Abstract
The volcanic cones of Auckland are distinctive landmarks of that city, currently realized as an ‘archipelago’ of grassy reserved hills. Most of these cones were modified as hill forts during former occupation by Māori. Here, we review the Auckland volcanic reserve network and survey the current groundcover vegetation and soils of four large representative examples. Forty-eight reserves currently occur on remnant volcanic features. As well as two inshore island reserves (2372 ha), the other 46 reserves total 1241 ha. Most of the volcanic reserves support exotic grassland with rare forest patches. Groundcover vegetation follows a topographic sequence from flatter, fertile sites dominated by Lolium perenne, Trifolium repens and Pennisetum clandestinum, to moderately steep sites dominated by a diverse range of exotic grasses, to steep, lower fertility sites dominated by Microlaena stipoides. Pennisetum clandestinum is a major component of groundcover vegetation on many sites across all slope classes, its abundance varying in relation to soil N levels rather than to changes in slope. Soil analyses indicate that soils are nutrient-rich with high soil carbon and phosphorus. Future management should restore some native forest and introduce more herbaceous native species where low groundcover vegetation is desirable. Decreasing soil nutrient levels through strategies such as cessation of fertilizer application may assist these processes.
Introduction
The volcanic cones of Auckland are distinctive landmarks of that city, for the most part currently realized as an ‘archipelago’ of grassy hills (all <200 m above sea level) that rise above the urban matrix. These cones are part of the Auckland volcanic field which is a dormant basaltic volcanic field underlying much of metropolitan Auckland. Fifty-three eruption centres ranging in age from approximately 250,000 to 600 years since eruption have been identified in this field (Searle & Mayhill Citation1981; Homer et al. Citation2000; Hayward et al. Citation2011a,Citationb; Lindsay et al. 2011).
As well as being important geological remnants of Auckland's volcanic character and centres of urban biodiversity and recreation, most of the cones were long occupied by Māori in the centuries prior to European arrival in New Zealand (Hayward Citation1983), and so have strong cultural and archaeological significance. During their occupation, the Māori inhabitants modified the slopes and summits of the cones by earthworks to create hill forts (pā) composed of a series of terraces, over-steepened slopes, ditches and pits (Fairfield Citation1941; Fox Citation1984). These cultural earthworks are now prominent features of the cone slopes. Further modification of many of the cones occurred in the twentieth century through quarrying for scoria, construction of urban water reservoirs, and emplacement of defensive positions during the Second World War. However, long-held concerns for the preservation of the cones (e.g. Fowlds Citation1928; Golson Citation1957) and recognition of their natural, cultural and recreational values to the residents of Auckland have now halted further large-scale human modification, and they have been proposed collectively as a World Heritage Site (Department of Conservation Citation2006).
The dominant approach to management of these grasslands was by grazing with sheep (Ovis aries) in the early decades of the twentieth century, replaced by cattle (Bos taurus) in the last few decades due to the vulnerability of sheep to attacks from domestic dogs. In the past 20 years, there have been reductions in cattle stocking rates on these cones due largely to concerns about erosion damage to archaeological features (Trimble & Mendel Citation1995; Jones Citation1998, Citation2007; Dodd et al. Citation2011). Grazing is also viewed as inappropriate by some Māori descendants of the original inhabitants (Paterson Citation2009), so its type, extent and even continuation are therefore in question. However, concerns for the potential build-up of flammable biomass in these grasslands and increasing rates of woody weed colonization are further issues that have arisen with a decline in grazing intensity. Woody plants also obscure and disrupt archaeological earthworks (Jones Citation2007) and incursions of these have been generally managed by manual removal and herbicide use. However, the urban context of the cones means that the range of herbicide products that can be applied is restricted by local public safety policies.
The vegetation of Auckland is notable globally for its high number of naturalized species (Esler Citation1987). Consistent with this, the plant composition on the cone reserves is clearly dominated by introduced species, many of which are recognized weeds (Esler Citation1988). In particular, following the introduction of Pennisetum clandestinum into New Zealand in the 1920s (Percival Citation1979), this C4 grass of African origin has spread widely in Auckland and has become abundant on the volcanic cones (Esler & Esler Citation2010). It is unclear how much of the cones are now covered by P. clandestinum and other weedy species, and whether native species continue to contribute to the current cone vegetation, or have been lost.
Management of these reserves is complex because it seeks to balance a range of different values: cultural, historical, environmental, recreational, and for biodiversity. There is ongoing debate on what types of vegetative cover will help achieve the multiple goals related to these values, and how such vegetation types, once defined, should be managed (e.g. Paterson Citation2009). The objectives of this paper are to review the extent and vegetation character of the Auckland volcanic reserve network, survey the current groundcover vegetation and soils on four of the most prominent volcanic cones of Auckland, analyse relationships among these components, and suggest options for the future management of the volcanic cone reserves.
Methods
Study areas
We collated information on Auckland green space reserves to identify all such reserves that directly encompass volcanic field features, and noted total area, major vegetation cover types, and the management agency responsible. Following this, we selected four of the largest reserves on which to carry out the vegetation and soil survey. These were Maungakiekie (One Tree Hill), Maungawhau (Mt Eden), Maungarei (Mt Wellington) and Ohinerangi (Mt Hobson) (). All these cones were the sites of former hill forts with slopes extensively reshaped by Māori earthworks.
The cone of Maungakiekie (183 m asl) is protected within Cornwall Park (172 ha) and the adjoining One Tree Hill Domain (48 ha), which together form the largest continuous area of green space within Auckland city. Maungakiekie probably erupted >28,500 years ago (Lindsay et al. Citation2011) with three craters and extensive lava flows. The Maungakiekie reserves are mostly grassland with scattered trees and are currently grazed by sheep (approximately 13 stock units/ha), with 300 kg/ha superphosphate applied every second year (Dodd et al. Citation2011).
Maungawhau is the highest volcanic cone of the Auckland volcanic field at 196 m asl, erupting 28,000 years ago (Lindsay et al. Citation2011). It is encompassed by the 28 ha Mt Eden Domain which is dominated by grassland on its upper slopes but a developing forest on its lower slopes. The grasslands of the reserve were fertilized and grazed by cattle for several decades, but have not been fertilized since c. 1990 and the cattle were removed in 2009 (Dodd et al. Citation2011).
Maungarei (135 m asl) is one of the youngest cones of the Auckland volcanic field, erupting only 10,000 years ago (Lindsay et al. Citation2011). The reserve that includes this cone is the Mt Wellington Domain (27 ha) and is almost completely grassland. It has been grazed by cattle at low intensity for several decades (six stock units/ha) and lower flat areas are regularly fertilized with phosphorus and sulphur by ground spreader (Dodd et al. Citation2011).
The fourth cone used in the survey was Ohinerangi (143 m asl) which is encompassed by the 14 ha Mt Hobson Domain. The cone was formed by eruption which from stratigraphic evidence also occurred >28,500 years ago (Lindsay et al. Citation2011). Again, the predominant vegetation is grassland, although there is a small area of trees on the north-eastern slopes. The reserve has had a varied management history including both grazing and fertilizer application, but has most recently been grazed by cattle only (seven stock units/ha) and without annual fertilizer additions since c. 1990 (Dodd et al. Citation2011).
Vegetation and soil survey
We undertook a vegetation and soil survey in November–December 2006 on the four volcanic cones mentioned above. A total of 70 sites on these cones were identified by a stratified random selection process which distributed them equally across seven categories of slope and aspect (Briggs & Burns Citation2007).
Soil samples of 10×25 mm-diameter cores to 75 mm depth were taken from each site, except where it was inappropriate to do so after taking archaeological advice (five sites excluded). The samples were analysed for pH, total C, total N, C/N ratio, Olsen P, phosphate retention, Ca, Mg, K, Na, cation exchange capacity (CEC) and base saturation using standard methods (Blakemore et al. Citation1987).
At each site, we surveyed five 1×1 m plots, each 1 m apart on a line transect for plant composition and abundance. In each plot, slope was categorized using a clinometer into one of four categories: flat to gentle = < 10°; moderate = 10°–20°; steep = 20°–35°; and very steep = > 35°, sward height was measured to the uppermost leaf blade (as a proxy for grazing intensity), and the identity and cover classes of the vascular plant taxa present were recorded. Most taxa were identified to species, although a few taxa were identified only to genus when identification to species was uncertain (e.g. Rytidosperma spp., Bromus spp.). The cover of each taxon was estimated within semi-quantitative cover classes (i.e.+= <1%; 1 = 1%–5%; 2 = > 5%–25%; 3 = > 25%–50%; 4 = > 50%–75%; 5 = > 75%–95%; 6 = > 95%–100%). Cover of bare ground, dung and rock were assessed on the same scale. Cover of overhead tree canopy was visually assessed. The cover class scores for each species in each of the five plots at a site were averaged using cover class centroids (i.e. 0.5, 3, 15, 37.5, 62.5, 85 and 97.5) to obtain a single mean cover score estimate for each species at a site.
Statistical analysis
Plant community composition was examined using classification and ordination. Data used were mean cover score for each species at a site, square root transformed to reduce the influence of abundant species and take into account rarer species. A cluster analysis was used to aggregate the sites into discrete vegetation units based on group-average linking on Bray-Curtis similarities. Ordination was then applied to the same dataset using non-metric multidimensional scaling (nMDS, Clarke Citation1993) as implemented in the program Primer 6.1.2 (Plymouth Marine Laboratory, UK). A two-dimensional scatter plot was constructed from the first two ordination axes of the nMDS with each point representing the community structure of a site. Soils and environmental data (including tree canopy cover, sward height, rock and dung cover, aspect and slope) were compiled by vegetation unit and, as well, relationships with the first two ordination axes explored using Pearson correlation coefficients and ANOVA within IBM SPSS version 19. Soil properties were also analysed with ANOVA, using cone and topographic strata as factors.
Results
Extent of volcanic cone reserve network
Forty-eight parcels of reserved land currently occur on remnants of the Auckland volcanic field () and are almost all managed by Auckland Council or the New Zealand Department of Conservation (often under co-management agreements with local Māori). Cornwall Park is managed by the Cornwall Park Trust Board. The reserves include the islands of Rangitoto (2311 ha) and Motukorea (61 ha; Brown's Island). The other 46 non-island reserves on the Auckland mainland total approximately 1241 ha and are scattered throughout the urban matrix. They provide a substantial proportion of the current green space network within the city. As well, two further areas covering another 237 ha (Puketutu Island and Highbrook Park) are currently administered by trusts with agreements to vest these lands as reserves with Auckland Council in the future.
Apart from Rangitoto Island (forested), the large majority of the volcanic reserves support open grassland with relatively rare patches of native and exotic trees. The few exceptions are the 0.7 ha Withiel Thomas Reserve (Smale & Gardner Citation1999), a 0.15 ha stand of native trees on the Otuataua Stonefields reserve (Cameron Citation1999), approximately 4 ha of restored forest planted in the 1940s at Mt Smart Domain (Auckland Regional Council Citation2010), areas of regenerating scrub and weedy forest on the perimeter reserves of Orakei Basin (approximately 9.8 ha) and Tuff Crater (approximately 10.4 ha), and scrub on the Wiri Lava Cave Scientific Reserve (1.5 ha). These mean that only approximately 2% of the area within these reserves (excluding Rangitoto) has some form of woody cover.
Groundcover vegetation and soils
Over the 70 sites surveyed, we recorded a total of 89 vascular plant taxa. The majority of these were exotic plant species, with only seven native species. Of these native species, only four were endemic. The four most abundant and widely distributed plant species were P. clandestinum (mean site cover = 12.7%, occurring in 64% of sites), Microlaena stipoides (11.6%, 69%), Lolium perenne (10.3%, 90%) and Trifolium repens (10.2%, 87%).
The classification of community composition indicated eight distinct groundcover vegetation units (, ). These were (following the naming criteria of Atkinson [Citation1985]):
L. perenne–T. repens–P. clandestinum grassland (32 sites).
P. clandestinum–T. repens grassland (three sites).
Bothriochloa macra–Festuca rubra grassland (two sites).
Arrhenatherum elatius–(Rytidosperma sp.Footnote1) grassland (two sites).
Stenotaphrum secundatum–P. clandestinum grassland (four sites).
M. stipoides–(Dactylis glomerata–P. clandestinum) grassland (22 sites).
M. stipoides–Viola odorata–Tradescantia fluminensis–Helix hedera grassland (two sites).
M. stipoides grassland (three sites).
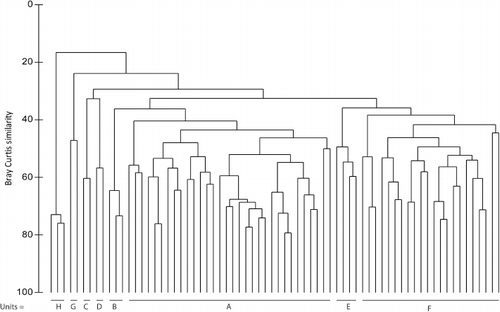
Table 1 Mean % cover scores for dominant species in each of the eight recognized vegetation units (A–H, described in text) on four Auckland volcanic cones: Maungakiekie, Maungawhau, Maungarei and Ohinerangi (means <1% omitted).
provides the mean cover percentage of the dominant species in each of these units. The nMDS ordination of the variation in site composition shows mostly distinct separation among these units ().
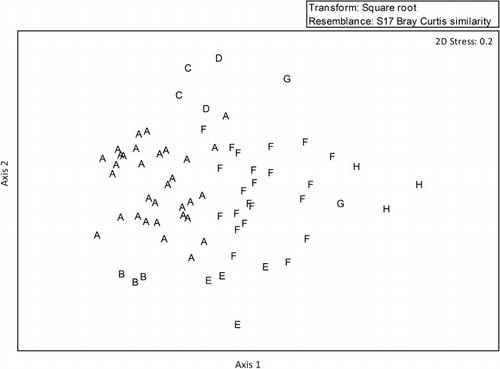
Three of the most common vegetation units (i.e. A, F, H) are segregated along axis 1 () and this sequence describes most of the compositional change on the cones. The axis generally follows a topographic gradient from flat and gently sloped sites on the left-hand side of the axis, with steep and very steep sites on the right-hand side (). Percentage cover of bare soil increases along this axis (), suggesting that erosion is most common on steeper slopes. As well, soil pH is positively correlated with axis 1 (), rising from approximately 5.5 to 7 from flat to steep sites. The high correlations of axis 1 with increasing sward height and tree cover and reducing dung cover () also suggest this axis reflects changes from high to low grazing intensity, and increased shading from trees. The open, flat sites with somewhat acidic soils and higher grazing intensity on the left of the axis are generally covered by P. clandestinum, L. perenne, T. repens, Plantago lanceolata and Agrostis capillaris, whereas the more shaded, steeper sites with low grazing intensity and more neutral soils on the right of the axis support swards dominated by M. stipoides, Dactylis glomerata and Holcus lanatus (). Microlaena stipoides, in particular, is highly correlated with tree cover and forms almost pure stands under shade ().
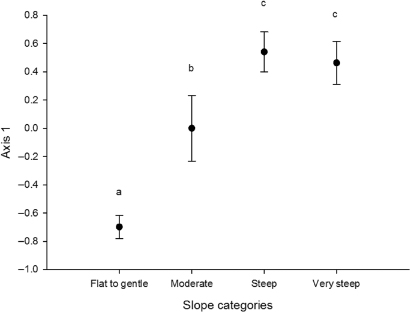
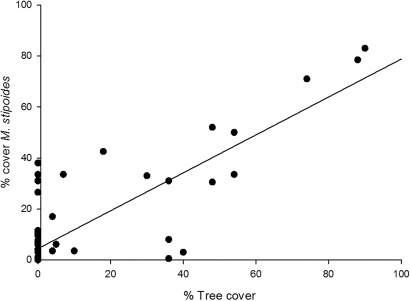
Table 2 Pearson correlation coefficients for environmental variables with first two nMDS ordination axes with significant results.
In contrast to axis 1, axis 2 is not correlated with many environmental variables () and does not differ significantly with slope category (ANOVA, F = 0.602, P=0.6). It is strongly but negatively correlated, however, with soil factors, particularly total C, total N, and K (). This implies that vegetation units that occur low on axis 2 (i.e. B and E, ) have soils with relatively high levels of C, N and K, and vegetation units that occur high on axis 2 (i.e. C and D, ) have soils with low levels of these elements. Vegetation units B and E have high abundances of P. clandestinum () and the abundance of this species shows a strong positive association with soil N content () but not with slope (ANOVA, F = 1.86, P=0.14) or aspect (ANOVA, F = 1.12, P=0.35). Units C and D, occurring on more infertile soils, are dominated by Bothriochloa macra, Festuca rubra, Arrhenatherum elatius, Paspalum dilatatum, Rytidosperma sp. and Sporobolus africanus (). These soil differences across axis 2 can occur on a single cone; but they are not evenly spread among the cones with soils on Maungarei, in particular, scoring low on axis 2 (suggesting relatively high soil N) and soils on Maungakiekie scoring high (suggesting relatively low soil N) ().
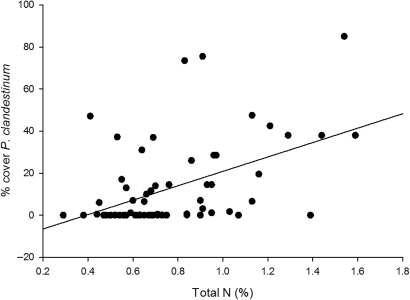
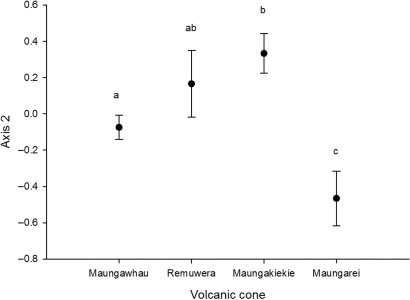
Soil analyses of volcanic cone sites showed that they were generally nutrient-rich with large, active organic pools and generally high levels of P () compared with most natural soils in New Zealand (Blakemore et al. Citation1987). Maungawhau and Maungarei in particular have high soil organic matter and soil N, but by contrast Maungakiekie had the highest Olsen P levels (). Flat and gently sloped sites tended to have higher levels of C, N and P in their soils than sloping sites () and northerly slopes had higher levels of Olsen P than southerly slopes ().
Table 3 Differences in soil characteristics among volcanic cones, aspect and slope categories on four volcanic cones in Auckland: Maungakiekie, Maungawhau, Maungarei and Ohinerangi. Significantly different values (P<0.05) among categories are indicated by different lower case letters.
Discussion
Before Māori occupation of the Auckland isthmus in the fourteenth century (Hayward Citation1983), podocarp-hardwood forest dominated by Metrosideros spp. and other hardwoods grew on and around the Auckland volcanic cones as evidenced by palynological investigations (Horrocks et al. Citation2002, Citation2005a,Citationb). Little of this lava-field forest remains (Millener Citation1979; Cranwell Citation1981), although fragments still occur near Maungawhau (Esler Citation1991; Cameron Citation1999; Smale & Gardner Citation1999) and on the Otuataua Stonefields Reserve (Cameron Citation1991, Citation1999). Wilson (Citation1927), Millener (Citation1979) and Cranwell (Citation1981) also described native trees, ferns, herbs and grasses characteristic of this original vegetation from the Maungarei lava-field, which has subsequently been quarried and lost.
Once Māori arrived and settled in large numbers on the cones, it seems likely that these were almost totally cleared of tall vegetation by burning, and largely supported native grasslands dominated by Microlaena stipoides and Rytidosperma spp. over the period of their occupation (Esler Citation2004). However, abandonment of these cones by Māori in the early nineteenth century as a result of intertribal conflict (Hayward Citation1983) was probably responsible for an abundance of Pteridium esculentum fernland and patches of Leptospermum scoparium scrub on the volcanic cones observed at the time of European settlement (Esler Citation1991, Citation2004). By 1870, European settlers had cleared almost all remaining native vegetation from the Auckland isthmus, including the cones, leading to the observation of Kirk (Citation1870) that ‘the scoria cones of the Isthmus have become covered with a dense sward of introduced grasses and small forage plants, amongst which a few native plants … still maintain their existence’. Early photographs of Mt Eden and Mt Albert show an almost complete absence of trees. Since then, although more trees have been established on the cones, largely through planting, the volcanic cone reserves have been predominantly maintained as grasslands of introduced species (Esler Citation2004; this study).
Our broad analysis of the current vegetation cover of these reserves confirmed that, apart from Rangitoto Island, they were almost all still covered by grasslands. Although this grassland vegetation type is recommended for protective cover of archaeological earthworks (Jones Citation2007), the area of the reserves that represented the primeval lava forest cover of the Auckland volcanic field was particularly low, approximately 2% (Smale & Gardner Citation1999; Cameron Citation1999), and ‘not sufficient representation of this once more widespread unusual forest type on the Auckland isthmus’ (Cameron Citation1999). Increasing the area of such forest on these reserves by ecological restoration techniques on sites that would least compromise archaeological values would undoubtedly increase the biodiversity and historic values of these reserves.
The terrain of the reserves includes manually steepened slopes and flattened areas that are consistent with features of other historic earthworks or hill forts elsewhere (Aust et al. Citation2003; Payne et al. Citation2006). However, such over-steepened earthen slopes are generally vulnerable to soil erosion (Jones Citation1998) and colonization by burrowing animals such as rabbits (Aust et al. Citation2003; Rimmington Citation2004; Dunwell & Trout Citation1999). Monitoring of erosion of experimental earthworks and sedimentation in ditches on earthworks constructed in modern times indicate that, although earthwork form becomes muted in only a few years, the quantity and quality of the vegetative cover can have strong effects in slowing feature degradation such as by erosion (Bell et al. Citation1996).
As expected, the plant composition of the volcanic cone reserve groundcover was strongly dominated by exotic species. Although only seven (8%) native species were encountered, out of a total of 89, two were still abundant and widespread (M. stipoides and A. novae-zelandiae; ) and offer possible opportunities for augmentation. Increasing the abundance of other uncommon native species on the cones (e.g. Muehlenbeckia complexa, Pteridium esculentum, Paesia scaberula and Dichondra repens) or introducing currently absent low-growing native species on to the volcanic cones (e.g. Phormium cookianum and Metrosideros perforata [Jones Citation2007]) should be considered to increase the biodiversity values of the groundcover.
Compared with our survey from within a limited number of survey sites, Esler & Esler (Citation2011) list 140 vascular plant species from a more comprehensive survey of the grassland flora of Maungawhau. This list is also highly dominated by exotic species, with only 10 (7%) native species identified.
Groundcover vegetation composition was clearly differentiated along a gradient that was characterized by topographic influences on tree cover, grazing intensity, erosion and soil chemistry. There are abundant examples in the New Zealand pastoral ecology literature illustrating similar patterns. Slope and aspect in low-altitude pastoral steeplands influence soil moisture balance (Bircham & Gillingham Citation1986), soil temperature (Gillingham & Bell Citation1977; Radcliffe Citation1981) and soil nutrient distribution (via animal transfer effects, Gillingham & During Citation1973). These factors in turn influence species composition and net primary productivity (Grant & Brock Citation1974; Ledgard et al. Citation1982; Lambert et al. Citation1986). Lambert et al. (Citation1986) concluded that desired changes in pasture composition require changes in underlying environmental conditions, e.g. fertility, rather than simply seeding in new species, and this has been borne out in practice (e.g. Webby et al. Citation1990).
The dominant differentiation of groundcover vegetation by slope on the volcanic cone reserves also suggests that management could be varied by broad slope classes (flat and gently sloping sites should be managed differently from steeper slopes). As well, there were variations in soil fertility by volcanic cone, with the youngest volcanic cone sampled (Maungarei, Lindsay et al. Citation2011) having soils with the highest N levels. Cone age would also provide a context within which to vary management action.
Many of the exotic pasture grasses and legumes that are currently dominant on the volcanic cones (i.e. L. perenne, P. clandestinum and T. repens) are generally well adapted to high fertility soils. The soil survey demonstrated that historic fertilizer application on the cones has maintained relatively high Olsen P levels. The abundance of N-fixing legumes such as T. repens will also contribute to N inputs that are recycled by grazing animals, thereby also increasing soil N fertility, particularly on areas that animals frequent. This situation tends to maintain the competitive advantage of exotic species with high growth rates on flatter sites. In contrast, indigenous species are generally regarded as having slow growth rates and being better adapted to low fertility soils (Craine et al. Citation2006). Alleviating this inherent resistance to vegetation change will require cessation of fertilizer input where it is still occurring. For example, P. clandestinum abundance is directly related to soil N content (), so allowing soil fertility to ‘relax’ to natural levels should decrease P. clandestinum vigour and possibly abundance on the cones. However, fertility is likely to take a long time (possibly decades) to decline substantially. Possible actions to accelerate reductions in soil fertility could involve N immobilization strategies (Perry et al. Citation2010), such as addition of C to soils or biomass removal, and should be investigated.
Our vegetation survey indicated that the native grass M. stipoides was still widespread on the cone reserves at the time of the survey despite P. clandestinum invasion (Esler Citation2004; Esler & Esler Citation2010) but was significantly more abundant on medium and steep slopes than on flat sites (F = 4.15, P < 0.01), although this may have also reflected its close association with tree cover which is more common on slopes (). The association of M. stipoides with tree cover and its tolerance of infertile soils have been noted elsewhere in New Zealand (Allen et al. Citation1995; Smale et al. Citation1997). Microlaena stipoides appears to be the most appropriate native candidate for grassland compositional change (Smith Citation2003; Stewart Citation2004; Esler Citation2004). It is a naturally short (<25 cm), relatively unpalatable, turf-forming (shortly rhizomatous) grass that occurs in a wide range of light conditions, from dense shade to full sun, with most growth occurring in spring and early summer, and less in autumn and winter (Levy Citation1970; Robinson & Archer Citation1988; Magcale-Macandog & Whalley Citation1991). Dense M. stipoides swards effectively resist invasion by most other herbaceous and woody species (Esler Citation2004), so swards of this species would effectively slow natural succession to woody ecosystems. Increasing M. stipoides abundance on the cones may therefore be a useful management goal.
The urban reserves based on cones and other features of the Auckland volcanic field represent a substantial green space network that is a defining characteristic of Auckland city. They are key components of the natural, historic and cultural identity of Auckland, and their management is rightly the subject of critical debate and investment. The vegetation survey provided in this study provides a benchmark for future assessment of vegetation condition. The groundcover vegetation on the volcanic cone reserves should be monitored to determine trends in species composition (including indigenous biodiversity and weeds) and vegetation structure (e.g. for estimation of fire risk), and to assess the effectiveness of vegetation management. Currently, each of the Auckland volcanic cone reserves is managed largely independently. This may change if the current proposal for recognizing them as a World Heritage Site (Department of Conservation Citation2006) is successful. Managing the Auckland volcanic field reserves as a combined entity would allow particular reserves to be dedicated to achieving certain values rather than requiring each reserve to successfully meet the full range of management goals. In this way, and with creative management, the volcanic cone reserves of Auckland have further potential to provide increased natural, historic and cultural values.
Acknowledgements
We thank Craig Briggs (Landcare Research) for spatial analysis of topography on the cones, Robbie Price and Mark Smale (Landcare Research), Alec McGowan and Ian Power (AgResearch) for assistance with the field survey, Mat Campbell and Louise Furey (GFC Heritage) for archaeological advice, and Brian Daly (Landcare Research) for soil sample analysis.
Notes
1 The Rytidosperma sp. is probably R. racemosum, an exotic species identified on Maungawhau by Esler & Esler (Citation2011).
References
- Allen RB, Platt KH, Coker REJ 1995. Understorey species composition patterns in a Pinus radiata plantation on the central North Island volcanic plateau, New Zealand. New Zealand Journal of Forestry Science 25: 301–317.
- Atkinson IAE 1985. Derivation of vegetation mapping units for an ecological survey of Tongariro National Park, North Island, New Zealand. New Zealand Journal of Botany 23: 361–378.10.1080/0028825X.1985.10425343
- Auckland Regional Council 2010. Mt Smart Stadium (Mt Smart (Rarotonga) Domain) draft management plan. Second review. Auckland Regional Council. http://www.arc.govt.nz/albany/fms/main/Documents/Parks/Parks%20projects%20and%20plans/Park%20management%20plan/Mt%20Smart%20Stadium%20Draft%20Management%20Plan.pdf (accessed August 2012).
- Aust WM, Azola A, Johnson JE 2003. Management effects on erosion of Civil War military earthworks. Journal of Soil and Water Conservation 58: 13–20.
- Bell M, Fowler PJ, Hillson SW 1996. The Experimental Earthwork Project, 1960–1992. CBA Research Report. York, Council for British Archaeology. 267 p.
- Bircham JS, Gillingham AG 1986. A soil water balance for sloping land. New Zealand Journal of Agricultural Research 29: 315–323.10.1080/00288233.1986.10426988
- Blakemore LC, Searle PL, Daly BK 1987. Methods for chemical analysis of soils. New Zealand Soil Bureau Report 80. Lower Hutt, NZ Soil Bureau, DSIR. 103 p.
- Briggs C, Burns B 2007. Landform diversity of the Auckland Volcanic Cones. Landcare Research Contract Report LC0607/081.
- Cameron EK 1991. Mangere—a small forest remnant and Sicyos australis. Auckland Botanical Society Journal 46: 83–84.
- Cameron EK 1999. Mt Eden Rock Forests, Auckland City. Auckland Botanical Society Journal 54: 46–51.
- Clarke KR 1993. Non-parametric multivariate analyses of changes in community structure. Australian Journal of Ecology 18: 117–43.
- Craine JM, Lee WG, Walker S 2006. The context of plant invasions in New Zealand: evolutionary history and novel niches. In: Allen RB, Lee WG eds. Biological invasions in New Zealand. Berlin, Springer-Verlag. 457 p.
- Cranwell LM 1981. The botany of Auckland. Auckland, Auckland Institute and War Memorial Museum. 155 p.
- Department of Conservation 2006. Our World Heritage. A tentative list of New Zealand cultural and natural heritage sites. A report to the Department of Conservation by the Cultural and Natural Heritage Advisory Groups. Wellington, Department of Conservation. http://www.doc.govt.nz/upload/documents/getting-involved/consultations/consultations-results/our-world-heritage/our-world-heritage.pdf (accessed August 2012).
- Dodd M, Burns B, Smale M 2011. Auckland volcanic cones groundcover research: final report. Unpublished AgResearch contract report prepared for Auckland Council. 78 p.
- Dunwell AJ, Trout RC 1999. Burrowing animals and archaeology. Historic Scotland Technical Advice Note 16. Edinburgh, Historic Scotland. 14 p.
- Esler AE 1987. The naturalisation of plants in urban Auckland, New Zealand. 1. The introduction and spread of alien plants. New Zealand Journal of Botany 25: 511–522.
- Esler AE 1988. The naturalisation of plants in urban Auckland, New Zealand. 6. Alien plants as weeds. New Zealand Journal of Botany 26: 585–618.
- Esler AE 1991. Changes in the native plant cover of urban Auckland, New Zealand. New Zealand Journal of Botany 29: 177–196.
- Esler AE 2004. Wild plants in Auckland. Auckland, Auckland University Press. 218 p.
- Esler A, Esler W 2010. The grassland on Mount Eden—pasture dynamics. Auckland Botanical Society Journal 65: 137–140.
- Esler A, Esler W 2011. Grassland on Mt Eden—the flora. Auckland Botanical Society Journal 66: 122–127.
- Fairfield FG 1941. Maungakiekie One Tree Hill, Auckland. Description of some ethnological discoveries. The Journal of the Polynesian Society 50: 92–104.
- Fowlds GM 1928. Auckland's unique heritage: 63 wonderful volcanic cones and craters: an appeal to save them. Report on preservation of volcanic cones and craters. Auckland, Auckland Town Planning Association. 26 p.
- Fox A 1984. Maungakiekie: the Maori pa on One Tree Hill, Auckland. Auckland, One Tree Hill Borough Council. 16 p.
- Gillingham AG, Bell LD 1977. Effect of aspect and cloudiness on grass and soil temperatures at a hill site in Raglan County. New Zealand Journal of Agricultural Research 20: 37–44.10.1080/00288233.1977.10427299
- Gillingham AG, During C 1973. Pasture production and fertility transfer within a long-established hill pasture. New Zealand Journal of Experimental Agriculture 1: 227–232.
- Golson J 1957. Auckland's volcanic cones: a report on their condition and a plea for their preservation. Auckland, Historic Auckland Society. 32 p.
- Grant DA, Brock JL 1974. A survey of pasture composition in relation to soils and topography on a hill country farm in the southern Ruahine Range, New Zealand. New Zealand Journal of Experimental Agriculture 2: 243–250.
- Hayward BW 1983. Prehistoric pa sites of metropolitan Auckland. Tane 29: 3–14.
- Hayward BW, Murdoch GM, Maitland G 2011a. Volcanoes of Auckland: the essential guide. Auckland, Auckland University Press. 233 p.
- Hayward BW, Kenny JA, Grenfell HR 2011b. More volcanoes recognised in Auckland Volcanic Field. GeoScience Society of New Zealand Newsletter 5: 11–16.
- Homer L, Moore P, Kermode L 2000. Lava and strata: a guide to the volcanoes and rock formations of Auckland. Wellington, Landscape Publications. 96 p.
- Horrocks M, Deng Y, Nichol SL, Shane PA, Ogden J 2002. A palaeoenvironmental record of natural and human change from the Auckland Isthmus, New Zealand, during the late Holocene. Journal of the Royal Society of New Zealand 32: 337–353.
- Horrocks M, Augustinus P, Deng Y, Shane PA, Andersson S 2005a. Holocene vegetation, environment and tephra recorded from Lake Pupuke, Auckland. New Zealand Journal of Geology and Geophysics 48: 85–94.
- Horrocks M, Nichol SL, D'Costa DM, Shane P, Prior C 2005b. Palaeoenvironment and human impact in modifying vegetation at Mt St John, Auckland Isthmus, New Zealand. New Zealand Journal of Botany 43: 211–221.
- Jones KL 1998. The state of large earthwork sites in the United Kingdom. Antiquity 72: 293–307.
- Jones KL 2007. Caring for archaeological sites: practical guidelines for protecting and managing archaeological sites in New Zealand. Wellington, Department of Conservation. 126 p.
- Kirk T 1870. On the flora of the isthmus of Auckland and the Takapuna District. Transactions of the New Zealand Institute 3: 148–161.
- Lambert MG, Clark DA, Grant DA, Costall DA 1986. Influence of fertiliser and grazing management on North Island moist hill country 2. Pasture botanical composition. New Zealand Journal of Agricultural Research 29: 1–10.
- Ledgard SF, Sheath GW, Gillingham AG 1982. Influence of some soil and pasture components on the growth of hill country pastures. New Zealand Journal of Experimental Agriculture 10: 239–244.
- Levy EB 1970. Grasslands of New Zealand. 3rd edition Wellington, Government Printer. 374 p.
- Lindsay J, Leonard G, Smid E, Hayward BW 2011. Age of the Auckland volcanic field: a review of existing data. New Zealand Journal of Geology and Geophysics 54: 379–401.
- Magcale-Macandog DB, Whalley RDB 1991. Distribution of Microlaena stipoides and its association with introduced perennial grasses in a permanent pasture on the northern tablelands of New South Wales. Australian Journal of Botany 39: 295–303.
- Millener LH 1979. Forest, scrub and fresh-water communities. In: Brook PJ ed. Natural History of Auckland: an introduction. Auckland, Auckland Institute and Museum. 79 p.
- Paterson M 2009. Ngati Whatua o Orakei co-management of heritage places. Unpublished MSc thesis. University of Auckland, Auckland, New Zealand. 287 p.
- Payne A, Corney M, Cunliffe B 2006. The Wessex Hillforts Project: extensive survey of hillfort interiors in central southern England. London, English Heritage. 171 p.
- Percival NS 1979. Survey of kikuyu grass (Pennisetum clandestinum Hochst.) in New Zealand pastures. New Zealand Journal of Experimental Agriculture 6: 19–21.
- Perry LG, Blumenthal DM, Monaco TA, Paschke MW, Redente EF 2010. Immobilizing nitrogen to control plant invasion. Oecologia 163: 13–24.
- Radcliffe JE 1981. Aspect influences on pasture microclimate at Coopers Creek, North Canterbury. New Zealand Journal of Agricultural Research 24: 55–66.
- Rimmington JN 2004. Managing earthwork monuments: A guidance manual for the care of archaeological earthworks under grassland management. UK, London, English Heritage. 95 p.
- Robinson GG, Archer KA 1988. Agronomic potential of native grass species on the Northern Tablelands of New South Wales. I. Growth and herbage production. Australian Journal of Agricultural Research 39: 415–423.
- Searle EJ, Mayhill RD 1981. City of volcanoes: a geology of Auckland. 2nd edition Auckland, Longman Paul. 195 p.
- Smale MC, Gardner RO 1999. Survival of Mount Eden Bush, an urban forest remnant in Auckland, New Zealand. Pacific Conservation Biology 5: 83–93.
- Smale MC, McLeod M, Smale PN 1997. Vegetation and soil recovery on shallow landslide scars in tertiary hill country, East Cape region, New Zealand. New Zealand Journal of Ecology 21: 31–41.
- Smith P 2003. The suitability of a native grass species, Microlaena stipoides, for use as an amenity turfgrass in the Auckland region. Proceedings of the 2003 RNZIH conference: Greening the city: bringing biodiversity back into the urban environment. http://www.rnzih.org.nz/pages/2003_Conference_Proceedings_PDFs/33_Philip_Smith.pdf (accessed August 2012).
- Stewart A 2004. The potential of native NZ grasses for turf. New Zealand Turf Management Journal: 13–18.
- Trimble SW, Mendel AC 1995. The cow as a geomorphic agent—a critical review. Geomorphology 13: 233–253.
- Webby RW, Sheath GW, Boom CJ 1990. Performance of new pasture cultivars in a hill country finishing system. Proceedings of the New Zealand Grassland Association 51: 151–156.
- Wilson JH 1927: Some crevice plants from the lava field at Mt Wellington. Transactions of the New Zealand Institute 58: 255–263.