that the kea had seen them. If a kea entered the ‘zone’, a timer was started. Any kea that walked or flew away without entering the zone was determined to have declined to take part in the test, and were recorded for comparisons of participation rate relative to location and age class. For those kea that entered the zone and therefore took part in the test, the timer ran either until it left the zone or until the block was contacted. If a kea exited the zone without contacting the block and then re-entered (<10% of cases), the timer was stopped on exit and restarted upon re-entry and the cumulative time within the zone was recorded. For every kea that participated in the test, the total time within the zone was recorded, as was the contact outcome (1 = contacted object; 0 = no contact), and latency to contact. If a group of kea were present, only one individual was tested, chosen at random before the blocks were placed on the mats. No individual kea were tested more than once. All latency-to-contact experiments were conducted during summer months between 2019 and 2021.
Figure 1. Kea neophilia latency-to-contact experiment setup (not to scale) with blue plastic blocks placed in the centre of two 90 × 90 cm hessian mats placed 5 m apart. A 2.5 m ‘zone’ extends around the entire setup.
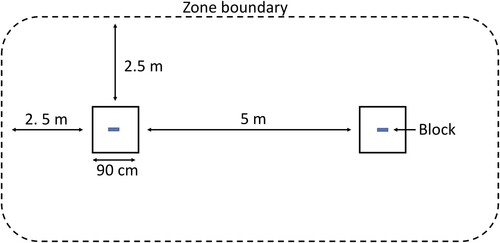
Kea were captured for blood sampling directly after finishing the experiment so only those kea that contacted the novel object were sampled, as it was not possible to capture those birds that had flown away before contacting the object. Not every kea that contacted the object was sampled for blood because the snare setup used for catching does not guarantee a successful catch. As such, the sample size of those tested for lead (N = 28) is less than the total number that contacted the object (N = 41). Blood samples were taken from the brachial wing vein using a 0.3 ml syringe, from which 50 μl was measured with a heparinised capillary tube into a vial containing dilute hydrochloric acid as provided by the blood lead analyser manufacturer (LeadCare® II Blood Lead Analyzer Model 70-6529, Magellan Diagnostics, MA, USA). Blood samples were processed according to manufacturer instructions within 72 h of collection. Whole red blood cells were lysed in HCl and transferred to the analyser for anodic stripping voltammetry, whereby an electrical potential causes lead to collect on a sensor. Due to the remote sampling locations, it was impossible to immediately process lead samples. Accuracy of delayed testing was verified by processing a subset of five samples immediately and keeping a second sample from the same individuals for 72 h before processing to ensure results remained the same (Kea Conservation Trust, unpublished data). The analyser had a maximum detectable BLC of 65 μg/dl and a minimum of 3.3 μg/dl.
Diet/foraging experiment
Blood for our foraging experiment was sampled separately from the latency-to-contact experiment and does not represent the same individuals as those tested in that experiment. Samples were collectd from throughout kea range () and were divided into adjacent and remote categories as in the latency-to-contact experiment. Blood turnover rate in birds is 2–4 weeks (Dalerum and Angerbjorn Citation2005) and therefore represents the recent diet of kea. About 0.1 ml of blood was stored in a labelled 0.5 ml plastic screw-cap vial that was approximately half-filled with 70% ethanol. Ethanol at this concentration acts as a preservative and does not affect carbon or nitrogen isotope values during analysis (Hobson et al. Citation1997). Blood samples were refrigerated at 4°C at the earliest possible time after returning from the field and were stored un-dried for a maximum of eight weeks before further preparation. Blood samples were dried by placing uncapped vials in a 60°C oven for 48 h and individually ground to a fine powder using a mortar and pestle. Between 0.5 and 0.7 mg of dried blood was weighed using a Mettler-Toledo UMX2 (Greifensee, Switzerland) ultra-microbalance accurate to 0.1 μg, and crimped into a 4 × 6 mm tin capsule for mass spectrometry.
Stable carbon and nitrogen isotopic compositions were determined by continuous flow combustion isotope ratio mass spectrometry. Prepared tin capsules of sample were placed in a 100-well Zero-Blank autosampler (Costech Analytical Technologies, Valencia, CA 91355, USA). The autosampler was connected to an Elemental Combustion System 4010 (ECS 4010; Costech Analytical Technologies, Valencia, CA 91355, USA), configured for standard dual nitrogen and carbon analysis, under a continuous flow of ultra-high purity (>99.9995%) helium. The ECS combustion reactor was set to 950°C, the reduction reactor was set to 650°C, and the gas chromatography column was set to 30°C. The ECS 4010 was connected to a Delta V Plus Isotope Ratio Mass Spectrometer (Thermo Scientific, Bremen 28199, Germany) via a ConFlo IV (Thermo Scientific, Bremen 28199, Germany) continuous flow interface.
To quantify internal precision, standard on–off (i.e. Zero Enrichment) and linearity tests were performed at the beginning and end of every analytical sequence. External precision and accuracy were quantified through the analysis of certified reference materials, including IAEA-CH-3, NBS-19, NBS-22, USGS-24, IAEA-N-1 and IAEA-N-2, at the beginning and end of each analytical sequence. Internal calibrated reference materials, including acetanilide, urea and collagen, were also analysed at the beginning, middle and end of every analytical sequence for further quality control and checks on potential instrument drift. All isotopic compositions, including sample unknowns, certified reference materials, and calibrated reference materials were reported in standard delta notation. Internal precision was better than 0.1‰ for both δ13C (V-PDB) and δ15N (Air), and external precision and accuracy were both better than 0.2‰ for both δ13C and δ15N. Nitrogen to carbon dioxide fast peak jumps were calibrated prior to each analytical sequence and peak centre scans were performed prior to each individual sample and reference material analysis using Isodat 3.0 (Thermo Scientific, Bremen 28199, Germany).
Isotope ratios are reported parts per thousand (‰) as δX, the ratio of heavy to light isotopes relative to the Vienna Pee Dee Belemnite scale for carbon and Air scale for nitrogen:
where X is either 13C or 15N, and R is either 13C/12C or 15N/14N, respectively.
Statistical analysis
Demographic representativeness and age structure
To assess the demographic representation of kea in our sample, we used a Wilcoxon signed-rank test to compare the proportions of each age group in adjacent and remote areas to those catalogued in the DOC internal database (unpublished data). As all kea catch sites were GPS located, we filtered the DOC database for all kea caught for banding at sites that fell under our definition of adjacent and remote within the same timeframe that latency-to-contact data were collected (2019 to 2021). We then conducted a Chi-square test of independence on our dataset to determine whether there were differences in age structure of kea populations in adjacent and remote areas.
Latency-to-contact experiment
Effect of proximity to humans (adjacent/remote) on the probability of participation and of contacting the novel object was tested using Chi-square tests of independence or two-sided Fisher’s exact tests. Where multiple comparisons were made using the same datasets, Bonferroni adjustments were made.
For the kea that entered the zone (N = 73), we used the binary outcome of the test (contacted/did not contact object) and the time spent in the zone to conduct a Cox proportional hazard regression to determine which variables were associated with increased neophilia. We modelled whether time taken to contact the novel object was related to (a) location (remote n = 54, adjacent n = 19). (b) presence of conspecifics (present n = 53, not present = 20) and (c) age (fledgling n = 12, juvenile/subadult n = 20, adult n = 39 and unknown n = 2), or a combination of these variables. Main effects and two-way interactions were modelled, and the best model was determined as that with the lowest AIC. A separate analysis was conducted using the same methods on the subset of individuals that had been sampled for lead (N = 28) using the same predictor variables as above, with the addition of blood lead concentration as a predictor variable.
Foraging experiment
The trophic levels at which kea forage varies by region (Greer et al. Citation2015a), and to prevent regional variation from obscuring any potential trends, only blood samples from the Fiordland region were used for stable isotope analysis (SIA). Data were analysed using the Bayesian mixing model package MixSIAR (Stock et al. Citation2018) using R software (R Core Team Citation2020). Models were created using informative priors based on feeding observations and faecal analyses in previous studies of kea diet (0.5 animal, 0.5 plant; O'Donnell and Dilks Citation1994; Young et al. Citation2012; Greer et al. Citation2015a). Raw source data were used with no fixed, or nested factors, and a multiplicative residual process error term was applied (Stock and Semmens Citation2016).
Kea-specific diet-feather discrimination factors experimentally determined by Greer and others (Citation2015b) were incorporated into the model. In order to employ this feather discrimination factor in the model, blood isotope ratios were converted to feather ratios according to regression equations from Greer et al. (Citation2015b) that were calculated using simultaneously grown feathers and blood from kea nestlings: δ13Cfeather = 0.973 (δ13Cblood) + 1.122 and δ15Nfeather = 0.920 (δ15Nblood) + 1.041. No data are available on the digestibility of plant and invertebrate food sources in kea, so the uncertainty around the discrimination factors in the mixing models was extended by 0.3‰, according to a recommendation by Phillips et al. (Citation2014): Δ13Cdiet-feather = 4.00‰ ± 0.33; Δ15Ndiet-feather = 3.10‰ ± 0.50. Location (i.e. adjacent/remote) was modelled as a random factor (Semmens et al. Citation2009) to determine whether a difference in diet existed between the two locations. Run length was set to ‘long’ and Gelman-Rubin and Geweke diagnostics were analysed to ensure convergence was reached.
Results
Demographic representativeness and age structure
There was no difference in the relative proportions of each age group in adjacent and remote areas between the DOC dataset and the animals we tested for neophilia (W1 = 9.0, p = 0.438). However, there was a significant difference in age group representation between adjacent and remote areas in our dataset (X23 = 16.56, p < 0.001, N = 83), with a higher proportion of older kea in remote locations and younger kea in adjacent areas. Adults made up the greatest proportion of individuals in remote areas (55.6% compared with 30% in adjacent areas in our dataset), while fledglings comprised 40% of individuals in adjacent areas, and 6.3% in remote areas ().
Table 1. Percent representation of kea age groups in adjacent and remote areas tested in this study and within the DOC database.
Blood lead concentration
Blood lead concentration was tested in 28 individuals, with a range from <3.0 μg/dl (at the lower limit of detectability) to 32.4 μg/dl, with a mean of 9.7 μg/dl. Samples were collected from 9 adults, 13 juvenile/subadults and 6 fledglings of which 13 were sampled in adjacent areas and 15 were remote. Conspecifics were present during latency-to-contact tests for 20 of the 28 individuals that were sampled for lead.
Latency-to-contact experiment
There was an 85–95% participation rate in the latency-to-contact experiment across locations (adjacent and remote). There was no effect of location on the probability of participating in a test (Fisher’s exact test, p = 0.438): of the 63 remote kea in latency-to-contact trials, 14.3% (n = 9) did not participate, and of the 20 adjacent kea, 5.0% (n = 1) did not participate (A). There was, however, a significant association between location and contacting the object (i.e. the highest level of neophilia). Of the kea from adjacent areas to enter the zone (i.e. that participated in the test), 84.2% contacted the object, as opposed to 46.3% of those from remote areas (Fisher’s exact test, p = 0.006; A). Additionally, the median time taken for kea in adjacent areas to contact the object was 3 min (25% percentile 0.5 min; 75% percentile 6.0 min) which was faster than those in remote regions (median 9 min; 25% percentile 4.0 min, 75% percentile 16.25 min).
Figure 3. Percentage of kea in the latency-to-contact experiment that (1) participated and contacted the novel object, (2) participated but did not contact the object, and (3) did not participate in the experiment. A, In adjacent and remote regions. B, By age class. Sample sizes for each category within bars. Total N for each bar above bar. Note, N is lower in panel B because the age of some kea could not be reliably determined from a distance.
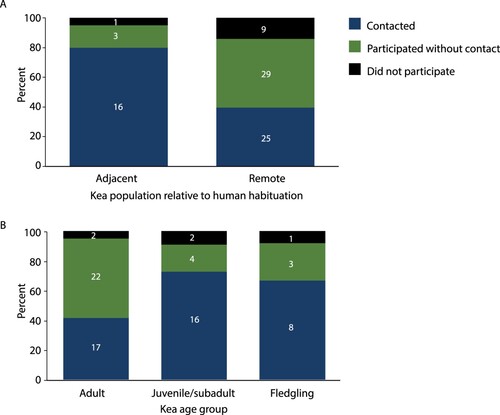
There was no effect of age (adult, juvenile/subadult, fledgling) on test participation (X22 = 0.472, p < 0.79, N = 75; B), but there was a significant effect of age on whether the participants contacted the object (X22 = 8.301, p < 0.016, N = 70; B). Of the kea that entered the zone, 43.6% of adults, 80.0% of juvenile/subadults and 72.7% of fledglings contacted the object. Adults were less likely to contact the object than a kea of a younger age group. This was a marginal trend for adult vs. fledglings (Fisher’s exact test p = 0.17, N = 50) and was significant for adult vs. juvenile/subadults (Bonferroni-corrected Fisher’s exact test p = 0.024, N = 59). There was no difference in the proportion of the juvenile/subadult group and fledglings to contact the object (Fisher’s exact test p = 0.676, N = 31).
Cox regression analysis showed the best model to be main effects (AIC = 209.3), rather than two-way interactions (AIC = 214.0) relative to an empty model with no covariates (AIC = 224.4). Age was the greatest predictor of a kea contacting the object, with juveniles and fledglings at least three times more likely to contact the object at any given time than adults (hazard ratio (HR) juvenile = 3.8, 95% CI = 1.7–8.5; HR fledgling = 3.2, 95% CI = 1.2–8.1; ). Kea in adjacent areas were also around three times more likely to contact the object than those in remote areas (HR adjacent = 3.1, 95% CI = 1.5-6.4; ). The presence of conspecifics did not have a significant effect (HR = 1.6, 95% CI = 0.73–3.6).
Figure 4. Cumulative counts of minutes taken to contact the novel objects by kea that participated in the latency-to-contact experiment. A, In regions adjacent to human settlements or roads (N = 19) and those remote (>40 km) from human settlements (N = 54). B, By adult (N = 39), juvenile/subadult (N = 20) and fledgling (N = 11) kea. C, By kea with conspecifics present (N = 62) and not present (N = 21).
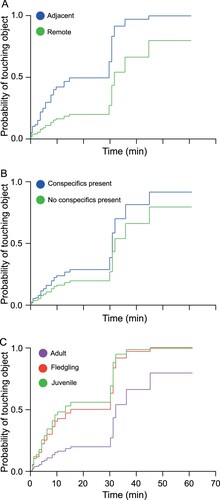
In the reduced model that included only individuals that had been sampled for lead, for every unit increase in BLC (μg/dl), a kea’s HR was increased by 6.5% (HR = 1.065, 95% CI = 1.0–1.1).
Foraging experiment
Nitrogen isotope ratios in the blood of kea in remote areas ranged from – 1.32 to 5.96 ‰, with a mean of 0.82 ‰ and those of adjacent kea ranged from – 3.55 to 6.75 ‰, mean 0.26 ‰. The ratio of carbon isotopes in remote kea blood ranged from – 28.14 to – 22.73‰, mean – 25.08 ‰ and adjacent kea from – 26.96 to – 23.81 ‰, mean – 25.28 ‰. Stable isotope mixing models estimated the diet of kea in adjacent areas to be 90.2% plants (95 C.I. = 75.3–100%) which was a slightly lower trophic level than kea in remote populations (73.3% plants, 95% C.I. = 62.8–84.6%; ).
Discussion
We found that kea exploratory and foraging behaviour differed relative to their proximity to humans: adjacent populations were more explorative and/or less cautious, foraged at a lower trophic level, and exhibited a younger age structure than populations remote from human influence. Neophilic behaviour was also related to age and blood lead levels. Younger kea and kea with higher blood lead levels took less time to contact novel objects than adults, but we found no effect of conspecific presence on the time taken to approach a novel object.
Many of the kea that approached a novel object exhibited a high degree of neophilia, regardless of location. Following an initial approach, however, a greater proportion of kea adjacent to human settlements contacted the object and were faster to do so than individuals from remote areas, suggesting that kea from adjacent areas adopted a less cautious approach. Our findings are consistent with several avian studies indicating a reduction in neophobia associated with urban environments (e.g. Sol et al. Citation2011; Tryjanowski et al. Citation2016; Jarjour et al. Citation2020). That the opposite trend is also observed (e.g. Echeverría and Vassallo Citation2008; Miranda et al. Citation2013) reinforces the idea that a species’ ecology can determine the direction of a behavioural change (Mettke-Hofmann et al. Citation2002). Human-influenced environments enable greater exposure to novelty, which may cause behaviourally plastic species to alter to suit their environment and experience (Greenberg and Mettke-Hofmann Citation2001). Exploratory tendencies in birds may also be inherited (Miranda Citation2017); however, given the kea’s ability for wide dispersal it is less plausible that the observed difference is caused by micro-evolutionary change than by behavioural plasticity.
In many animals, including birds, younger individuals exhibit enhanced neophilia relative to older conspecifics because the risk of exploration is surpassed by the enhanced learning opportunity for young individuals (Vince Citation1960; O’Hara et al. Citation2017). This was evident in our study, with juveniles/subadults being the most likely to contact the object. Kea in adjacent areas were significantly younger than those in remote areas, both in our study and in the wider DOC database, which may partially explain the elevated neophilia in adjacent populations, however our models did not find the combination of age and location to be significant. It is unclear why younger kea appear to be attracted to human settlements, but their gregariousness, and the high incidence of novelty, as well as a steady supply of anthropogenic food, may attract young kea that lack the experience to forage efficiently for themselves.
Kea with higher BLC approached and contacted the object faster than those with lower BLC, which is consistent with an unpublished study showing that kea with more explorative personalities had a higher BLC (Reid Citation2008). Attributing cause is problematic, because having an explorative personality may predispose a kea to lead exposure, while lead exposure may generate increased exploration due to its neurobehavioural effects. However, studies on lab-reared and wild animals that have looked at the effect of lead on exploratory behaviour have typically either found no lasting effect (e.g. Ruuskanen et al. Citation2015), or reduced exploration with increasing BLC (e.g. Moreira et al. Citation2001; Grunst et al. Citation2018). Our finding that neophilia and BLC appear to be linked in kea suggests that the response to lead exposure may depend on the ecology of the species. There is evidence that neophobic behaviour is unrelated to toxic metal exposure in great tits, Parus major (Grunst et al. Citation2019), which may indicate that more explorative kea may be predisposed to lead poisoning, rather than the lead causing the behavioural change, although this should be viewed with caution given the low sample size of individuals with very elevated blood lead levels in this study. If kea with explorative personalities are predisposed to ingest lead, these individuals may suffer reduced reproductive success due to the toxin’s influence on their behaviour and reproductive physiology, and selection for less explorative individuals may result. Kea in both adjacent and remote areas displayed elevated BLC and further investigation of spatial distribution of lead poisoning is warranted. The high incidence of elevated BLC in our samples calls for an urgent need to remove lead sources from the kea’s environment.
Stable isotope analyses of carbon and nitrogen in kea blood indicated that adjacent kea foraged at a lower trophic level than remote populations. This observation may be partly explained by the younger demographic of kea in near proximity to humans. Young kea naturally forage at a lower trophic level than adults (McLean et al. CitationIn Press), possibly due to a lack of foraging experience or differing metabolic needs to adults. The prevalence of lead poisoning in kea populations near human settlements (Reid et al. Citation2012) may also explain the observed trophic level difference. BLC is negatively correlated with trophic level of kea foraging (LM, unpublished data) and the neurobehavioural effects of lead may impair kea performing the cognitively demanding and learnt behaviours (e.g. excavating, searching for hidden foods) needed to forage for high trophic level foods. When exposed to lead, herring gulls (Larus argentatus), a species with a similar niche and behavioural pattern to kea, perform poorly in learned tasks (Burger and Gochfeld Citation2005). Alternatively, the difference may simply be caused by food availability, given that adjacent and remote areas were separated by at least 40 km and regional variation in kea diet (Greer et al. Citation2015a) is reflective of food availability in different areas, but this seems somewhat unlikely as all kea were sampled within the same ecological region (Fiordland). Finally, the difference may reflect a dietary difference that is caused by anthropogenic foods. Many of the adjacent kea in this study were sampled for stable isotope analysis near Homer Tunnel, a known scrounge site with a consistent source of low trophic level foods, such as bread (LM pers. obs.). Whether this food supply contributes a significant portion of foods consumed by local kea or is consumed over and above naturally sourced foods is unknown. If anthropogenic foods make up a high proportion of assimilated nutrients, we expect to see a correspondingly low trophic level in these populations (as observed here). However, detailed stable isotope analyses that incorporate human food source data are needed to make the distinction between anthropogenic foods and the possible drivers of the observed low trophic level in the adjacent populations tested.
Increased neophilia or willingness to explore novelty among adjacent kea supports our finding indicating that kea in areas adjacent to humans may be at higher risk of ingesting poison baits than remote kea (Kemp et al. Citation2019). Despite the observed behavioural differences, kea are highly mobile (Jackson Citation1960), and individuals may move between these areas, or occupy habitat between these extremes. With detailed movement data unavailable, any delineation between adjacent and remote populations in conservation policy requires further research to understand dispersal dynamics. In our analysis, the presence of conspecifics did not affect neophilia in kea, which was unexpected given that kea often forage in demographically mixed groups (Diamond and Bond Citation1991) which may influence consumption of novel food (see Greggor et al. Citation2015 for a discussion).
We urge care in extrapolating our results into a conservation management context due to several caveats. First, contacting a plastic block does not necessarily engender ingestion of poison bait. While this is a plausible outcome given the kea’s inquisitive nature and opportunistic generalist foraging behaviour, the conditions of this test and a 1080 operation differ, as our latency-to-contact test was designed to draw attention to the novel object by having a human place it in the presence of the test subject, rather come across it as a chance encounter. Although it is not known what effect the human observer has on encounter rate, our results do indicate relative behavioural differences between adjacent and remote populations. In a practical sense, while the time taken for a kea to approach a novel object is irrelevant if the eventual outcome is the same (i.e. ingestion of poison bait), our results show that remote populations are also less likely to contact the object, which implies a higher rate of avoidance of novelty in these populations. Reassuringly, we verified that adult kea, and those in remote locations, are the least neophilic, suggesting that they may elude future encounters with poison baits. As kea are fast learners and can be conditioned to avoid baits for long periods of time (Nichols et al. Citation2020; McLean et al. Citation2022), strategies in which conditioned aversion training is targeted at populations near human settlements, and at times of the year in which fledglings have left the nest, would likely be effective methods to trial before future bait drops.
Acknowledgements
The authors are grateful to the KCT and DOC fieldworkers who assisted with data collection and provided feedback on the methods, and to reviewers for significantly improving this manuscript. This work was carried out under a Wildlife Act Authority granted to Kea Conservation Trust (53716-FAU) and was approved by the University of Canterbury Animal Ethics Committee (2018/19R).
Data availability statement
Latency to contact data are available in Dryad doi: 10.5061/dryad.4tmpg4fft
.Disclosure statement
No potential conflict of interest was reported by the author(s).
Additional information
Funding
References
- Berlyne DE. 1950. Novelty and curiosity as determinants of exploratory behaviour. Br J Psychol. 41:68–80.
- Brown GE, Ferrari MC, Elvidge CK, Ramnarine I, Chivers DP. 2013. Phenotypically plastic neophobia: a response to variable predation risk. Proc Roy Soc, B. 280:20122712. doi:10.1098/rspb.2012.2712
- Burger J, Gochfeld M. 2005. Effects of lead on learning in herring gulls: an avian wildlife model for neurobehavioral deficits. Neurotoxicology. 26:615–624. doi:10.1016/j.neuro.2005.01.005
- Cowan P, Crowell M. 2017. Visual and taste cues for minimising native bird interactions with toxic 1080 baits – a review of current practices. N Z J Ecol. 41:178–185.
- Dalerum F, Angerbjorn A. 2005. Resolving temporal variation in vertebrate diets using naturally occurring stable isotopes. Oecologia. 144:647–658. doi:10.1007/s00442-005-0118-0
- Diamond J, Bond AB. 1991. Social behavior and the ontogeny of foraging in the kea (Nestor notabilis). Ethology. 88:128–144. doi:10.1111/j.1439-0310.1991.tb00268.x
- Echeverría AI, Vassallo AI. 2008. Novelty responses in a bird assemblage inhabiting an urban area. Ethology. 114:616–624. doi:10.1111/j.1439-0310.2008.01512.x
- Elliott G, Kemp J. 2016. Large-scale pest control in New Zealand beech forests. Ecol Manag Restor. 17:200–209. doi:10.1111/emr.12227
- Gajdon GK, Fijn N, Huber L. 2004. Testing social learning in a wild mountain parrot, the kea (Nestor notabilis). Learn Behav. 32:62–71. doi:10.3758/BF03196007
- Gajdon GK, Fijn N, Huber L. 2006. Limited spread of innovation in a wild parrot, the kea (Nestor notabilis). Anim Cogn. 9:173–181. doi:10.1007/s10071-006-0018-7
- Greenberg R. 2003. The role of neophobia and neophilia in the development of innovative behaviour of birds. In: Reader SM, Laland KN, editors. Animal innovation. Oxford University Press; p. 175–196.
- Greenberg R, Mettke-Hofmann C. 2001. Ecological aspects of neophobia and neophilia in birds. Curr Ornithol. 16:119–178.
- Greer AL, Gajdon GK, Nelson XJ. 2015a. Intraspecific variation in the foraging ecology of kea, the world’s only mountain-and rainforest-dwelling parrot. N Z J Ecol. 39:254–261.
- Greer AL, Horton TW, Nelson XJ. 2015b. Simple ways to calculate stable isotope discrimination factors and convert between tissue types. Methods Ecol Evol. 6:1341–1348. doi:10.1111/2041-210X.12421
- Greggor AL, Thornton A, Clayton NS. 2015. Neophobia is not only avoidance: improving neophobia tests by combining cognition and ecology. Curr Opin Behav Sci. 6:82–89. doi:10.1016/j.cobeha.2015.10.007
- Griffin AS, Netto K, Peneaux C. 2017. Neophilia, innovation and learning in an urbanized world: a critical evaluation of mixed findings. Curr Opin Behav Sci. 16:15–22. doi:10.1016/j.cobeha.2017.01.004
- Grunst AS, Grunst ML, Pinxten R, Eens M. 2019. Personality and plasticity in neophobia levels vary with anthropogenic disturbance but not toxic metal exposure in urban great tits: urban disturbance, metal pollution and neophobia. Sci Total Environ. 656:997–1009. doi:10.1016/j.scitotenv.2018.11.383
- Grunst AS, Grunst ML, Thys B, Raap T, Daem N, Pinxten R, Eens M. 2018. Variation in personality traits across a metal pollution gradient in a free-living songbird. Sci Total Environ. 630:668–678. doi:10.1016/j.scitotenv.2018.02.191
- Hobson KA, Gibbs HL, Gloutney ML. 1997. Preservation of blood and tissue samples for stable-carbon and stable-nitrogen isotope analysis. Can J Zool. 75:1720–1723. doi:10.1139/z97-799
- Jackson JR. 1960. Keas at Arthurs Pass. Notornis. 9:39–58.
- Jarjour C, Evans JC, Routh M, Morand-Ferron J. 2020. Does city life reduce neophobia? A study on wild black-capped chickadees. Behav Ecol. 31:123–131.
- Kavčič I, Adamič M, Kaczensky P, Krofel M, Kobal M, Jerina K. 2015. Fast food bears: brown bear diet in a human-dominated landscape with intensive supplemental feeding. Wildlife Biol. 21:1–8. doi:10.2981/wlb.00013
- Kemp J, Mosen CC, Elliot G, Hunter CM. 2018. Effects of the aerial application of 1080 to control pest mammals on kea reproductive success. N Z J Ecol. 42:1–11.
- Kemp J, Mosen CC, Elliott G, Hunter C, van Klink P. 2019. Kea survival during aerial poisoning for rat and possum control. N Z J Ecol. 43:3351.
- Kennedy EM, Kemp JR, Mosen CC, Perry GL, Dennis TE. 2015. GPS telemetry for parrots: a case study with the Kea (Nestor notabilis). The Auk: Ornithological Advances. 132:389–396.
- Martini D, Dussex N, Robertson BC, Gemmell NJ, Knapp M. 2021. Evolution of the ‘world’s only alpine parrot’: genomic adaptation or phenotypic plasticity, behaviour and ecology? Mol Ecol. 30:6370–6386. doi:10.1111/mec.15978
- McLean LRW, Horton TW, Robertson BC, Robertson F, Nelson XJ. In Press. Reproductive roles as likely drivers of sexual dimorphism in New Zealand’s endangered alpine parrot, the kea. Ornithology. forthcoming.
- McLean LRW, Nichols M, Taylor AH, Nelson XJ. 2022. Memory retention of a conditioned aversion in New Zealand’s alpine parrot, the kea (Nestor notabilis). J Wildl Manag. 86:22221. doi:10.1002/jwmg.22221
- Mettke-Hofmann C, Winkler H, Leisler B. 2002. The significance of ecological factors for exploration and neophobia in parrots. Ethology. 108:249–272. doi:10.1046/j.1439-0310.2002.00773.x
- Miranda AC. 2017. Mechanisms of behavioural change in urban animals: the role of microevolution and phenotypic plasticity. In: Murgui E, Hedblom M, editors. Ecology and conservation of birds in urban environments. Cham: Springer; p. 113–132.
- Miranda AC, Schielzeth H, Sonntag T, Partecke J. 2013. Urbanization and its effects on personality traits: a result of microevolution or phenotypic plasticity? Glob Chang Biol. 19:2634–2644. doi:10.1111/gcb.12258
- Montgomery K. 1955. The relation between fear induced by novel stimulation and exploratory drive. J Comp Physiol Psychol. 48:254. doi:10.1037/h0043788
- Moreira EG, Vassilieff I, VSl V. 2001. Developmental lead exposure: behavioral alterations in the short and long term. Neurotoxicol Teratol. 23:489–495. doi:10.1016/S0892-0362(01)00159-3
- Nichols M, Bell P, Mulgan N, Taylor A. 2020. Conditioned aversion in kea to cereal bait: a captive study using anthraquinone. Appl Anim Behav Sci. 230:105077. doi:10.1016/j.applanim.2020.105077
- O'Donnell CF, Dilks PJ. 1994. Foods and foraging of forest birds in temperate rainforest, South Westland, New Zealand. N Z J Ecol. 18:87–107.
- O’Hara M, Mioduszewska B, von Bayern A, Auersperg A, Bugnyar T, Wilkinson A, Huber L, Gajdon GK. 2017. The temporal dependence of exploration on neotic style in birds. Sci Rep. 7:4742. doi:10.1038/s41598-017-04751-0
- Orr-Walker T, Adams NJ, Roberts LG, Kemp JR, Spurr EB. 2012. Effectiveness of the bird repellents anthraquinone and d-pulegone on an endemic New Zealand parrot, the kea (Nestor notabilis). Appl Anim Behav Sci. 137:80–85. doi:10.1016/j.applanim.2012.01.002
- Pearce G, Russell EM. 1971. Exploration of novel objects by marsupials. Behaviour. 40:312–322. doi:10.1163/156853971X00456
- Phillips DL, Inger R, Bearhop S, Jackson AL, Moore JW, Parnell AC, Semmens BX, Ward EJ. 2014. Best practices for use of stable isotope mixing models in food-web studies. Can J Zool. 92:823–835. doi:10.1139/cjz-2014-0127
- Powell SB, Paulus MP, Hartman DS, Godel T, Geyer MA. 2003. Ro-10-5824 is a selective dopamine d4 receptor agonist that increases novel object exploration in c57 mice. Neuropharmacology. 44:473–481. doi:10.1016/S0028-3908(02)00412-4
- R Core Team. 2020. R: A Language and Environment for Statistical Computing. R Foundation for Statistical Computing, Vienna, Austria: https://www.R-project.org/.
- Reid C. 2008. Exploration – avoidance and an anthropogenic toxin (lead Pb) in a wild parrot (kea: Nestor notabilis). (Master of Science), Victoria University of Wellington.
- Reid C, McInnes K, McLelland JM, Gartrell BD. 2012. Anthropogenic lead (Pb) exposure in populations of a wild parrot (kea Nestor notabilis). N Z J Ecol. 36:56–63.
- Ruuskanen S, Eeva T, Kotitalo P, Stauffer J, Rainio M. 2015. No delayed behavioral and phenotypic responses to experimental early-life lead exposure in great tits (Parus major). Environ Sci Pollut Res. 22:2610–2621. doi:10.1007/s11356-014-3498-z
- Schwing R. 2010. Scavenging behaviour of kea (Nestor notabilis). Notornis. 57:98–99.
- Semmens BX, Ward EJ, Moore JW, Darimont CT. 2009. Quantifying inter-and intra-population niche variability using hierarchical Bayesian stable isotope mixing models. PLoS ONE 4:e6187. doi:10.1371/journal.pone.0006187
- Sol D, Griffin AS, Bartomeus I, Boyce H. 2011. Exploring or avoiding novel food resources? The novelty conflict in an invasive bird. PLoS ONE. 6:e19535. doi:10.1371/journal.pone.0019535
- Stock BC, Jackson AL, Ward EJ, Parnell AC, Phillips DL, Semmens BX. 2018. Analyzing mixing systems using a new generation of Bayesian tracer mixing models. PeerJ. 6:e5096. doi:10.7717/peerj.5096
- Stock BC, Semmens BX. 2016. Unifying error structures in commonly used biotracer mixing models. Ecology. 97:2562–2569. doi:10.1002/ecy.1517
- Tebbich S, Taborsky M, Winkler H. 1996. Social manipulation causes cooperation in keas. Anim Behav. 52:1–10. doi:10.1006/anbe.1996.0147
- Tryjanowski P, Møller AP, Morelli F, Biaduń W, Brauze T, Ciach M, Czechowski P, Czyż P, Dulisz B, Goławski A, Hetmański T. 2016. Urbanization affects neophilia and risk-taking at bird-feeders. Sci Rep. 6:1–7. doi:10.1038/srep28575
- Vince M. 1960. Developmental changes in responsiveness in the great tit (Parus major). Behaviour. 15:219–242. doi:10.1163/156853960X00241
- Weisstaub NV, Zhou M, Lira A, Lambe E, González-Maeso J, Hornung J-P, Sibille E, Underwood M, Itohara S, Dauer WT, Ansorge MS. 2006. Cortical 5-ht2a receptor signaling modulates anxiety-like behaviors in mice. Science. 313:536–540. doi:10.1126/science.1123432
- Weser C, Ross JG. 2013. The effect of colour on bait consumption of kea (Nestor notabilis): implications for deterring birds from toxic baits. N Z J Zool. 40:137–144. doi:10.1080/03014223.2012.710639
- Winkler H, Leisler B. 1999. Exploration and curiosity in birds: functions and mechanisms. Proceedings of the 22nd International Ornithological Congress, Durban.
- Young LM, Kelly D, Nelson XJ. 2012. Alpine flora may depend on declining frugivorous parrot for seed dispersal. Biol Cons. 147:133–142. doi:10.1016/j.biocon.2011.12.023