Abstract
High prevalence of Plasmodium infection in some non-native bird species in New Zealand, coupled with distributional expansion of the introduced mosquito vector Culex quinquefasciatus, has raised concerns that immunologically naïve native species might be increasingly at risk from malarial parasites. We combined polymerase chain reaction (PCR) screening of archived blood samples with long-term field data of red-billed gulls (Larus scopulinus) at Kaikoura Peninsula to estimate the prevalence of malarial infection and its effect upon host condition and breeding success. Plasmodium prevalence assessed with PCR was 7.8±3.4%, 10-fold greater than estimates from microscopic analysis of blood smears (c. 0.7%, Tompkins et al. 2010). Phylogenetic analysis of parasite cytochrome b sequences isolated from red-billed gulls indicates that lineage RBG1 falls within a clade containing P. cathemerium morphospecies, whereas RBG2, isolated from a single individual, clusters with P. elongatum. We show that red-billed gulls infected with Plasmodium have bred at Kaikoura dating back to at least 1992, that chronic infections of lineage RBG1 are maintained over several years in some individuals, and that infection is associated with decreased body condition in breeding individuals of both sexes. There is currently no evidence that Plasmodium infection is associated with reduced breeding performance. However, further study involving the ecological context and temporal dynamics of infection is needed to assess cumulative effects on lifetime reproductive success.
Introduction
The emergence and spread of vector-borne diseases is a research priority for risk management in biodiversity protection and wildlife conservation (Daszak et al. Citation2000). Perhaps best studied amongst avian parasites are intracellular haemosporidians of the genera Haemoproteus, Plasmodium, and Leucocytozoon, with species of Plasmodium as the causative agent of avian malarial infections (Valkiūnas Citation2005). Vectored by blood-sucking mosquitoes (Diptera: Culicidae), Plasmodium infections progress from a prepatent phase where parasites multiply in host tissues to an acute phase with peak parasitaemia in circulating blood cells. Individuals surviving the acute phase of infection may harbour chronic infections characterized by low levels of circulating parasites, or latent infections where parasites are sequestered in internal organs (Atkinson & van Riper Citation1991; Valkiunas Citation2005). Such individuals may therefore persist as asymptomatic carriers and act as reservoirs of infection that facilitate the spread of disease throughout local avian communities (Atkinson &van Riper Citation1991; Valkiūnas Citation2005; Tompkins & Gleeson Citation2006).
The health consequences associated with primary Plasmodium infection can vary from no detectable effect to severe illness and ultimately death (Bennett et al. Citation1993; Valkiunas Citation2005). Hemolysis and phagocytosis of parasitized erythrocytes can lead to severe anaemia. Decreased plasma pH and increased blood protein concentrations can further exacerbate the effect of lowered red blood cell counts by decreasing the oxygen-binding capacity of haemoglobin molecules and impairing capillary circulation during the peak of infection (van Riper et al. Citation1994; Valkiūnas Citation2005; Atkinson Citation2008). Enlargement of the spleen and liver due to hyperplasia (unusual cell proliferation) or elevated phagocytic activity of lymphoid-macrophage cells is also characteristic of haemosporidian infections (van Riper et al. Citation1994; Valkiūnas Citation2005; Atkinson Citation2008). Additionally, inflammation or damage to endothelial cells lining capillary walls or capillary occlusion by agglomerations of parasitized erythrocytes can limit blood flow to internal organs, resulting in anoxia, necrosis, or cerebral stroke (van Riper et al. Citation1994; Atkinson Citation2008).
Several factors probably interact to determine the severity and pathogenicity of Plasmodium infections, including parasite species and lineage, co-infection with multiple malarial parasites or concomitant infection with other pathogens, and host species, sex, or age (Weatherhead & Bennett Citation1992; Valkiūnas Citation2005; Marzal et al. Citation2008; Palinauskas et al. Citation2008; Alley et al. Citation2010). The ecological and life history context of infection may also contribute to both the severity of primary infection and recrudescence of chronic infections by influencing host condition and stress-mediated responses during periods of poor food availability or unfavourable environmental conditions, or as the result of trade-offs between resources allocated to immune system function and energetically costly behaviours such as breeding and migration (Applegate Citation1970; Sheldon & Verhulst Citation1996; Møller Citation1997; Nordling et al. Citation1998; Merino et al. Citation2000; Garvin et al. Citation2006; French et al. Citation2009; Knowles et al. Citation2010). Reports of mortality in captive individuals, where greatest susceptibility is often found in immunologically naïve individuals originating from regions free of Plasmodium spp., indicate that host immunogenetics and the evolutionary history of host-parasite interactions are also potentially important determinants of disease outcome (Bennett et al. Citation1993; Valkiūnas Citation2005). Less is known about the pathogenicity of avian malaria in the wild, as affected individuals are unlikely to be sampled during the peak of infection (Bennett et al. Citation1993). However, dramatic losses of endemic bird species following the introduction of both Plasmodium relictum and the exotic vector Culex quinquefasciatus to the Hawaiian Islands is a classic example of the potential impact of avian malaria on native species that have evolved in its absence (van Riper et al. Citation1986; Atkinson et al. Citation1995).
The same combination of introduced malarial parasites, exotic mosquito vectors, and an immunologically naïve avifauna that is associated with such severe consequences for Hawaiian species is also of concern in New Zealand. Plasmodium spp., believed to be historically absent from New Zealand, have now been reported in a number of native bird species, and deaths of captive keas (Nestor notabilis), yellowheads (Mohoua ochrocephala), and New Zealand dotterel (Charadrius obscurus) following exposure to malarial parasites underscore the potential vulnerability of wild populations to Plasmodium infection (Bennett et al. Citation1993; Holder et al. Citation1999; Tompkins & Gleeson Citation2006; Derraik et al. Citation2008; Tompkins et al. Citation2008). Recent surveys have reported a high prevalence of malarial infection in some non-native bird species, and the presence of such reservoir hosts, combined with the southward spread of C. quinquefasciatus from its original sites of introduction in Auckland and Northland, raises concerns that native species may be increasingly at risk from exposure to Plasmodium parasites (Holder et al. Citation1999; Tompkins & Gleeson Citation2006).
Although the risk of avian malaria is well recognized, there is still little information concerning its distribution, prevalence, and impact on native species throughout New Zealand. Recent efforts to address this issue have included large-scale sampling of arthropod vectors and disease screening of native and introduced bird species (Cane & Slaney Citation2008, Citation2009; Tompkins et al. Citation2010). Included in surveyed locations is the Kaikoura Peninsula (42°26′S 173°42′E), where the largest breeding colony of red-billed gulls (Larus scopulinus, also L. novaehollandiae scopulinus) on the South Island is situated (Gurr & Kinsky Citation1965). Low haemosporidian prevalence in peripheral blood smears and low abundance of mosquito vectors at Kaikoura suggest that transmission of malarial infection at this site is limited by the availability of suitable vector habitat (Cane & Slaney Citation2008, Citation2009; Tompkins et al. Citation2010). However, while microscopic examination of blood films is a valuable tool for linking sequences to parasite species and identifying co-infection by multiple malarial parasites (Valkiūnas et al. Citation2006,, Citation2008a), polymerase chain reaction (PCR) diagnostics provide greater sensitivity to detect low-intensity infections (Jarvi et al. Citation2002; Fallon & Ricklefs Citation2008). Low-level chronic infection could be common if individuals are exposed to malarial parasites primarily during the non-breeding season and have already progressed through the acute stage of infection prior to their return to breed at Kaikoura. Identification of these infections, and the sequencing of parasite genetic lineages, is essential to better understand the extent of exposure and transmission dynamics of malarial infection in native species, and to assess the possible health impacts of acute versus chronic infections.
The Kaikoura colony of red-billed gulls is the subject of extensive research, with annual monitoring of breeding biology since 1964, and the collection of blood samples for genetic analyses beginning in 1992. This population has declined substantially in recent years, from a relatively stable adult population of 16,000–19,000 individuals from 1983–1993 to an estimated 9000 individuals by 2003 (Mills et al. Citation2008). Although red-billed gulls remain a common species nationally (Wilson Citation2007), recent declines at Kaikoura, as well as at the two largest North Island breeding colonies (Three Kings Islands and Mokohinau Islands), have led to their classification as a nationally vulnerable threatened species according to the New Zealand Threat Classification System (Miskelly et al. Citation2008). While the factors contributing to these declines are not fully understood, the suggested involvement of changes to ocean productivity, possibly linked to global climate change (Mills et al. Citation2008; Miskelly et al. Citation2008), means that the impact of avian malaria could be compounded by stresses associated with food limitation or fluctuating environmental conditions during the breeding period.
High philopatry with respect to natal colony (Mills Citation1973) and long-term study involving ringing of nestlings and individual colour-banding of adults has resulted in pedigree, survivorship, and breeding success data for a large subset of the Kaikoura population (Mills et al. Citation2008). Knowledge of baseline ecological and life history parameters for this population, combined with the ability to monitor the fate of infected individuals, provides valuable information that is often not available when studying parasitism in wild populations (Wobeser Citation2008). In this study, we used PCR techniques to retroactively screen for malarial infection in blood samples collected from adult red-billed gulls at Kaikoura during the 1992–2004 breeding seasons. Used in conjunction with the available field data, our aim was to assess not only the prevalence of Plasmodium infection, but also the potential consequences of infection upon survivorship, breeding condition, and reproductive performance within this population.
Methods
Field methods and sample collection
Red-billed gulls begin arriving at Kaikoura in mid-July, with egg laying typically starting in the first week of October (Mills Citation1973). Although red-billed gulls are single brooded, re-laying frequently occurs following loss of the first clutch or brood, and extends the egg laying period through early January (Mills et al. Citation1996). During the non-breeding period, most adults disperse from Kaikoura and have been sighted as far away as Auckland and Invercargill, but most individuals are found within a distance of 380 km of the colony (J. Mills, unpubl. data).
During each breeding season, nests of colour-marked adults were numbered and checked at least every 2–3 days. Adults were caught using automatic drop traps during incubation, or by pole netting if caught later in the season. Weight (measured to the nearest gram), and morphological measurements (including unflattened wing chord to the nearest cm, and total culmen length and bill depth through angle, to the nearest mm) were recorded, and blood samples were collected by brachial venipuncture and flash-frozen in liquid nitrogen or stored in 70% ethanol. Individuals were sexed by a combination of bill length and depth at gonys (Mills Citation1971), and sexes were confirmed by DNA-based typing using primers 2550F/2718R and methods as described in Fridolfsson and Ellegren (Citation1999). Fieldwork was performed in accordance with permits issued by the New Zealand Department of Conservation.
Screening for avian malarial infection
A total of 271 blood samples collected from 243 adult individuals were screened for malarial infection. Individuals were sampled only once within a breeding season. However, blood samples from multiple seasons were available for 27 of the 243 individuals, with 26 individuals sampled in two different years, and the remaining individual sampled in three years. DNA was isolated from whole blood using the DNeasy Blood & Tissue Kit (Qiagen). Screening for malarial infection used established PCR diagnostic techniques to amplify a portion of the mitochondrial cytochrome b gene from Haemoproteus and Plasmodium parasites with primer sets HAEMF/HAEMR2 (Bensch et al. Citation2000; Waldenström et al. Citation2004), and L15183/H15725 (Szymanksi & Lovette Citation2005). As only Plasmodium infections were detected during initial screening, and because nested PCR approaches have been shown to result in a higher detection rate of Plasmodium infections, which often occur at low intensity (Waldenström et al. Citation2004), we also attempted to screen samples using an established nested PCR approach with primers HAEMNF/HAEMNR2 followed by HAEMF/HAEMR2 as described by Waldenström et al. (Citation2004). However, our use of the published protocol produced a nonspecific PCR band of similar size to the target product (sequencing of this product yielded messy sequence traces matching a region of the chicken Z chromosome with a BLAST evalue of 2e−19). We were unable to optimize PCR conditions to eliminate this additional product without also losing detection of some confirmed positive samples. We therefore used FastPCR v. 6.0 software (Kalendar et al. Citation2009) to design a new set of nested PCR primers from an alignment of the Plasmodium lineage (RBG1) isolated from all individuals testing positive using the single PCR screening methodologies and sequences of described Plasmodium morphospecies ().
Figure 1 Alignment of Plasmodium cytochrome b nucleotide sequences used for nested Avmal primer design. Species names are followed by GenBank accession numbers. Dots indicate nucleotide identity with red-billed gull isolate RBG1, and primer-binding sites are shaded in grey.
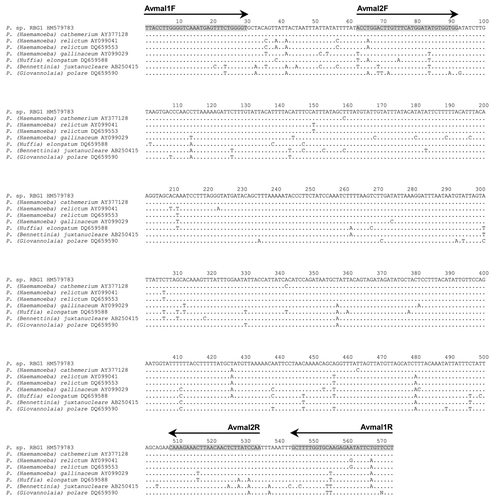
A 573 bp product (including primers) was amplified with primers Avmal1F (5′-TTACCTTGGGGTCAAATGAGTTTCTGGGGT-3′) and Avmal1R (5′-AGGAACAGAATATTCTCTTGCACCAAAAGC-3′). Each 25 µL reaction contained final concentrations of: 1X Erika Hagelberg (EH) buffer (10 mM Tris-HCl pH 8.3, 50 mM KCl, 2.5 mM MgCl2, 0.01% gelatin), 0.4 mM of each dNTP, 0.6 µM of each primer, 0.5 U Taq DNA polymerase (Invitrogen), and 50–100 ng of template DNA. PCR cycling conditions were: initial denaturation at 94 °C for 5 min, followed by five cycles with denaturation at 94 °C for 45 s, touchdown annealing of 60 °C → 55 °C (−1 °C/cycle) for 45 s, and elongation at 72 °C for 45 s, an additional 20 cycles with constant annealing at 55 °C, and a final elongation step at 72 °C for 5 min. Subsequently, 2 µL of the first amplification product was used in a nested PCR with primers Avmal2F (5′-ACCTGGACTTGTTTCATGGATATGTGGTGG-3′) and Avmal2R (5′-TTGGATAAGAGTTGTTAAGTTTCTTTG-3′) to produce a 472 bp final product, including priming sites. Reaction conditions were as described for the initial PCR, with the exception of a touchdown annealing profile of 58 °C → 52 °C (−1 °C/cycle), followed by 30 cycles with constant annealing at 52 °C.
Due to our use of archival blood samples collected for other research purposes, blood smears were unavailable to validate the diagnostic efficacy (sensitivity and specificity) of the newly developed nested PCR primers. We stress that our purpose was to design a nested protocol to identify additional infections corresponding to the lineage (RBG1) isolated during preliminary screening with the two established single PCR protocols, and we do not advocate the use of this new methodology as a general diagnostic tool. As a conservative approach, we screened all samples with each of the three PCR methodologies, and sequenced both strands of all PCR products. Negative and positive controls were included in each PCR run, and the quality of template DNA was evaluated by UV spectrophotometry and by amplification of a panel of 14 red-billed gull nuclear loci to ensure that negative results were not caused by amplification failure due to poor quality DNA. PCR products were visualized on 1.2% agarose gels, and positive samples recovered by band excision and filtered pipette tip centrifugation (Dean & Greenwald Citation1995). Standard sequencing reactions used 4 µL of recovered DNA with Big Dye Terminator v. 3.1 chemistry (Applied Biosystems), and fragments were resolved by automated capillary electrophoresis on an ABI3100.
Phylogenetic analysis
ChromasPro v. 1.5 (Technelysium Pty Ltd.) was used for sequence editing and contig assembly. BLAST (Altschul et al. Citation1997) similarity searches were used to compare Plasmodium sequences isolated from red-billed gull samples to those available in GenBank and the MalAvi database of avian malarial sequences (Bensch et al. Citation2009). For phylogenetic analyses, we included known morphospecies of avian Plasmodium parasites where genetic analyses were accompanied by microscopic examination of blood films (Perkins & Schall Citation2002; Wiersch et al. Citation2005; Beadell et al. Citation2006; Omori et al. Citation2007; Valkiunas et al. Citation2007, Citation2008b), in addition to taxonomically unclassified lineages with greatest BLAST sequence similarity to red-billed gull isolates. Three Plasmodium parasites of primates (P. falciparum and P. vivax from humans, and P. knowlesi from Old World monkeys; Perkins & Schall Citation2002) were included as outgroups. GenBank accession numbers of all sequences included in phylogenetic analyses appear in . Sequences were aligned using Clustal W (Thompson et al. Citation1994), and pairwise nucleotide identities were calculated in BioEdit Sequence Alignment Editor v. 7.0.5.3 (Hall Citation1999).
Figure 2 Maximum likelihood phylogenies of avian Plasmodium lineages and primate P. knowlesi, P. vivax, and P. falciparum outgroups, built using (A) 415 bp of sequence located between Avmal2 F/R nested primers, and (B) 744 bp sequence contigs from the three screening methodologies (RBG1 only). Sequences isolated from red-billed gulls are shown in bold. Taxon labels list parasite morphospecies (when known), followed by the genetic lineage name according to MalAvi database entries (Bensch et al. Citation2009) and GenBank accession numbers. Values are shown for nodes with >50% bootstrap support, and scale bars indicate the number of nucleotide substitutions per site.
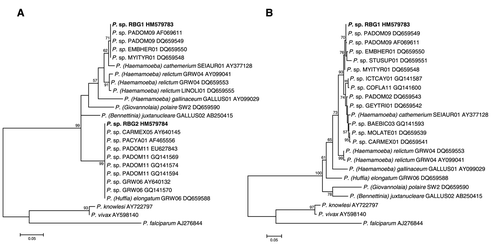
Maximum likelihood phylogenetic trees were built for the 415 bp of sequence located between Avmal2 F/R priming sites, in addition to the 744 bp of sequence resulting from assembly of overlapping products generated by the three parasite screening protocols. Best-fitting models of nucleotide substitution were chosen according to the Akaike information criterion (AIC) with jModelTest v. 0.1.1 (Posada Citation2008). Trees were built in PhyML v. 3.0 (Guindon & Gascuel Citation2003), with a GTR + I+Γ substitution model and empirical base frequencies in both instances, and gamma distribution shape parameter (α) and proportion of invariant sites (Pinv) of α = 0.508 and Pinv = 0.349 for the smaller 415 bp dataset, and α = 0.478 and Pinv = 0.343 for the phylogeny built from the larger cyt b fragment. Node support was estimated from 1000 bootstrap resamplings of input data, and phylogenetic trees were visualized using Tree Explorer in MEGA v. 4.0 (Tamura et al. Citation2007).
Statistical analysis
Condition indices were calculated separately for males and females by regressing body mass on the first component of a principal components analysis of total culmen length, bill depth through angle, and wing length. Since previous studies have shown a significant interannual variation in mean adult body mass of both sexes that is correlated with the availability of the principal food item (Nyctiphanes australis, a coastal planktonic euphausiid; Mills et al. Citation2008), we performed separate regressions for each study year and used the standardized residuals as our measure of condition. This approach allowed us to account for year effects due to differing food availability using a large dataset (females n=1640, males n=1371) rather than the smaller sample screened for malarial infection.
We used multiple linear regression to estimate the influence of malarial infection upon condition of breeding individuals. In addition to infection status (presence/absence), we considered the laying date relative to the seasonal median and the sampling date relative to the first day of laying as explanatory covariates to account for variation in condition associated with breeding chronology.
Logistic and Poisson regression were used to analyse the relationship between malarial infection and reproductive performance in pairs with known infection status of both partners (n=51 nests, 16 with at least one infected partner). We considered the number of eggs laid, brood size, number of chicks fledged (each with a Poisson distribution of errors), and nesting success (defined as fledging at least one chick, binomial distribution) as separate response variables. Since renesting occurred in few of the sampled pairs (six of 51, or 11.8%), we considered only the first nesting attempt within the season. Infection status of each partner or of the parental pair, condition, age, breeding season, laying date, and pair-bond status (first time the pair bred together versus an established pair bond) were considered as explanatory covariates. Our ability to explore these relationships was limited by the small sample size of infected individuals and missing data for some variables. We therefore also compared whether the mean number of eggs laid, brood size, and number of chicks fledged at the first nest differed for parasitized and unparasitized males or females, irrespective of additional covariates.
In all analyses, we avoided replication within the dataset by including a single sample for individuals that were screened for malarial infection in multiple years. If an individual tested positive in a single year, we included information from that season. Otherwise, one sample per individual was chosen based either on field data availability, or by random selection.
Results
Sequence analysis of Plasmodium lineages
Of 271 blood samples screened for avian malaria (from 243 individual adults), 21 samples tested positive for Plasmodium infection (from 19 individuals; two individuals tested positive in both 1994 and 1995). Two Plasmodium cytochrome b haplotypes were identified with DNA sequencing. RBG1 (deposited under GenBank accession HM579783) was isolated from 16 samples (all originating from different individuals) using the HAEMF/HAEMR2 and L15183/H15725 primer pairs. The nested PCR protocol also yielded positive results for these 16 samples, plus an additional five samples that gave negative results using the single-reaction screening methods. The 415 bp of sequence obtained for four of these samples was identical to that of RBG1, whereas a second Plasmodium lineage, RBG2 (GenBank accession HM579784), was isolated from a single blood sample collected in 2001.
The fragment amplified by the nested PCR protocol is not diagnostic to the level of parasite lineage, as sequences isolated from red-billed gulls were identical to multiple genetic lineages of Plasmodium following MalAvi database annotations (Bensch et al. Citation2009; A, RBG1 is identical to lineages PADOM09, EMBHER01, and MYITYR01; RBG2 is identical to CARMEX05, PACYA01, PADOM11, and GRW06). The phylogenetic tree built using this sequence did, however, place RBG2 within a well-supported clade with P. elongatum (A). P. elongatum lineage GRW06 has been isolated from both North and South Island saddlebacks (Philesturnus carunculatus rufusater and P. carunculatus carunculatus, respectively; Alley et al. Citation2010). Additional sequence is therefore needed to determine whether RBG2 also corresponds to this parasite lineage.
In contrast, RBG1 has not previously been reported in the New Zealand avifauna, but shows greatest similarity to lineage PADOM09 isolated from North and South American passerines (MalAvi database), with 100% nucleotide identity across the 744 bp of isolated sequence to GenBank accession DQ659549 and 99.9% identity to AF069611. Although this lineage was originally linked to P. elongatum morphospecies (Escalente et al. Citation1998), more recent analysis instead clusters it with P. cathemerium (GenBank AY377128) within a P. relictum/P. cathemerium clade (Valkiūnas et al. Citation2008b). Our results agree with this latter assessment, as RBG1 falls within the sister group to a clade containing this P. cathemerium sequence (with 98.8% nucleotide identity to RBG1 across the 744 bp fragment), and with these two sister groups forming a clade that is separated from P. relictum with moderate bootstrap support (B). We note that infection with lineage RBG1/PADOM09 cannot be confirmed for two individuals where only the shorter Avmal2F/R sequence fragment was isolated, although infection with RBG1 appears likely given the low infection prevalence and low diversity of recovered sequences.
Prevalence of malarial infections
Across all breeding seasons, 19 of 243 adult individuals (7.8±3.4%) tested positive for Plasmodium infection (). This estimate of total prevalence in red-billed gulls is lower than the New Zealand average of 25.5% reported for non-native passerines (χ2=29.84, df = 1, P=5e−8), but similar to the reported estimate of 9.4% for Christchurch (Fisher's exact test P = 0.617; Tompkins & Gleeson Citation2006). For the 27 individuals sampled in multiple breeding seasons, 22 individuals tested negative in each of two years, one individual tested negative in three successive years, two individuals shifted from uninfected to infected status (both with RBG1), and the remaining two individuals tested positive for RBG1 in both years screened. Estimates of seasonal prevalence varied between 0% and 16.7% (). However, limited sampling within most years resulted in wide 95% confidence intervals surrounding these estimates. We therefore detected no significant difference across seasons (Fisher's exact test P=0.469), and estimates of seasonal prevalence were instead correlated with sampling effort (Spearman's r=0.592, P=0.033).
Table 1 Prevalence of Plasmodium infection across breeding seasons.
The number of males and females infected with Plasmodium was not significantly different (males: 9 of 95 [9.5%], females 10 of 148 [6.8%], χ2=0.545, df = 1, P=0.46). Amongst individuals of known age (banded as chicks, n=117), no infected individuals were found in the youngest cohorts (). Despite this absence of infection in younger birds, there was no significant difference either between the mean age of infected and uninfected individuals (n infected=9, mean±SD 13.78±3.96; n uninfected=108, mean±SD 10.98±6.13; Mann-Whitney U = 358.5, P=0.19), or between infected and uninfected birds grouped by age category (as shown in ; Fisher's exact test P=0.292).
Survivorship c. 1 year post screening (to the beginning of the next breeding season) was known for 212 individuals (87.2%). The fate of three infected birds was unknown (all carried lineage RBG1), while the remaining 16 lived at least 1 year. Survivorship between infected and uninfected individuals does not differ even if the three missing infected individuals are considered dead (i.e. 3 of 19 [15.8%] infected versus 13 of 196 [6.6%] uninfected birds dying within the year, Fisher's exact test, P=0.179).
Relationship of Plasmodium infection with individual condition and reproductive performance
For males, both the sampling date and laying date of their female partners showed significant negative relationships with body condition in linear regression models (laying date β =− 0.028±0.01, P=0.012; sampling date β =− 0.019±0.01, P=0.024). However, as there was a significant negative correlation between laying and sampling dates (r=− 0.420, P=0.0004), the best overall model for males included a composite interaction term for these two variables (). Despite the small sample size of infected individuals, there was a significant negative relationship between infection status and condition of breeding individuals of both sexes (), with semipartial correlations indicating that Plasmodium infection uniquely explained 10.5% of the total variance in condition of females and 11.5% in males. The effect of covariates accounting for laying and/or sampling dates remained significant in regression analyses restricted to uninfected individuals (females: n = 65, sampling date2 β=− 0.0003±0.0001, P=0.046; males: n=60, laying×sampling date β =− 0.0007±0.0002, P=0.006), indicating that the decline in post-laying condition is probably a general phenomenon as described for other species (e.g. Dawson & Bortolotti Citation2000) rather than being driven by the inclusion of infected individuals.
Table 2 Multiple linear regression of condition on Plasmodium infection and breeding chronology covariates.
There was no evidence of a reduction in breeding effort or reproductive success associated with Plasmodium infection. In regression analyses, no combination of infection status with other explanatory covariates showed a significant relationship with any of the measures used as estimates of breeding performance (results not shown). The mean number of eggs laid, brood size, and number of chicks fledged from the first clutch of the season were also not significantly different in parasitized and unparasitized individuals of each sex ().
Table 3 Comparison of reproductive performance in Plasmodium infected and uninfected individuals.
Discussion
The prevalence of malarial infections in red-billed gulls at Kaikoura across all seasons (7.8%) is lower than the New Zealand average in non-native passerine hosts (25.5%), but similar to reports from Christchurch (9.4%), which is consistent with a previously described pattern of decreasing malarial prevalence from north to south (Tompkins & Gleeson Citation2006). This geographic gradient mirrors the distribution and southward spread of the invasive mosquito Culex quinquefasciatus, a known vector of avian malaria in other regions, from its original sites of introduction in Northland and Auckland (Holder et al. Citation1999). Low prevalence in red-billed gulls could also reflect low abundance of mosquito vectors near the Kaikoura breeding colony (Cane & Slaney Citation2008, Citation2009), where gulls spend most of the spring and summer months. It is currently unknown whether infection occurs at the breeding colony itself, during visits to sites with higher vector abundance within the breeding season (e.g. sites of human habitation, garbage dumps), or in the late summer after birds have dispersed from the colony. Recent evidence also suggests a likely role of the endemic and widespread C. pervigilans as an avian malaria vector (Massey et al. Citation2007; Derraik et al. Citation2008). Further work is needed to establish when, where, and by which vector(s) these infections occur to better understand both the infection dynamics in red-billed gulls and the risk of exposure to other endemic species from infected gulls.
Two lines of evidence suggest that the Plasmodium infections detected in this study might correspond to individuals in the chronic stage of infection. First, infections were maintained in two individuals where blood samples were available for consecutive years, confirming that chronic infection with lineage RBG1 occurs in at least some individuals. Second, microscopic inspection of peripheral blood smears resulted in a lower estimate of haemosporidian prevalence of 0.7% for this population of red-billed gulls during the 2007–2008 and 2008–2009 breeding seasons (n = 140; Tompkins et al. Citation2010). The 10-fold higher prevalence estimated from PCR-based assays is probably attributable to the greater sensitivity of this methodology to detect low-level Plasmodium infections (Jarvi et al. Citation2002; Fallon & Ricklefs Citation2008), and is also consistent with observations that wild-caught individuals typically harbour chronic infections characterized by low numbers of circulating parasites (Valkiūnas Citation2005). However, differing prevalence estimates could also reflect temporal or life history dynamics of host-parasite interactions. Although our samples were collected at the same location and similar time of year to those of Tompkins et al. (Citation2010), density-dependent effects on red-billed gull breeding behaviour throughout this period of population decline (Mills et al. Citation2008) and/or seasonal variation in climatic conditions or food availability could affect the abundance of mosquito vectors or the susceptibility of avian hosts. Our limited sampling within individual breeding seasons currently precludes analysis of these factors, and additional data are needed to investigate the temporal dynamics of Plasmodium infection within this population.
The occurrence of chronic infection has potential implications for disease surveillance and risk assessment. Prevalence of infection might be underestimated, since both microscopic and PCR-based screening methodologies detect parasites circulating in peripheral blood and will generate negative test results for individuals where latent infections are sequestered in the liver (Jarvi et al. Citation2002). Additionally, although we found no difference in survivorship of infected and uninfected individuals, sampling of chronically infected birds that have survived the acute stage of infection and acquired premunition against reinfection by the same malarial lineage is uninformative regarding the morbidity and mortality associated with primary Plasmodium infection (Valkiūnas Citation2005; Atkinson Citation2008).
Plasmodium infection was negatively related to post-laying condition of breeding red-billed gulls of both sexes. Support for a general association between haemosporidian parasitism and host condition is mixed, with some studies finding no effect (Smith & Cox Citation1972; Bennet et al. Citation1988; Weatherhead & Bennett Citation1992), and others reporting negative relationships in breeding (Bosch et al. Citation1997; Ots & Hõrak Citation1998; Shutler et al. Citation1999; Dawson & Bortolotti Citation2000; Merino et al. Citation2000; Schrader et al. Citation2003) or migrating birds (Garvin et al. Citation2006; DeGroote & Rodewald Citation2010). Results within a single host species may vary according to study year, geographic location, parasite genus and lineage, infection prevalence and intensity, breeding chronology, gender, and/or host age (e.g. Bosch et al. Citation1997; Ots & Hõrak Citation1998; Dawson & Bortolotti Citation2000; DeGroote & Rodewald Citation2010), underscoring the potential importance of ecological, life history, and spatiotemporal context in interpreting fitness impacts of haematozoan infection in wild populations (Møller Citation1997).
Although Plasmodium infection uniquely explained a significant amount of the total variation in condition of red-billed gulls (10.5% in females and 11.5% in males), the models left most of the overall variance unexplained. In addition to a small sample size of infected individuals, the use of condition indices may have limited our ability to account for a greater proportion of the overall variation in condition. Residuals from regression of mass on measurements of body size may fail to fully account for the effects of structural size or shape (Gosler & Harper Citation2000; Green Citation2001), and the use of additional measures such as pectoral muscle profiles or ultrasonic measurement of pectoral muscle thickness should be considered in future studies (Bolton et al. Citation1991; Lindström et al. Citation2000). Use of a binomial (presence/absence) measure of infection may be less informative than parasitaemia (or infection intensity, traditionally measured as the proportion of parasitized erythrocytes). Additionally, as PCR-based screening methods may preferentially amplify some parasite lineages, leading to an underestimation of the prevalence of mixed infections (Valkiūnas et al. Citation2006, Citation2008a), additive, antagonistic, or synergistic effects among malarial parasites could also account for some of the unexplained variance in breeding condition (e.g. Marzal et al. Citation2008). Combining molecular screening methods with microscopic examination of blood smears, which were unavailable in the current study, is therefore needed to better understand how both parasitaemia and co-infection contribute to the observed relationship between Plasmodium infection and individual condition. The relative contributions of individual immunogenetic variation and infection status of other pathogens of red-billed gulls, including arboviruses (Austin Citation1984; Tompkins et al. Citation2010), Babesia spp. (Tompkins et al. Citation2010), and gastrointestinal helminths (Fredensborg et al. Citation2004) also requires further study.
There was no evidence to support an association between Plasmodium infection and decreased reproductive performance in red-billed gulls, although we note that missing data for some covariates may have limited discriminatory power. Observational studies have generally not provided strong support for a relationship between haemosporidian infection and reproductive performance, leading some authors to suggest that despite the potential severity of primary infections, chronic infections typical of wild-caught individuals might be relatively benign (Shutler et al. Citation1999; Dawson & Bortolotti Citation2001; Bensch et al. Citation2007). In contrast, medication experiments in passerines have demonstrated associations between experimental reduction of Haemoproteus or Plasmodium parasitaemia and increased clutch size, hatching success and/or fledging success (Merino et al. Citation2000; Marzal et al. Citation2005; Knowles et al. Citation2010). Knowles et al. (Citation2010) concluded that even low-intensity Plasmodium infections can reduce reproductive success in blue tits (Cyanistes caeruleus), and might result in significant cumulative effects on lifetime reproductive success in long-lived organisms.
Observational studies and brood manipulation experiments have also shown a positive relationship between reproductive effort and post-breeding parasitaemia in a number of wild populations (e.g. Oppliger et al. Citation1997; Nordling et al. Citation1998; Dawson & Bortolotti Citation2001; Schrader et al. Citation2003). According to resource allocation-based theory, energetic trade-offs occur when limited resources must be apportioned to the competing interests of reproductive effort and immune system function (Gustafsson et al. Citation1994; Sheldon & Verhulst Citation1996; French et al. Citation2009). Accordingly, the positive correlation between reproductive effort and subsequent haemoparasite load is explained by recrudescence of chronic infections triggered by hormonal activity or physiological stress (Atkinson & van Riper Citation1991). Alternatively, individuals investing heavily in reproduction could experience increased susceptibility to novel infections if residual energetic resources are insufficient to mount an effective immune response (Richner et al. Citation1995; Deerenberg et al. Citation1997; Nordling et al. Citation1998). The correlative nature of our study means that the direction and causality of the observed association between Plasmodium infection and host condition cannot be conclusively determined. Monitoring of individuals throughout the breeding season, combined with immunocompetence assays, medication experiments, or experimental manipulation of reproductive effort could help to clarify the mechanisms underlying this relationship.
The consequences of energetic trade-offs to reproductive performance may depend upon the ecological context of infection. In particular, the availability of Nyctiphanes australis, the principal food item of red-billed gulls during the breeding season, may be important as previous work has shown a negative correlation between the proportion of non-breeders within the population and the mean annual availability of N. australis, a positive correlation between the mean body mass of both sexes and this measure of food availability, and a positive correlation between the interannual variation in female body mass and mean clutch size (Mills et al. Citation2008). Whether the cost of managing Plasmodium infection is sufficient to limit reproductive effort in years with poor food availability is therefore of interest, but requires greater depth of sampling within each breeding season than was performed here. Confirmation of whether chronically infected red-billed gulls experience the increased parasitaemia following reproduction seen in other species is also needed, as elevated levels of circulating parasites might increase the risk of Plasmodium transmission if recrudescence of infection coincides with the timing of peak vector activity or dispersal of gulls to areas of increased vector abundance.
In summary, we have confirmed that red-billed gulls infected with Plasmodium have bred at Kaikoura dating back to at least 1992, that chronic infections of lineage RBG1 are maintained over several years in at least some individuals, and that infection is associated with decreased condition in breeding individuals of both sexes. There is currently no evidence that Plasmodium infection is associated with reduced reproductive performance. However, further study involving the ecological context and temporal variation of infection is needed for more rigorous analysis. Finally, identification of the location, timing, and vectors associated with the transmission of avian malaria remains a priority for future research to assess the impact of the acute phase of infection, and the risk of both increased prevalence in red-billed gulls and transmission to other endemic species in relation to mosquito vector distribution and abundance.
Acknowledgements
We are grateful to the large number of people who have helped with fieldwork at Kaikoura over the past 46 years. Funding was provided by a Marsden grant from the Royal Society of New Zealand from 1996–1999 (JAM & AJB), the Royal Ontario Museum Governors Fund and the Natural Sciences and Engineering Research Council of Canada (AJB), and a NSERC PGS-D scholarship (AC).
References
- Alley , MR , Hale , KA , Cash , W , Ha , HJ and Howe , L . 2010 . Concurrent avian malaria and avipox virus infection in translocated South Island saddlebacks (Philesturnus carunculatus carunculatus) . New Zealand Veterinary Journal , 58 : 218 – 223 .
- Altschul , SF , Madden , TL , Schäffer , AA , Zhang , J , Zhang , Z , Miller , W and Lipman , DJ . 1997 . Gapped BLAST and PSI-BLAST: a new generation of protein database search programs . Nucleic Acids Research , 25 : 3389 – 3402 .
- Applegate , JE . 1970 . Population changes in latent avian malaria infections associated with season and corticosterone treatment . Journal of Parasitology , 56 : 439 – 443 .
- Atkinson , CT . 2008 . “ Avian malaria ” . In Parasitic diseases of wild birds , Edited by: Atkinson , CT , Thomas , NJ and Hunter , DB . 35 – 53 . Ames, IA : Wiley-Blackwell .
- Atkinson , CT and van Riper , C III . 1991 . “ Pathogenicity and epizootiology of avian haematozoa: Plasmodium, Leucocytozoon, and Haemoproteus ” . In Bird-parasite interactions: ecology, evolution, and behaviour , Edited by: Loye , JE and Zuk , M . 19 – 48 . Oxford : Oxford University Press .
- Atkinson , CT , Woods , KL , Dusek , RJ , Sileo , LS and Iko , WM . 1995 . Wildlife disease and conservation in Hawaii: pathogenicity of avian malaria (Plasmodium relictum) in experimentally infected Iiwi (Vestiaria coccinea) . Parasitology , 111 ( Suppl. S1 ) : S59 – S69 .
- Austin , FJ . 1984 . Ticks as arbovirus vectors in New Zealand . New Zealand Entomologist , 8 : 105 – 106 .
- Beadell , JS , Ishtiaq , F , Covas , R , Melo , M , Warren , BH , Atkinson , CT , Bensch , S , Graves , GR , Jhala , YV , Peirce , MA , Rahmani , AR , Fonseca , DM and Fleischer , RC . 2006 . Global phylogeographic limits of Hawaii's avian malaria . Proceedings of the Royal Society B: Biological Sciences , 273 : 2935 – 2944 .
- Bennett , GF , Caines , JR and Bishop , MA . 1988 . Influence of blood parasites on the body mass of passeriform birds . Journal of Wildlife Diseases , 24 : 339 – 343 .
- Bennett , GF , Peirce , MA and Ashford , RW . 1993 . Avian haematozoa: mortality and pathogenicity . Journal of Natural History , 27 : 993 – 1001 .
- Bensch , S , Stjernman , M , Hasselquist , D , Östman , Ö , Hansson , B , Westerdahl , H and Pinheiro , RT . 2000 . Host specificity in avian blood parasites: a study of Plasmodium and Haemoproteus mitochondrial DNA amplified from birds . Proceedings of the Royal Society B: Biological Sciences , 267 : 1583 – 1589 .
- Bensch , S , Waldenström , J , Jonzen , N , Westerdahl , H , Hansson , B , Sejberg , D and Hasselquist , D . 2007 . Temporal dynamics and diversity of avian malaria parasites in a single host species . Journal of Animal Ecology , 76 : 112 – 122 .
- Bensch , S , Hellgren , O and Perez-Tris , J . 2009 . MalAvi: a public database of malaria parasites and related haemosporidians in avian hosts based on mitochondrial cytochrome b lineages . Molecular Ecology Resources , 9 : 1353 – 1358 .
- Bolton , M , Monaghan , P and Houston , DC . 1991 . An improved technique for estimating pectoral muscle protein condition from body measurements of live gulls . Ibis , 133 : 264 – 270 .
- Bosch , M , Figuerola , J , Cantos , FJ and Velarde , R . 1997 . Intracolonial differences in the infestation by Haemoproteus lari on yellow-legged gulls Larus cachinnans . Ornis Fennica , 74 : 105 – 112 .
- Cane R , Slaney D 2008 . A field study into the ecology of vectors and vector-borne diseases in New Zealand: vector collection results – year one . Report to Landcare as part of the CDRP on vector ecology. MAF(BNZ). Unpublished report . 31 p.
- Cane R , Slaney D 2009 . A field study into the ecology of vectors and vector-borne diseases in New Zealand: vector collection results – year two . Report to Landcare as part of the CDRP on vector ecology. MAF(BNZ). Unpublished report . 16 p.
- Daszak , P , Cunningham , AA and Hyatt , AD . 2000 . Emerging infectious diseases of wildlife – threats to biodiversity and human health . Science , 287 : 443 – 449 .
- Dawson , RD and Bortolotti , GR . 2000 . Effects of hematozoan parasites on condition and return rates of American kestrels . Auk , 117 : 373 – 380 .
- Dawson , RD and Bortolotti , GR . 2001 . Sex-specific associations between reproductive output and hematozoan parasites of American kestrels . Oecologia , 126 : 193 – 200 .
- Dean , AD and Greenwald , JE . 1995 . Use of filtered pipet tips to elute DNA from agarose gels . Biotechniques , 18 : 980
- Deerenberg , C , Arpanius , V , Daan , S and Bos , N . 1997 . Reproductive effort decreases antibody responsiveness . Proceedings of the Royal Society B: Biological Sciences , 264 : 1021 – 1029 .
- DeGroote , LW and Rodewald , PG . 2010 . Blood parasites in migrating wood-warblers (Parulidae): effects on refueling, energetic condition, and migration timing . Journal of Avian Biology , 41 : 147 – 153 .
- Derraik , JGB , Tompkins , DM , Alley , MR , Holder , P and Atkinson , T . 2008 . Epidemiology of an avian malaria outbreak in a native bird species (Mohoua ochrocephala) in New Zealand . Journal of the Royal Society of New Zealand , 38 : 237 – 242 .
- Escalante , AA , Freeland , DE , Collins , WE and Lal , AA . 1998 . The evolution of primate malaria parasites based on the gene encoding cytochrome b from the linear mitochondrial genome . Proceedings of the National Academy of Sciences of the United States of America , 95 : 8124 – 8129 .
- Fallon , SM and Ricklefs , RE . 2008 . Parasitemia in PCR-detected Plasmodium and Haemoproteus infections in birds . Journal of Avian Biology , 39 : 514 – 522 .
- Fredensborg , BL , Latham , AD and Poulin , R . 2004 . New records of gastrointestinal helminths from the red-billed gull (Larus novaehollandiae scopulinus) . New Zealand Journal of Zoology , 31 : 75 – 80 .
- French , SS , Moore , MC and Demas , GE . 2009 . Ecological immunology: the organism in context . Integrative and Comparative Biology , 49 : 246 – 253 .
- Fridolfsson , AK and Ellegren , H . 1999 . A simple and universal method for molecular sexing of non-ratite birds . Journal of Avian Biology , 30 : 116 – 121 .
- Garvin , MC , Szell , CC and Moore , FR . 2006 . Blood parasites of nearctic-neotropical migrant passerine birds during spring trans-gulf migration: Impact on host body condition . Journal of Parasitology , 92 : 990 – 996 .
- Gosler , AG and Harper , D . 2000 . Assessing the heritability of body condition in birds: a challenge exemplified by the great tit Parus major L. (Aves) . Biological Journal of the Linnean Society , 71 : 103 – 117 .
- Green , AJ . 2001 . Mass/length residuals: measures of body condition or generators of spurious results? . Ecology , 82 : 1473 – 1483 .
- Guindon , S and Gascuel , O . 2003 . A simple, fast, and accurate algorithm to estimate large phylogenies by maximum likelihood . Systematic Biology , 52 : 696 – 704 .
- Gurr , L and Kinsky , FC . 1965 . The distribution of breeding colonies and status of the red-billed gull in New Zealand and its outlying islands . Notornis , 12 : 223 – 240 .
- Gustafsson , L , Nordling , D , Andersson , MS , Sheldon , BC and Qvarnström , A . 1994 . Infectious diseases, reproductive effort and the cost of reproduction in birds . Philosophical Transactions of the Royal Society of London B: Biological Sciences , 346 : 323 – 331 .
- Hall , TA . 1999 . BioEdit: a user-friendly biological sequence alignment editor and analysis program for windows 95/98/NT . Nucleic Acids Symposium Series , 41 : 95 – 98 .
- Holder , P , Brown , G and Bullians , M . 1999 . The mosquitoes of New Zealand and their animal disease significance . Surveillance , 26 : 12 – 15 .
- Jarvi , SI , Schultz , JJ and Atkinson , CT . 2002 . PCR diagnostics underestimate the prevalence of avian malaria (Plasmodium relictum) in experimentally-infected passerines . Journal of Parasitology , 88 : 153 – 158 .
- Kalendar , R , Lee , D and Schulman , AH . 2009 . FastPCR software for PCR primer and probe design and repeat search. Genes . Genomes and Genomics , 3 ( Special Issue 1 ) : 1 – 14 .
- Knowles , SCL , Palinauskas , V and Sheldon , BC . 2010 . Chronic malaria infections increase family inequalities and reduce parental fitness: experimental evidence from a wild bird population . Journal of Evolutionary Biology , 23 : 557 – 569 .
- Lindström , A , Kvist , A , Piersma , T , Dekinga , A and Dietz , MW . 2000 . Avian pectoral muscle size rapidly tracks body mass changes during flight, fasting and fuelling . Journal of Experimental Biology , 203 : 913 – 919 .
- Marzal , A , de Lope , F , Navarro , C and Møller , AP . 2005 . Malarial parasites decrease reproductive success: an experimental study in a passerine bird . Oecologia , 142 : 541 – 545 .
- Marzal , A , Bensch , S , Reviriego , M , Balbontin , J and de Lope , F . 2008 . Effects of malaria double infection in birds: one plus one is not two . Journal of Evolutionary Biology , 21 : 979 – 987 .
- Massey , B , Gleeson , DM , Slaney , D and Tompkins , DM . 2007 . PCR detection of Plasmodium and blood meal identification in a native New Zealand mosquito . Journal of Vector Ecology , 32 : 154 – 156 .
- Merino , S , Moreno , J , Sanz , JJ and Arriero , E . 2000 . Are avian blood parasites pathogenic in the wild? A medication experiment in blue tits (Parus caeruleus) . Proceedings of the Royal Society of London B: Biological Sciences , 267 : 2507 – 2510 .
- Mills , JA . 1971 . Sexing red-billed gulls from standard measurements . New Zealand Journal of Marine and Freshwater Research , 5 : 326 – 328 .
- Mills , JA . 1973 . The influence of age and pair-bond on the breeding biology of the red-billed gull Larus novaehollandiae scopulinus . Journal of Animal Ecology , 42 : 147 – 162 .
- Mills , JA , Yarrall , JW and Mills , DA . 1996 . “ Causes and consequences of mate fidelity in red-billed gulls ” . In Partnerships in birds: the study of monogamy , Edited by: Black , JM . 286 – 304 . New York : Oxford University Press .
- Mills , JA , Yarrall , JW , Bradford-Grieve , JM , Uddstrom , MJ , Renwick , JA and Merilä , J . 2008 . The impact of climate fluctuation on food availability and reproductive performance of the planktivorous red-billed gull Larus novaehollandiae scopulinus . Journal of Animal Ecology , 77 : 1129 – 1142 .
- Miskelly , CM , Dowding , JE , Elliott , GP , Hitchmough , RA , Powlesland , RG , Robertson , HA , Sagar , PM , Scofield , RP and Taylor , GA . 2008 . Conservation status of New Zealand birds, 2008 . Notornis , 55 : 117 – 135 .
- Møller , AP . 1997 . “ Parasitism and the evolution of host life history ” . In Host-parasite evolution: general principals and avian models , Edited by: Clayton , DH and Moore , J . 105 – 127 . Oxford : Oxford University Press .
- Nordling , D , Andersson , M , Zohari , S and Gustafsson , L . 1998 . Reproductive effort reduces specific immune response and parasite resistance . Proceedings of the Royal Society of London B: Biological Sciences , 265 : 1291 – 1298 .
- Omori , S , Sato , Y , Isobe , T , Yukawa , M and Murata , K . 2007 . Complete nucleotide sequences of the mitochondrial genomes of two avian malaria protozoa, Plasmodium gallinaceum and Plasmodium juxtanucleare . Parasitology Research , 100 : 661 – 664 .
- Oppliger , A , Christe , P and Richner , H . 1997 . Clutch size and malarial parasites in female great tits . Behavioral Ecology , 8 : 148 – 152 .
- Ots , I and Hõrak , P . 1998 . Health impact of blood parasites in breeding great tits . Oecologia , 116 : 441 – 448 .
- Palinauskas , V , Valkiūnas , G , Bolshakov , VC and Bensch , S . 2008 . Plasmodium relictum (lineage P-SGS1): effects on experimentally infected passerine birds . Experimental Parasitology , 120 : 372 – 380 .
- Perkins , SL and Schall , JJ . 2002 . A molecular phylogeny of malarial parasites recovered from cytochrome b gene sequences . Journal of Parasitology , 88 : 972 – 978 .
- Posada , D . 2008 . jModelTest: phylogenetic model averaging . Molecular Biology and Evolution , 25 : 1253 – 1256 .
- Richner , H , Christe , P and Opplinger , A . 1995 . Paternal investment affects prevalence of malaria . Proceedings of the National Academy of Sciences of the United States of America , 92 : 1192 – 1194 .
- Schrader , MS , Walters , EL , James , FC and Greiner , EC . 2003 . Seasonal prevalence of a haematozoan parasite of red-bellied woodpeckers (Melanerpes carolinus) and its association with host condition and overwinter survival . Auk , 120 : 130 – 137 .
- Sheldon , BC and Verhulst , S . 1996 . Ecological immunology: costly parasite defences and trade-offs in evolutionary ecology . Trends in Ecology and Evolution , 11 : 317 – 321 .
- Shutler , D , Clark , RG , Rutherford , ST and Mullie , A . 1999 . Blood parasites, clutch volume, and condition of gadwalls and mallards . Journal of Avian Biology , 30 : 295 – 301 .
- Smith , VW and Cox , FEG . 1972 . Blood parasites and weights of palaearctic migrants in central Nigeria . Ibis , 114 : 105 – 106 .
- Szymanski , MM and Lovette , IJ . 2005 . High lineage diversity and host sharing of malarial parasites in a local avian assemblage . Journal of Parasitology , 91 : 768 – 774 .
- Tamura , K , Dudley , J , Nei , M and Kumar , S . 2007 . MEGA4: Molecular Evolutionary Genetics Analysis (MEGA) software version 4.0 . Molecular Biology and Evolution , 24 : 1596 – 1599 .
- Thompson , JD , Higgins , DG and Gibson , TJ . 1994 . CLUSTAL W: improving the sensitivity of progressive multiple sequence alignment through sequence weighting, position-specific gap penalties and weight matrix choice . Nucleic Acids Research , 22 : 4673 – 4680 .
- Tompkins , DM and Gleeson , DM . 2006 . Relationship between avian malaria distribution and an exotic invasive mosquito in New Zealand . Journal of the Royal Society of New Zealand , 36 : 51 – 62 .
- Tompkins , D , Massey , B , Sturrock , H and Gleeson , D . 2008 . Avian malaria in native New Zealand birds? . Kararehe Kino , 13 : 8 – 9 .
- Tompkins D , Castro I , Barraclough R , Jakob-Hoff R 2010 . A field study into the ecology of vectors and vector-borne diseases in New Zealand. MAF(BNZ) . Unpublished report . 39 p.
- Valkiūnas G 2005 . Avian malaria parasites and other haemosporidia . Boca Raton, FL , CRC Press . 932 p.
- Valkiūnas , G , Bensch , S , Iezhova , TA , Križanauskienė , A , Hellgren , O and Bolshakov , CV . 2006 . Nested cytochrome b polymerase chain reaction diagnostics underestimate mixed infections of avian blood haemosporidian parasites: microscopy is still essential . Journal of Parasitology , 92 : 418 – 422 .
- Valkiūnas , G , Zehtindjiev , P , Hellgren , O , Ilieva , M , Iezhova , TA and Bensch , S . 2007 . Linkage between mitochondrial cytochrome b lineages and morphospecies of two avian malarial parasites, with a description of Plasmodium (Novyella) ashfordi sp. nov . Parasitology Research , 100 : 1311 – 1322 .
- Valkiūnas , G , Iezhova , TA , Križanauskienė , A , Palinauskas , V , Sehgal , RNM and Bensch , S . 2008a . A comparative analysis of microscopy and PCR-based detection methods for blood parasites . Journal of Parasitology , 94 : 1395 – 1401 .
- Valkiūnas , G , Zehtindjiev , P , Dimitrov , D , Križanauskienė , A , Iezhova , TA and Bensch , S . 2008b . Polymerase chain reaction-based identification of Plasmodium (Huffia) elongatum, with remarks on species identity of haemosporidian lineages deposited in GenBank . Parasitology Research , 102 : 1185 – 1193 .
- van Riper , III C , van Riper , SG , Goff , ML and Laird , M . 1986 . The epizootiology and ecological significance of malaria in Hawaiian land birds . Ecological Monographs , 56 : 327 – 344 .
- van Riper III C , Atkinson CT , Seed TM 1994 . Plasmodia of Birds . In: Kreier JP . Parasitic protozoa . Volume 7 . 2nd edition . San Diego, CA , Academic Press . Pp. 73 – 140 .
- Waldenström , J , Bensch , S , Hasselquist , D and Östman , O . 2004 . A new nested polymerase chain reaction method very efficient in detecting Plasmodium and Haemoproteus infections from avian blood . Journal of Parasitology , 90 : 191 – 194 .
- Weatherhead , PJ and Bennett , GF . 1992 . Ecology of parasitism of brown-headed cowbirds by hematozoa . Canadian Journal of Zoology , 70 : 1 – 7 .
- Wiersch , SC , Maier , WA and Kampen , H . 2005 . Plasmodium (Haemamoeba) cathemerium gene sequences for phylogenetic analysis of malaria parasites . Parasitology Research , 96 : 90 – 94 .
- Wilson , K-J . 2007 . “ Gulls, skuas and terns ” . In The state of New Zealand's birds, 2006. Special report – New Zealand's seabirds , Edited by: Wilson , K-J . Pp. 12 Wellington : Ornithological Society of New Zealand .
- Wobeser , GA . 2008 . “ Parasitism: costs and effects ” . In Parasitic diseases of wild birds , Edited by: Atkinson , CT , Thomas , NJ and Hunter , DB . 3 – 12 . Ames, IA : Wiley-Blackwell .