Abstract
Corynocarpus is a small, morphologically and phylogenetically isolated genus of trees from the southwest Pacific. We examined the phylogeny of Corynocarpus using the nuclear WAXY and ITS loci. Our molecular dating, when examined in combination with other published molecular dating studies, indicates that the origin of the genus and the arrival of Corynocarpus in New Zealand are likely to be much more recent than previously suggested. A second focus of our study was to investigate patterns of pre-European putative translocation of C. laevigatus (karaka, kōpi) by Māori throughout New Zealand using diversity at these two loci. Our New Zealand-wide sampling revealed the distinctiveness of specimens from the Three Kings Islands at both loci, precluding the Three Kings Islands as the source population for translocated C. laevigatus. However, the low level of sequence variation at these loci prevented further insight into the human-mediated dispersal of this species.
Introduction
The Corynocarpaceae is a small, morphologically and phylogenetically isolated family containing a single genus, Corynocarpus, of five tree species found in tropical to warm temperate areas in the southwest Pacific (Wagstaff & Dawson Citation2000; Filipowicz & Renner Citation2010). Corynocarpus similis is found in Vanuatu, the Solomon Islands, New Britain, New Ireland and the Bismarck Archipelago. Corynocarpus cribbianus is found in New Guinea (French Citation2006) and northeastern Queensland (van Steenis Citation1951). Corynocarpus rupestris occurs in isolated locations in Australia and has two subspecies. Corynocarpus rupestris subsp. rupestris occurs in northeastern New South Wales and is listed as vulnerable (Briggs & Leigh Citation1996). Corynocarpus rupestris subsp. arborescens is restricted to southeast Queensland (Guymer Citation1984). Corynocarpus dissimilis is endemic to New Caledonia (Hemsley Citation1903) and C. laevigatus is confined to mainland New Zealand (Aotearoa) and its offshore islands: Rekohu/Chatham Islands (hereafter Chatham Islands) and the Kermadec Islands (Molloy Citation1990).
Wagstaff & Dawson (Citation2000) examined the phylogenetic relationships of Corynocarpus by sequencing the nuclear internal transcribed spacer (ITS) region and chloroplast rbcL gene. They found C. similis to be sister to the other species within the genus. The closest relative of C. laevigatus was unclear because the position of C. dissimilis differed between their ITS and rbcL analyses, albeit with weak support. A reanalysis of the rbcL data showed that there was considerable conflict in the data at this locus (Atherton Citation2013), likely caused by multiple substitutions, making it unsuitable for inferring relationships in Corynocarpus.
Based on their analyses, Wagstaff & Dawson (Citation2000) suggest a palaeotropical centre of origin for the Corynocarpaceae, followed by two independent radiations into cooler climates. The first comprised C. cribbianus and C. rupestris extending through New Guinea to Australia, and the second comprised C. similis, C. dissimilis and C. laevigatus reaching New Zealand, through New Caledonia.
Wagstaff & Dawson (Citation2000) did not perform molecular dating but suggested that dispersal of Corynocarpus from New Caledonia to New Zealand may have occurred via land connections that were present in the mid-Tertiary (Herzer et al. Citation1997). Campbell (Citation2002) subsequently reported fossilised Corynocarpus kernels dating to the early Miocene (23–16 mya) at Landslip Hill in Southland, New Zealand, providing evidence for a long history of the genus in this country.
The cultivation of Corynocarpus
There are records of three species of Corynocarpus historically being used for food by indigenous peoples (Yen Citation1974; Wheatley Citation1992; Leach & Stowe Citation2005; French Citation2006). In New Zealand, the kernels of C. laevigatus (karaka, kōpi) were an important food source for Māori before European settlement (Leach & Stowe Citation2005). Prior to human arrival in New Zealand, C. laevigatus is thought to have been restricted to the northern North Island (Molloy Citation1990). Its occurrence in the southern North Island, the South Island, Chatham and Kermadec Islands () is associated with Māori and Moriori archaeological sites (Stowe Citation2003) and is considered to have resulted from translocations as part of its cultivation (Molloy Citation1990; Leach & Stowe Citation2005; Costall et al. Citation2006). The pollen record of C. laevigatus (Mildenhall Citation1994; Byrami et al. Citation2002; Wilmshurst et al. Citation2004) and Māori oral histories (reviewed in Leach & Stowe Citation2005) provide further support for this hypothesis. There is also some evidence that Māori selected for larger fruit size in cultivated trees (van Essen & Rapson Citation2005).
Figure 1 Distribution of Corynocarpus laevigatus. A, Across New Zealand including the Three Kings Islands off the northern tip of New Zealand's North Island, the Kermadec Islands to the north and the Chatham Islands to the west; B, northern South Island; C, North Island of New Zealand showing locations of inland C. laevigatus populations. The grey circles are records in the AK, CHR, NZFRI and WELT herbaria. Herbaria abbreviations follow Thiers (Citation2015). The black circles represent specimens sampled for this study.
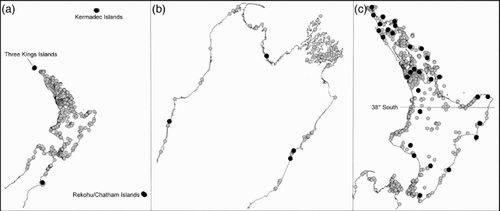
Genetic analyses potentially provide a means by which to trace the origins and human-mediated dispersal of cultivated species (reviewed in Zeder et al. Citation2006), such as C. laevigatus. Chloroplast sequence markers have been commonly used for such studies, but limited infraspecific variation was detected across the entire chloroplast genome of C. laevigatus (Atherton Citation2013). Nuclear sequence markers have also been successfully used to trace plant movements (e.g. Aguilar-Meléndez et al. Citation2009; Besnard & El Bakkali Citation2014). Wagstaff & Dawson (Citation2000) sequenced the ITS locus from five specimens of C. laevigatus but found no variation. However, four of these specimens were collected from within the putative translocated distribution, which may explain the resulting lack of genetic diversity. Expanding this sampling, particularly within the pre-human distribution of this species, may determine the site(s) of initial cultivation of C. laevigatus as well as the routes of its dispersal, including to the distant Kermadec and Chatham Island groups. We also sequence an additional nuclear locus, WAXY.
The aims of this study were to: 1. determine the relationships of the taxa within Corynocarpaceae, in particular the closest relative of C. laevigatus, by sequencing the nuclear gene WAXY; 2. test the hypothesis of a mid-Tertiary origin of Corynocarpus in New Zealand using ITS molecular dating; and 3. investigate the human-mediated dispersal history of C. laevigatus by sequencing the ITS and WAXY loci from additional specimens, with a focus on those collected from within the likely pre-human distribution of this species.
Methods
Sampling
Single DNA samples from each of the five species and subspecies of Corynocarpus endemic to regions outside New Zealand (C. cribbianus, C. dissimilis, C. similis, C. rupestris subsp. rupestris and C. rupestris subsp. arborescens) were available from the Wagstaff & Dawson (Citation2000) study. For this study we sampled an additional 44 accessions of C. laevigatus, covering its known range in New Zealand (Table S1). Representative herbarium vouchers have been deposited into the Auckland Museum (AK) and Museum of New Zealand Te Papa Tongarewa (WELT) herbaria.
DNA extraction, polymerase chain reaction amplification and sequencing
Fresh or silica-dried leaf tissue from C. laevigatus samples was snap-frozen in liquid nitrogen and powdered using a disposable grinder or milled from silica-dried leaves using a MagnaLyser with 2 mm zirconia beads (Biospec, Bartlesville, OK). Total genomic DNA was extracted from leaf tissue using a modified CTAB protocol (step 1, followed by steps 3–7 of table 1 in Shepherd & McLay Citation2011).
The nuclear ITS was PCR amplified and sequenced for the 44 new C. laevigatus samples using the primers ITS5 (White et al. Citation1990) and ITS28cc (Wagstaff & Garnock-Jones Citation1998). A fragment including exons 10–13 at the 3′ end of the nuclear WAXY gene was amplified for the overseas Corynocarpus samples (n = 5) and a subset of the C. laevigatus samples (n = 36) with the primers WAXY 10F and WAXY 13R (Tank & Olmstead Citation2009).
Polymerase chain reactions (PCRs) were performed using the thermocycling protocol outlined in Shaw et al. (Citation2005) on a T3 Thermocycler (Biometra, Göttingen, Germany) in 25 μl reactions containing c. 50 ng DNA, 2.5 mM MgCl2, 0.5 U Red Hot Taq DNA polymerase (Thermo Fisher Scientific, Waltham, MA), 2.5 μl 10 × buffer, 200 μM of each dNTP and 0.5 mM of each primer. Amplification products were purified by digestion with 0.2 U shrimp alkaline phosphatase (SAP; USB Corp., Cleveland, OH) and 1 U exonuclease I (ExoI, USB Corp.) at 37 °C for 30 min, followed by inactivation of the enzymes at 80 °C for 15 min.
Sequencing was performed in both directions with the ABI PRISM BigDye® Terminator v3.1 Ready Reaction Cycle Sequencing kit (Applied Biosystems, Foster City, CA) following the manufacturer's protocol. Unincorporated fluorescent dNTPs were removed using CleanSEQ (Agencourt, Beverly, MA) again following the manufacturer's protocol. Capillary separation was subsequently undertaken at the Massey Genome Service, Palmerston North. Sequences were edited with Sequencher 4.9 (GeneCodes Corp., Ann Arbor, MI).
Data analysis
The edited sequences were aligned using Muscle (www.ebi.ac.uk/Tools/msa/muscle). For both loci, the few indels were removed, resulting in alignments of length 669 bp (ITS) and 455 bp (WAXY).
Because previous phylogenies based on rbcL and ITS sequence data gave conflicting, albeit weakly supported relationships (Wagstaff & Dawson Citation2000; Atherton Citation2013), we used network methods to analyse our data. For each locus a NeighborNet network was constructed based on uncorrected p-distances using SplitsTree4 (Huson & Bryant Citation2006). The few ambiguous sites present were treated as missing data. Support was assessed using 1000 bootstrap pseudoreplicates.
In order to date the divergence times between ITS variants within C. laevigatus and between C. laevigatus and its nearest relative we performed molecular clock analyses in BEAST v2.1.3 (Bouckaert et al. Citation2014). To test for clock-like evolution we examined the log of the standard deviation in rates (ucld.stdev statistic) in TRACER v1.6 (Rambaut et al. Citation2013). The frequency histogram abutted zero, indicating that there is little rate variation across lineages and a strict molecular clock cannot be rejected (Drummond et al. Citation2007). Strict molecular clock analyses were performed on the ITS alignment with the HKY + G model of sequence evolution, selected using the Akaike information criterion in jModelTest v0.1.1 (Posada Citation2008), empirical base frequencies and a birth–death model tree prior. The analyses were run for 50 million generations, with the first 10% of samples discarded as burn-in. Convergence was confirmed by checking plots of –lnL across generations and ESS for each parameter (>200 for all cases) with TRACER v1.6 (Rambaut et al. Citation2013) and performing three independent analyses with different starting seeds.
The fossil record of the largely herbaceaous and insect-pollinated Cucurbitales is sparse (Crepet et al. Citation2004; Zhang et al. Citation2006). Additionally, the high level of ITS sequence variation between the Corynocarpaceae and other members of the Cucurbitales makes sequence alignment difficult across these distantly related taxa (Atherton Citation2013). Therefore, for molecular dating we used an average substitution rate of 2.15 × 10−9 substitutions per site per year ± 1.85 × 10−9, based on independently calibrated ITS substitution rates from 18 woody plant groups (Kay et al. Citation2006). We also performed analyses using the slowest substitution rate reported by Kay et al. (Citation2006) for a woody plant, 0.38 × 10−9 substitutions per site per year for Hamamelis (Wen & Shi Citation1999).
Results
Nuclear DNA sequences and phylogenetic relationships
The ITS sequences from 40 of the C. laevigatus specimens were identical to the five sequences obtained by Wagstaff & Dawson (Citation2000) for this species. The ITS sequences from the remaining four specimens, all from the Three Kings Islands (), differed from the other C. laevigatus sequences by four nucleotide substitutions. This is twice the number of nucleotide substitutions distinguishing the two subspecies of C. rupestris. Similarly, the only variation detected within C. laevigatus at the WAXY locus was a single substitution distinguishing the two Three Kings Islands’ C. laevigatus sequences from the remaining 34 specimens sampled from other locations in New Zealand.
Across the alignment of Corynocarpus ITS sequences with indels removed there were 38 variable sites, 14 of which were parsimony-informative. The ITS NeighborNet network (A) was largely tree-like, with a small amount of reticulation. The network suggests that the ancestral sequence of the two C. laevigatus sequences was either unsampled or no longer extant. Assuming that the root for the genus joins the branch leading to C. similis, following Wagstaff & Dawson (Citation2000), there was some support (64% bootstrap support [BS]) for C. dissimilis being sister to C. laevigatus. A conflicting split for C. dissimilis being sister to C. cribbianus received even less support (48% BS).
Figure 2 NeighborNet networks. A, ITS DNA sequences; B, WAXY DNA sequences. According to the analyses of Wagstaff & Dawson (Citation2000), Corynocarpus similis is the basally diverging taxon within the genus, so the root of the network falls on the branch leading to this species.
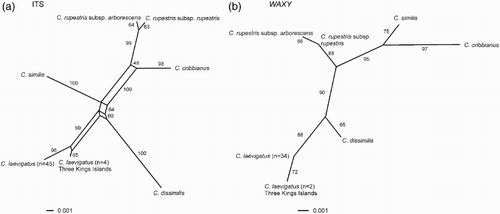
The WAXY alignment of all Corynocarpus sequences contained 18 variable sites, of which seven were parsimony-informative. The WAXY NeighborNet network (B) contained no reticulation. It indicates that the WAXY sequence variant found in C. laevigatus sampled from mainland New Zealand, the Chatham Islands and Kermadec Islands is ancestral to that detected from the Three Kings Islands. For WAXY, C. laevigatus is strongly supported as sister to C. dissimilis (90% BS).
The phylogenies inferred from the two loci differed in the relationships between C. cribbianus, C. rupestris and C. similis (A–B). In the ITS NeighborNet, C. rupestris is strongly supported as sister to C. cribbianus (100% BS), but with WAXY C. rupestris is sister to C. laevigatus and C. dissimilis (95% BS).
Molecular dating
The molecular clock analyses of ITS based on a substitution rate averaged from 18 woody plant species (Kay et al. Citation2006) suggested a late Pliocene/early Miocene date for the most recent common ancestor of the genus (6.6 mya, 95% highest posterior density [HPD] 2.4–11.8 mya). Corynocarpus laevigatus and C. dissimilis diverged approximately 4.9 mya (95% HPD 1.6–8.9 mya). The two sequence variants in C. laevigatus (Three Kings Islands versus remaining New Zealand samples) separated around 1.1 mya (95% HPD 0.2–3.2 mya).
Using the slowest recorded woody plant ITS substitution rate of 0.38 × 10−9 substitutions per site per year indicates late Eocene/Oligocene divergence dates for the most recent common ancestor of the genus (37.2 mya, 95% HPD 23.9–50.6 mya), and the divergence of C. laevigatus from C. dissimilis (28.1 mya, 95% HPD 16.7–40.6 mya). With this rate, the Three Kings Islands ITS variant is estimated to have diverged from the ITS variant found in the remaining New Zealand C. laevigatus 6.3 mya (2.1–16.2 mya).
Discussion
Genetic variation within C. laevigatus
Our New Zealand-wide sequencing of C. laevigatus revealed infraspecific DNA sequence variation for the first time, and at both the ITS and WAXY loci. Both markers showed the genetic distinctiveness of specimens sampled from the Three Kings Islands. For ITS the number of nucleotide substitutions detected between samples from the Three Kings Islands versus the remaining C. laevigatus sequences was twice the number of substitutions at this locus between the two subspecies of C. rupestris, which differ in a number of morphological characters. However, we do not think that the Three Kings Islands’ C. laevigatus should be recognised at the subspecific level because, as far as we are aware, there are no morphological characters distinguishing the Three Kings Islands population from remaining C. laevigatus.
The archipelago of the Three Kings Islands is 56 km from the northern tip of mainland New Zealand and comprises 13 islands ranging from small rock stacks to four main islands, of which the largest is Manawatāwhi (also known as Great Island) at just over 4 km2. Manawatāwhi was inhabited in 1642 when Tasman visited New Zealand (Cheeseman Citation1887), but was deserted around 1840. It is thought that the original vegetation of the islands was mixed broadleaf forest (Baylis Citation1951; de Lange et al. Citation1996).
The Three Kings Islands is home to a number of endemic taxa, including 13 endemic plant species and one endemic plant genus (Elingamita) (Oliver Citation1948; Cranwell Citation1962; Wright Citation1983), indicating a long history of isolation. Buckley & Leschen (Citation2013) used molecular dating to estimate divergence times between insect lineages on the Three Kings Islands and their sister groups from the rest of New Zealand. These dates ranged from 2.24–24 mya and led the authors to suggest that emergent land had been present in the area since the Miocene, but there was no land connection between the Three Kings Islands and mainland New Zealand during the Pleistocene when sea levels were lower due to glaciation. Our estimated divergence times for the Three Kings Islands ITS variant (0.3–2.9 mya and 1.8–16.7 mya for the average and slow ITS substitution rates, respectively) are consistent with, but at the younger end of, Buckley and Leschen's dates.
The genetic distinctiveness of sequences from the Three Kings Islands at both the ITS and WAXY loci preclude this site as the source for the putative translocated populations included in our sampling. The higher genetic diversity on the New Zealand mainland (including the Three Kings Islands) compared with the Kermadec Islands supports Stevenson's (Citation1978) suggestion that C. laevigatus was moved from the New Zealand mainland to the Kermadec Islands. However, the lack of further variation at these loci prevents any additional inferences about the origins of the translocated populations within the New Zealand mainland.
Previous studies have gained insight into human-mediated dispersal of plant crops with nuclear sequence markers, in some cases with only a single locus (e.g. Léotard et al. Citation2009). Whether a nuclear locus is informative for examining the dispersal of a given species depends on the level of genetic variation and genetic structuring it exhibits, both of which are dependent on the demographic history of the species. New genetic markers for C. laevigatus, which will hopefully display greater genetic diversity, are under development using next-generation sequencing methods.
Corynocarpus phylogeny
For WAXY there was strong support for C. dissimilis being sister to C. laevigatus. This relationship also received some support from ITS, although a conflicting split with low support was also present in the ITS data. Using the average ITS substitution rate for woody plants, the divergence date calculated for the split of C. dissimilis and C. laevigatus (1.6–8.9 mya) is much more recent than the mid-Tertiary date hypothesised by Wagstaff & Dawson (Citation2000). There are limitations in using an average ITS substitution rate for dating divergences in Corynocarpus. First, this average rate derives from plant lineages only distantly related to Corynocarpus. Second, there are errors associated with the individual rates used to calculate this average, introduced from a number of sources including inaccurate fossil dating and phylogenetic uncertainty (Kay et al. Citation2006) that are not taken into account. However, for Wagstaff & Dawson's (Citation2000) mid-Tertiary date to be correct, the rate of ITS evolution in Corynocarpus would have to be as slow as the slowest known rate for plants (i.e. Hamamelis; Wen & Shi Citation1999).
The divergence dates calculated using both the average and slowest rates are consistent with the pollen record, where the age of first appearance of Corynocarpus pollen in New Zealand is 2.5 mya (Mildenhall Citation1980). However, the dates calculated using the slowest ITS substitution rate would suggest a long presence of Corynocarpus in New Zealand without leaving evidence in the pollen record. This would not be unexpected given that Corynocarpus is severely under-represented in the pollen record (Dodson Citation1976; Holt Citation2009).
Our dates calculated with the average ITS substitution rate for both the split of C. laevigatus and the crown age (most recent common ancestor) of Corynocarpus postdate the early Miocene fossilised Corynocarpus kernels in New Zealand reported by Campbell (Citation2002). However, in the DNA sequence-based phylogeny of the Cucurbitales there is a long branch to the extant species of Corynocarpaceae (Wagstaff & Dawson Citation2000; Zhang et al. Citation2006). The Miocene fossil may represent an ancestor or extinct relative of the extant Corynocarpus species. If the dates calculated using the average ITS substitution rate are correct then an unbroken New Zealand-based lineage between the Miocene fossil and C. laevigatus is not supported, unless there have been multiple long-distance dispersal emigrations from New Zealand to found the species that now occur elsewhere.
Alternatively, if the rate of ITS evolution in Corynocarpus is as slow as the slowest known substitution rate in plants then a long history of presence of Corynocarpus in New Zealand is suggested and the Miocene fossil may represent a direct ancestor of C. laevigatus. However, the inferred ages of Corynocarpus divergence calculated using the slowest rate are inconsistent with Cucurbitales’ divergence estimates, calculated with mitochondrial matR and nuclear 18S sequences and calibrated with a Fagales fossil (Bellot & Renner Citation2014). Bellot & Renner (Citation2014) estimated that Corynocarpus diverged from Anisophyllea between 30–50 mya (fig. 3A–F; Bellot & Renner Citation2014). Their result is inconsistent with our molecular dating using the ITS slow rate because it is similar to our estimate for the most recent common ancestor of Corynocarpus. The long-branch to Corynocarpus that has been found in past phylogenies (Wagstaff & Dawson Citation2000) indicates considerable time elapsed between the divergence of Corynocarpaceae and the diversification of the genus.
Although our phylogenies of the two loci were largely in agreement over the relationship between C. laevigatus and C. dissimilis, the relationships of the remaining Corynocarpus species were less clear, with alternative phylogenies receiving high support. Discordance between tree topologies may result from incomplete lineage sorting or past hybridisation. All species of Corynocarpus are diploid except for both subspecies of C. rupestris, which are tetraploid (Dawson Citation1997). It is unknown if their origin included hybridisation (allopolyploidy), which may explain the contrasting topologies. Sequencing of additional nuclear loci is expected to bring further resolution to the evolutionary history of the genus.
Supplementary data
Table S1. Details of Corynocarpus specimens used in this study.
Table S1. Details of Corynocarpus specimens used in this study.
Download MS Word (111.9 KB)Acknowledgements
We would like to acknowledge all iwi and hapū who granted us permission to collect from within their rohe, in particular those who contacted and/or met with us in person: the Hokotehi Moriori Trust Board, Ngāti Kuri, Te Aupouri, Tiakina te Taiao, Rangitāne, Te Atiawa, Ngāi Tahu, Ngātiwai, Patuharakeke Trust, Ngā Puhi, Te Rarawa and Ngā Rauru. Samples were collected under Department of Conservation permits WA-23814-FLO, BOP-23814-FLO, TT-23661-FLO, NO-233360-FLO and Otari Wilton's Bush permit 145. We thank Rewi Elliot and Eleanor Burton (Otari Wilton's Bush, Wellington), Murray Thacker (Okains Bay), Kevin Matthews (Kaitaia), Barbara and Dan Ramsden (Moanaroa), Janene Collings, Jeremy Rolfe and David Havell (Department of Conservation), Trevor Thompson, Bill Wallace, Greg Blunden (QEII Trust), Tricia Aspin and Leon Perrie for assistance with sample collection. We also thank Leon Perrie, Jill Rapson and an anonymous reviewer for comments on the manuscript.
Disclosure statement
No potential conflict of interest was reported by the authors.
Additional information
Funding
References
- Aguilar-Meléndez A, Morrell PL, Roose ML, Kim SC 2009. Genetic diversity and structure in semiwild and domesticated chiles (Capsicum annuum; Solanaceae) from Mexico. American Journal of Botany 96: 1190–1202. doi: 10.3732/ajb.0800155
- Atherton RA 2013. Ngā Uri O Karaka: a genetic study of the karaka/kōpi tree in Aotearoa/New Zealand. Unpublished PhD thesis, Massey University, Palmerston North, New Zealand. 200p.
- Baylis GTS 1951. Incipient forest regeneration on Great Island, Three Kings group. Records of the Auckland Institute and Museum 5: 1–12.
- Bellot S, Renner SS 2014. Exploring new dating approaches for parasites: the worldwide Apodanthaceae (Cucurbitales) as an example. Molecular Phylogenetics and Evolution 80: 1–10.
- Besnard G, El Bakkali A 2014. Sequence analysis of single-copy genes in two wild olive subspecies: nucleotide diversity and potential use for testing admixture. Genome 57: 145–153. doi: 10.1139/gen-2014-0001
- Bouckaert R, Heled J, Kühnert D, Vaughan T, Wu CH, Xie D, et al. 2014. BEAST 2: a software platform for Bayesian evolutionary analysis. PloS Computational Biology 10(4): e1003537. doi: 10.1371/journal.pcbi.1003537
- Briggs JD, Leigh JH 1996. Rare or threatened Australian plants. 4th edition. Melbourne, CSIRO. 476 p.
- Buckley TR, Leschen RAB 2013. Comparative phylogenetic analysis reveals long-term isolation of lineages on the Three Kings Islands, New Zealand. Biological Journal of the Linnean Society 108: 361–377. doi: 10.1111/j.1095-8312.2012.02009.x
- Byrami M, Ogden J, Horrocks M, Deng Y, Shane P, Palmer J 2002. A palynological study of Polynesian and European effects on vegetation in Coromandel, New Zealand, showing the variability between four records from a single swamp. Journal of the Royal Society of New Zealand 32: 507–531. doi: 10.1080/03014223.2002.9517706
- Campbell JD 2002. Angiosperm fruit and leaf fossils from Miocene silcrete, Landslip Hill, northern Southland, New Zealand. Journal of the Royal Society of New Zealand 32: 149–154. doi: 10.1080/03014223.2002.9517687
- Cheeseman T 1887. Art. XXII—notes on the Three Kings Islands. Transactions and Proceedings of the Royal Society of New Zealand 20: 142–149.
- Costall JA, Carter RJ, Shimada Y, Anthony D, Rapson GL 2006. The endemic tree Corynocarpus laevigatus (karaka) as a weedy invader in forest remnants of southern North Island, New Zealand. New Zealand Journal of Botany 44: 5–22. doi: 10.1080/0028825X.2006.9513002
- Cranwell LM 1962. Endemism and isolation in the Three Kings Islands, New Zealand—with notes on pollen and spore types of the endemics. Records of the Auckland Institute and Museum 5: 215–232.
- Crepet WL, Nixon KC, Gandolfo MA 2004. Fossil evidence and phylogeny: the age of major angiosperm clades based on mesofossil and macrofossil evidence from Cretaceous deposits. American Journal of Botany 91: 1666–1682. doi: 10.3732/ajb.91.10.1666
- Dawson MI 1997. Chromosome numbers in Corynocarpus (Corynocarpaceae) New Zealand. Journal of Botany 35: 255–258.
- Dodson JR 1976. Modern pollen spectra from Chatham Island, New Zealand. New Zealand Journal of Botany 14: 341–347. doi: 10.1080/0028825X.1976.10428907
- Drummond AJ, Ho SYW, Rawlence N, Rambaut A 2007. A rough guide to BEAST 1.4. http://www.molecularevolution.org/molevolfiles/beast/BEAST14_MANUAL-7-6-07.pdf (accessed 27 July 2015).
- van Essen RA, Rapson GL 2005. Fruit size of karaka (Corynocarpus laevigatus) in relation to potential selection by Maori. New Zealand Botanical Society Newsletter 81: 13–16.
- Filipowicz N, Renner SS 2010. The worldwide holoparasitic Apodanthaceae confidently placed in the Cucurbitales by nuclear and mitochondrial gene trees. BMC Evolutionary Biology 10: 219. doi: 10.1186/1471-2148-10-219
- French BR 2006. Food crops of Papua New Guinea. www.foodplantsinternational.com (accessed 30 March 2015).
- Guymer GP 1984. Corynocarpus. Flora of Australia Online. Australian Biological Resources Study, Canberra. http://www.environment.gov.au/biodiversity/abrs/online-resources/flora/main/index.html (accessed 30 March 2015).
- Hemsley WB 1903. On the genus Corynocarpus, Forst., with descriptions of two new species. Annals of Botany 17: 743–760.
- Herzer RH, Chaproniere GCH, Edwards AR, Hollis CJ, Pelletier B, Raine JI, et al. 1997. Seismic stratigraphy and structural history of the Reinga Basin and its margins, southern Norfolk Ridge system. New Zealand Journal of Geology and Geophysics 40: 425–451. doi: 10.1080/00288306.1997.9514774
- Holt K 2009. The Quarternary history of Chatham Island, New Zealand. Unpublished PhD thesis. Massey University, Palmerston North. 243p.
- Huson DH, Bryant D 2006. Application of phylogenetic networks in evolutionary studies. Molecular Biology and Evolution 23: 254–267. doi: 10.1093/molbev/msj030
- Kay K, Whittall J, Hodges S 2006. A survey of nuclear ribosomal internal transcribed spacer substitution rates across angiosperms: an approximate molecular clock with life history effects. BMC Evolutionary Biology 6: 1471–2148. doi: 10.1186/1471-2148-6-36
- de Lange PJ, Lowe DJ, Newnham RM 1996. What was the original forest cover of Great Island (Three Kings)? Conservation Science Newsletter (Science and Research Division. Department of Conservation) 21/22: 7–11.
- Leach HM, Stowe CJ 2005. Oceanic arboriculture at the margins: the case of the karaka (Corynocarpus laevigatus) in Aotearoa. Journal of the Polynesian Society 114: 7–27.
- Léotard G, Duputié A, Kjellberg F, Douzery EJP, Debain C, de Granville JJ, et al. 2009. Phylogeography and the origin of cassava: new insights from the northern rim of the Amazonian basin. Molecular Phylogenetics and Evolution 53: 329–334. doi: 10.1016/j.ympev.2009.05.003
- Mildenhall DC 1980. New Zealand late Cretaceous and cenozoic plant biogeography: a contribution. Palaeogeography, Palaeoclimatology, Palaeoecology 31: 197–233.
- Mildenhall DC 1994. Palynological reconnaissance of Early Cretaceous to Holocene sediments, Chatham Islands, New Zealand. Lower Hutt, New Zealand, Institute of Geological & Nuclear Sciences Ltd.
- Molloy BPJ 1990. The origin, relationships, and use of karaka or kopi (Corynocarpus laevigatus). In: Kapoor WHAP ed. Nga Mahi Maori o te Wao Nui a Tane: contributions to an International Workshop on Ethnobotany, Te Rehua Marae. Christchurch, Botany Division, Department of Scientific and Industrial Research. Pp. 48–53.
- Oliver WRB 1948. The flora of the Three Kings Islands. Records of the Auckland Institute and Museum 3: 211–238.
- Posada D 2008. jModelTest: phylogenetic model averaging. Molecular Biology and Evolution 25: 1253–1256. doi: 10.1093/molbev/msn083
- Rambaut A, Suchard MA, Xie W, Drummond AJ 2013. Tracer v1.6. http://tree.bio.ed.ac.uk/software/tracer
- Shaw J, Lickey EB, Beck JT, Farmer SB, Liu W 2005. The tortoise and the hare II: relative utility of 21 noncoding chloroplast DNA sequences for phylogenetic analysis. American Journal of Botany 92: 142–166. doi: 10.3732/ajb.92.1.142
- Shepherd LD, McLay TGB 2011. Two micro-scale protocols for the isolation of DNA from polysaccharide-rich plant tissue. Journal of Plant Research 124: 311–314. doi: 10.1007/s10265-010-0379-5
- van Steenis CGGJ 1951. Corynocarpaceae. In: Flora Malesiana Series 1: Spermatophyta. Jakarta, Noordhoff–Kolff. Pp. 262–264.
- Stevenson G 1978. Botanical evidence linking the New Zealand Maoris with New Caledonia and the New Hebrides. Nature 276: 704–705. doi: 10.1038/276704a0
- Stowe CJ 2003. The ecology and ethnobotany of karaka (Corynocarpus laevigatus). Unpublished MSc thesis, University of Otago, Dunedin, New Zealand. 238 p.
- Tank DC, Olmstead RG 2009. The evolutionary origin of a second radiation of annual Castilleja (Orobanchaceae) species in South America: the role of long distance dispersal and allopolyploidy. American Journal of Botany 96: 1907–1921. doi: 10.3732/ajb.0800416
- Thiers B 2015 (continuously updated). ‘Index herbariorum: a global directory of public herbaria and associated staff.’ New York Botanical Garden's Virtual Herbarium. http://sweetgum.nybg.org/ih/ (accessed 3 June 2015).
- Wagstaff S, Dawson M 2000. Classification, origin, and patterns of diversification of Corynocarpus (Corynocarpaceae) inferred from DNA sequences. Systematic Botany 25: 134–149. doi: 10.2307/2666679
- Wagstaff SJ, Garnock-Jones PJ 1998. Evolution and biogeography of the Hebe complex (Scrophulariaceae) inferred from ITS sequences. New Zealand Journal of Botany 36: 425–437. doi: 10.1080/0028825X.1998.9512581
- Wen J, Shi S 1999. A phylogenetic and biogeographic study of Hamamelis (Hamamelidaceae), an eastern Asian and North American disjunct genus. Biochemical Systematics and Ecology 27: 55–66. doi: 10.1016/S0305-1978(98)00067-2
- Wheatley JI 1992. A guide to the common trees of Vanuatu with lists of their traditional uses and ni-Vanuatu names. Port Vila, Department of Forestry.
- White T, Bruns T, Lee S, Taylor J 1990. Amplification and direct sequencing of fungal ribosomal RNA genes for phylogenetics. In Innis M, Gelfand D, Shinsky J, White T eds. PCR protocols: a guide to methods and applications. New York, Academic Press. Pp. 315–322.
- Wilmshurst JM, Higham TFG, Allen H, Johns D 2004. Early Maori settlement impacts in northern coastal Taranaki, New Zealand. New Zealand Journal of Ecology 28: 167–179.
- Wright AE 1983. Conservation status of the Three Kings Islands endemic flora in 1982. Records of the Auckland Institute and Museum 20: 175–184.
- Yen DE 1974. Arboriculture in the subsistence of Santa Cruz, Solomon Islands. Economic Botany 28: 247–284. doi: 10.1007/BF02861424
- Zeder MA, Emshwiller E, Smith BD & Bradley DG 2006. Documenting domestication: the intersection of genetics and archaeology. Trends in Genetics 22: 139–155. doi: 10.1016/j.tig.2006.01.007
- Zhang L-B, Simmons MP, Kocyan A, Renner SS 2006. Phylogeny of the Cucurbitales based on DNA sequences from three genomes: implications for morphological and sexual system evolution. Molecular Phylogenetics and Evolution 39: 305–322. doi: 10.1016/j.ympev.2005.10.002