ABSTRACT
The Glen Murray fossil penguin was one of the first ancient penguins to be reported from the North Island of New Zealand when it was described in 1973. However, its significance for penguin diversity has remained largely unresolved since initial description. Here we show that the Glen Murray penguin exhibits two of three traits that are autapomorphic for Kairuku: the femur is very stout with a midshaft width <15% of the total femoral length and the tibiotarsus has a distinctly convex medial border in distal view. The third trait (absence of the crista medialis hypotarsi) cannot be assessed as the proximal end of the tarsometatarsus is missing. The Glen Murray penguin is most likely a distinct species that should be assigned to the genus Kairuku, although we refer it to Kairuku sp. pending the discovery of more material. Reappraisal of local stratigraphy indicates that the Glen Murray fossil penguin is from the Dunphail Siltstone member of the Glen Massey Formation and can be assigned to the lower Whaingaroan stage (34.6–29.8 Ma).
Introduction
Evolution of the penguin body plan can be tracked through a comparatively rich fossil record distributed across southern landmasses (Ksepka & Ando Citation2011). New Zealand in particular is a major source of penguin fossils and has a deep fossil record with respect to marine birds (Slack et al. Citation2006; Mayr & Scofield Citation2014, Citation2016). The 60 million year history of Zealandian penguins reveals a rapid early evolution towards the characteristically fusiform body shape, a huge range in body size and a remarkable record of high diversity levels across the Cenozoic (Fordyce & Jones Citation1990; Ksepka et al. Citation2006, 2012; Slack et al. Citation2006; Fordyce & Thomas Citation2011). Zealandia is a subcontinent that splintered from the ancient supercontinent Gondwana; modern-day New Zealand is effectively subaerial Zealandia (Mortimer Citation2004). The diversity of Zealandian penguins that lived during the Oligocene (34–23 Ma) ranged from little blue (Eudyptula minor Forster, Citation1781)-sized species (i.e. approximately 30 cm tall) through to giants that exceeded the size of the largest living species, the emperor penguin (extinct taxa 1.28 m tall) (Fordyce & Jones Citation1990; Ksepka et al. Citation2012). The driver behind the growth and eventual winnowing of penguin diversity is unknown (Ando & Fordyce Citation2014), with current evidence suggesting that penguin diversity peaked during the Eocene and Oligocene (56–23 Ma). The Oligocene in particular was a time of major geographic reconfiguration for Zealandia and elsewhere (e.g. Strogen et al. Citation2014); however, finding a causative link between environmental change and penguin diversity would require finer resolution of the penguin fossil record. Most of the New Zealand Oligocene penguin fossils come from Canterbury Basin sediments (southeastern Zealandia) and comparatively little is known about penguin diversity from the North Island (more northern and eastern palaeogeographic regions of Oligocene Zealandia). The discovery of the Glen Murray penguin was thus significant for confirming that ancient penguin remains could be recovered from Te Kuiti Group sediments (Simpson Citation1971a; Grant-Mackie & Simpson Citation1973).
The Glen Murray penguin was discovered in 1971 in the Waikato District of New Zealand. The partially preserved hindlimb bones were larger than equivalent bones in modern emperor penguins. They were immediately identified to the level of Sphenisciformes, but the eroded remains were missing key characters known to diagnose genera or species based on the state of taxonomic knowledge at the time (Grant-Mackie & Simpson Citation1973). Five species of giant fossil penguin were known in the early 1970s: Anthropornis nordenskjoeldi Wiman, Citation1905; Anthropornis grandis Wiman, Citation1905; Pachydyptes ponderosus Oliver, Citation1930; Palaeeudyptes antarcticus Huxley, Citation1859; and Palaeeudyptes marplesi Brodkorb, Citation1963. Each of these species except Pachydyptes ponderosus had skeletal elements that overlapped with the Glen Murray penguin. Grant-Mackie & Simpson (Citation1973) observed that:
as far as preserved, the femur and the tarsometatarsus of the Glen Murray Penguin show considerable resemblance to Palaeeudyptes marplesi both in size and in form. They show less resemblance to any other named taxon … [w]ithout assurance that diagnostic characters are present, it would be irresponsible to refer this specimen to Palaeeudyptes marplesi or Palaeeudyptes. Without assurance that some such characters are absent and others probably diagnostic are present, it would be irresponsible to take this specimen as representative of some other taxon …
Now though, we have the benefit of more than 40 additional years of fossil penguin discoveries and taxonomic revisions. Over this time, the Glen Murray penguin has received little attention in comparative studies, likely because it was not diagnosed beyond the level of Sphenisciformes.
The number of described fossil penguin species has significantly increased since the early 1970s. Of these, the most significant discoveries with respect to the Glen Murray penguin have been Kairuku grebneffi (Ksepka et al. Citation2012) and K. waitaki (Ksepka et al. Citation2012) from North Otago and South Canterbury in the South Island of New Zealand (). The two described Kairuku species and the Glen Murray penguin all lived during the Oligocene and were similarly sized giants. The respective Canterbury and Waikato basin depositional environments for Kairuku and the Glen Murray penguin were potentially contiguous habitats during the Oligocene given the wide ranges of large extant penguins (Ancel et al. Citation1992; King Citation2000). Crucially, the preserved fossil bones in common between Kairuku and the Glen Murray penguin contain morphological features used to diagnose Kairuku. Here, we review the morphology, taxonomy and implications of the Glen Murray penguin in light of recent discoveries.
Figure 1. Locations of Kairuku depositional environments. A, Kairuku sp. was recovered from the Waikato province in the North Island of New Zealand and Kairuku grebneffi and Kairuku waitaki were collected in the South Island; B, hypothesised extent of subaerial land surface during the Late Oligocene (27 Ma), as adapted from King (Citation2000) (equivalent map for Early Oligocene not available); C, palaeogeographic reconstruction of the Kairuku sp. collection location during the lower Whaingaroan (34.6–29.8 Ma), as adapted from Kamp et al. (Citation2014a).
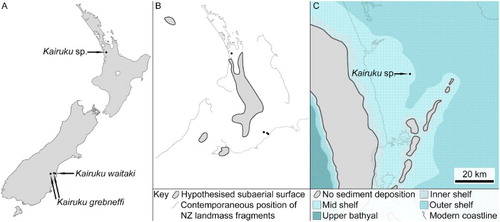
Geological setting
The Glen Murray penguin is from the Glen Massey Formation (Te Kuiti Group; Kear & Schofield Citation1959; White & Waterhouse Citation1993) and was collected from farmland near Glen Murray in the North Island of New Zealand (New Zealand fossil record number R13/f7034; Grant-Mackie & Simpson Citation1973; Edbrooke Citation2001) (). The fossil location is mapped within the geology of the Auckland area (Edbrooke Citation2001) but is within the political bounds of the Waikato District (Statistics New Zealand Citation2006). The fossil bones are from ex situ blocks of massive, glauconitic fine-grained sand that crops out through a paddock along the northern bank of the Awaroa Stream ‘some 650 m upstream from the Glen Murray-Woodleigh road and 25 m above the stream level’ (Grant-Mackie & Simpson Citation1973). The exposures of glauconitic sand are interspersed with calcareous concretionary horizons containing up to 12 cm long fossil oyster shells (gen. et sp. indet.). Weathered outcrop surfaces are orange with iron-staining and freshly exposed surfaces are yellow to grey.
The collection location is within the mapped area for the Glen Massey Formation (Purser Citation1961; Edbrooke Citation2001; Kamp et al. Citation2014a) and the sedimentology is consistent with the Dunphail Siltstone member (Kear & Schofield Citation1959). The upper age limit for the Glen Massey Formation is lower Whaingaroan stage (Kamp et al. Citation2014a, Citation2014b). However, the micropalaeontology reported by Grant-Mackie & Simpson (Citation1973) is inconsistent with that known for the Glen Massey Formation. Specifically, Globigerina ciperoensis Bolli, Citation1954, Globigerina euapertura Jenkins, Citation1960 and Bolivina pontis Finlay, Citation1939 from the host matrix would indicate an upper Whaingaroan age (29.8–27.3 Ma; Grant-Mackie & Simpson Citation1973; Hornibrook et al. Citation1989; Raine et al. Citation2015). Kamp et al. (Citation2014b) observe that the upper Whaingaroan ‘is defined as strata lacking Subbotina angiporoides and containing Notorotalia stachei’ and reiterate Jenkins (Citation1966) and Jenkins (Citation1971) when stating that ‘the lower Whaingaroan is defined as those beds lacking Globigerapsis index and containing Subbotina angiporoides and Globigerina ampliapertura’. The discrepancy between the upper Whaingaroan age from Grant-Mackie & Simpson (Citation1973) and the lower Whaingaroan age from Kamp et al. (Citation2014a) may have arisen from the misidentification of Globigerina ciperoensis. Without Globigerina ciperoensis, the foraminiferal age diagnosis from Grant-Mackie & Simpson (Citation1973) would have been broadened to include the entire Whaingaroan stage (34.6–27.3 Ma; Hornibrook et al. Citation1989; Raine et al. Citation2015). We analysed a small sample of sediment from the Glen Murray penguin fossil locality to see if we could recover the same microfossil fauna reported by Grant-Mackie & Simpson (Citation1973). About 200 g of fresh, unweathered sediment from the penguin fossil locality was placed in boiling water until it disaggregated, which took approximately 1 h. Water and the entrained fine sediment fraction were decanted and the bulk of the sediment was left to dry. The sediment was not sieved prior to study under a microscope. In a subsample of the disaggregated sediment we identified only benthic foraminifera: Cibicides thiara Stache, Citation1864; Globocassidulina subglobosa Brady, Citation1881; Lenticulina gyroscalpra Stache, Citation1864; and Melonis dorreeni Hornibrook, Citation1961. We did not find Gaudryina reussi Stache, Citation1864 or Notorotalia stachei Finlay, Citation1939, which have been reported from other samples of Dunphail Siltstone (Kamp et al. Citation2014b). Our microfossil analysis did not resolve the discrepancy in reported ages and instead yielded a Runangan to Waitakian age (36.7–21.7 Ma) (Hornibrook et al. Citation1989; Kamp et al. Citation2014b); this is consistent with the observation from Kamp et al. (Citation2014b) that ‘shallow water assemblages [from Glen Massey Formation], lacking planktic foraminifera, are difficult to differentiate from Runangan assemblages’ While we acknowledge that our analysis may have been biased by not using a sieve when decanting sediment, we did not find clear evidence for an upper Whaingaroan age (i.e. Grant-Mackie & Simpson Citation1973). However, based on the stratigraphic position of the fossil location, we revise the age of the Glen Murray penguin to lower Whaingaroan (34.6–29.8 Ma).
The Glen Massey Formation was deposited along the western continental shelf of Zealandia during a major Cenozoic marine transgression (Nelson & Hume Citation1987; King Citation2000; Kamp et al. Citation2014a; Strogen et al. Citation2014). Following the separation of Zealandia from Gondwana, the proto-New Zealand landmass drifted north and steadily subsided (discussed in Scott et al. Citation2014 and references therein). The progressive drowning of the subcontinent is recorded in sedimentary sequences around New Zealand (e.g. Canterbury and Waikato basins; Kear & Schofield Citation1959; Browne & Field Citation1985). The Dunphail Siltstone member within the Waikato Basin was deposited close to the peak drowning of Zealandia and represents a mid-shelf environment (Kamp et al. Citation2014a). A marine strait may have extended between the Waikato Basin and the Taranaki Basin and provided foraging opportunities for penguins (Kamp et al. Citation2014a). All reported South Island Kairuku specimens were collected from the Kokoamu Greensand in the Canterbury Basin (Gage Citation1957), which represents an outer- to mid-shelf depositional environment in the late Oligocene (29–30 to 26 Ma; Ayress Citation1993; Raine et al. Citation2015). The Glen Murray penguin thus comes from a proto-Waikato region that is a broadly similar in both age and depositional setting to the South Island Kairuku penguins. While several other penguin taxa are known from Kokoamu Greensand (including Archaeospheniscus lowei Marples, Citation1952; Archaeospheniscus lopdelli Marples, Citation1952; Duntroonornis parvus Marples, Citation1952; and Platydyptes novaezealandiae Oliver, Citation1930), no other fossil penguins have been reported from the Glen Massey Formation. This may be an artefact of differential collecting effort which has historically been concentrated in the south.
Materials and methods
Osteological and orientation terminology follows Baumel & Witmer (Citation1993). Sphenisciformes and Spheniscidae are used in the sense of Clarke et al. (Citation2003): Sphenisciformes is used for all penguins sharing the loss of aerial flight and Spheniscidae restricted to the penguin crown clade (the most recent common ancestor of all extant penguins and its descendants). The fossils are deposited in the University of Auckland Geological Collections, Auckland, New Zealand (accession number UA V005). These specimens were compared with bones of emperor penguins Aptenodytes forsteri Gray, Citation1844 to assess ontogeny: USNM 346257 (adult), USNM 536434 (unfledged juvenile) and USNM 560769 (chick), and also to other fossil specimens: Burnside Formation ‘Palaeeudyptes’ (OM GL435 C48:73-81); Palaeeudyptes marplesi (OM GL429 C50:25-47); Kairuku grebneffi (OU 22065); K. waitaki (OU 12652 + OU22094); and Palaeeudyptes klekowskii (Myrcha et al. Citation1990) (IB/P/B-0369, photo provided by P. Jadwiszczak). Institutional abbreviations: USNM—National Museum of Natural History, Smithsonian Institution, Washington DC, USA; OM—Otago Museum, Dunedin, New Zealand; OU—Otago University Geology Museum, Dunedin, New Zealand; UA—University of Auckland Geology Collections, Auckland, New Zealand.
Systematic palaeontology
Aves Linnaeus, Citation1758 (sensu Gauthier, Citation1986).
Sphenisciformes Sharpe, Citation1891 (sensu Clarke et al. Citation2003).
Kairuku Ksepka et al., Citation2012.
Kairuku sp.
Material
UA V005a-t: Nearly complete right femur (V005a,b; ), partial right tibiotarsus (V005 g,h-n; –), distal half of right tarsometatarsus (V005c,d; proximal half preserved as an impression) (), three incomplete left metatarsal trochlea (V005e), and one pedal phalanx (V005o,p; anatomical position uncertain) and other bone fragments (V005f,q-t). Specimen collected by Mrs J.P. Simpson in 1971.
Figure 2. Glen Murray penguin (Kairuku sp.) right femur. The robustness index of the femur is 0.156, which is comparable to other Kairuku species.
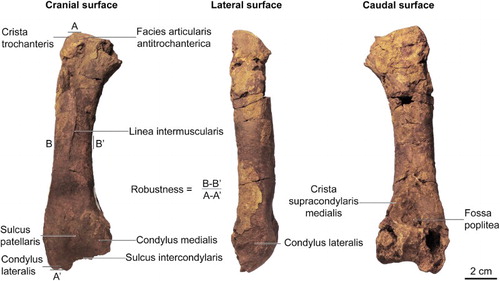
Figure 3. Glen Murray penguin (Kairuku sp.) incomplete right tibiotarsus. The rounded medial border in distal view is an autapomorphy for Kairuku.
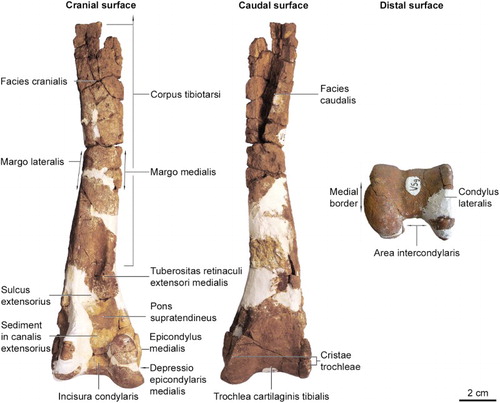
Figure 4. Variation in tibiotarsus morphology between similarly sized fossil penguin species. The distal surfaces of the Kairuku species show a strong to moderate convex projection of the medial condyle. Likewise, the Glen Murray penguin (Kairuku sp.) shows moderate medial projection. The medial projection is less pronounced in Palaeeudyptes klekowskii and the Burnside Formation ‘Palaeeudyptes’. P. klekowskii image supplied by P. Jadwiszczak. Burnside Formation ‘Palaeeudyptes’ image supplied by M. Richards and Otago Museum (Dunedin, New Zealand).
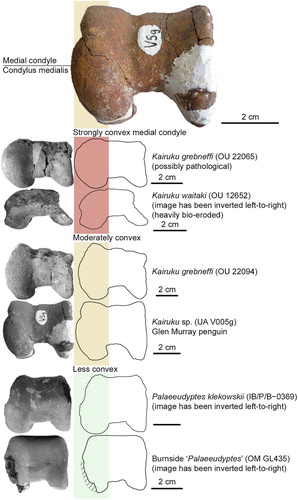
Referral
Ksepka et al. (Citation2012) identified three autapomorphies for Kairuku: (1) femur midshaft width greater than 15% of the total length; (2) a strongly convex medial border of the tibiotarsus in distal view; and (3) absence of crista medialis hypotarsi (tarsometatarsus). UA V005 exhibits trait (1) and an intermediate condition for trait (2) with a moderately convex medial border of the tibiotarsus (more well developed than other penguins, but less so than in K. waitaki and K. grebneffi). Trait 3 cannot be observed due to preservation. UA V005 differs from K. waitaki and K. grebneffi in the presence of a large interosseus space between os metatarsale III and os metatarsale IV. The femur, tibiotarsus and tarsometatarsus are also larger than the equivalent bones in K. waitaki and K. grebneffi, but these size differences may not be diagnostic given the small sample size available. Considering all features, a referral to Kairuku can be supported but the UA V005 cannot be assigned to either K. waitaki or K. grebneffi.
Locality and horizon
Glen Massey Formation, Waikato District, New Zealand (about 37.49°S 174.96°E; New Zealand Fossil Record File R13/f7034 [formerly N51/f1034]); lower Whaingaroan stage (uppermost Priabonian to Rupelian), early Oligocene, 34.6–29.8 Ma (Raine et al. Citation2015).
Measurements
See .
Table 1. Measurements from the Glen Murray penguin (Kairuku sp.) and other ‘giant’ New Zealand penguins.
Description
The caput femorale is missing but the remainder of the proximal femur is intact, with the facies articularis antitrochanterica gently rising towards a rounded crista trochanteris. There is no raised surface on the lateral edge for the attachment of m. iliofemoralis externus and m. iliotrochanteris caudalis. Erosion on the cranial surface has removed the distal end of the trochanter femoris and the proximal end of the linea intermuscularis. The remaining section of the linea intermuscularis has strong relief as it runs along the middle third of the diaphysis. The shaft is nearly straight in cranial view. The cranial surface immediately distal to the linea intermuscularis line is broad and smooth, before narrowing into a deep sulcus patellaris that continues into a deep sulcus intercondylaris. The width of the sulcus patellaris is approximately one third of the distal femur width. The condylus medialis and condylus lateralis are approximately equal in size on the cranial surface and are not preserved on the caudal surface. The lateral edge is partially preserved and the epicondylus lateralis and trochlea fibularis (fibular semicondyle sensu; Elzanowski Citation2008) have been eroded away. The medial edge is also partially preserved and does not have a distinct epicondyle or impressio ansae m. iliofibularis. Erosion at the proximal end of the caudal surface has removed the attachment site for m. obturator medialis; the diaphysis on the caudal surface is smooth and shows no evidence for a strong linea intermuscularis. A deep fossa poplitea and prominent crista supracondylaris medialis are preserved.
The proximal half of the right tibiotarsus has been heavily eroded and the proximal quarter is missing. Erosion has exposed the cross section of the tibiotarsus at approximately midshaft level, revealing a 3–7 mm thick wall of osteosclerotic compacta around a hollow medullary cavity. The preserved lower section of the shaft widens and becomes more craniocaudally compressed towards the distal end of the bone. Erosion at the distal end has removed much of the condylus lateralis including the sulcus m. fibularis. The medial tuberositas retinaculi extensori is prominent and sits approximately midway along the length of the sulcus extensorius. Although sediment fills the canalis extensorius, the incisura intercondylaris is still recognisable as a deep feature between the two large condyles. The distal border of the condylus medialis projects medially and has a convex margin.
The distal half of the right tarsometatarsus is preserved although the plantar surface is embedded in sediment. The incomplete foramen vasculare proximale laterale is expanded distally so as to become confluent with the deepened sulcus longitudinalis dorsalis lateralis, which together create a large interosseus space between os metatarsale III and os metatarsale IV. Although such an elongate space is observed in immature individuals of extant penguins, UA V005 otherwise appears to represent a mature individual and so we interpret this as a diagnostic character rather than an ontogenetic feature. In contrast to the sulcus longitudinalis dorsalis lateralis, the sulcus longitudinalis dorsalis medialis is very shallow. Likewise, complete fusion of os metatarsale II and os metatarsale III suggest post-fledge maturity: in juveniles of the emperor penguin Aptenodytes forsteri a large interosseus space remains opens between both os metarsale II and III and between os metatarsale III and IV. In dorsal view, trochlea metatarsi II projects to nearly the same distal extent as trochlea metatarsi III, whereas trochlea metatarsi IV is retracted well proximal to this level. The plantar-medial corner of trochlea metatarsi II has been eroded and reconstructed, precluding ascertainment of its shape. A foramen vasculare distale is absent.
Comparison
The right hindlimb bones of the Glen Murray penguin (hereafter Kairuku sp.) indicate that it was within the top echelon of penguin body size (broadly shared by Arthrodytes Ameghino, Citation1905; Anthropodytes Simpson, Citation1959; Anthropornis; Icadyptes Clarke et al., Citation2007; Inkayacu Clarke et al., Citation2010; Kairuku; Pachydyptes; and Palaeeudyptes). However, the stout femoral proportions of Kairuku sp. are shared only with K. grebneffi and K. waitaki. The femur robustness ratio (midshaft width ÷ length) of Kairuku sp. is 0.156, compared with 0.154 for K. grebneffi and 0.155 for K. waitaki, whereas this ratio is ≤0.14 in other giant penguins (Ksepka et al. Citation2012). Although features of the femur have only rarely been included in the diagnoses of fossil penguin species, additional traits can be considered as well. The tab-like process and widened and sharply distally tapering condylus medialis of Incayaku (Clarke et al. Citation2010) and the convex facies articularis antitrochanterica in Perudyptes Clarke et al., Citation2007, are absent in Kairuku sp. Autapomorphies identified for Madrynornis Acosta Hospitaleche et al., Citation2007 include a trochanter femoris that is much more proximal than the caput femorale. Although the caput femorale is missing in Kairuku sp., the low trochanter femoris suggests that this feature was not present, and it can also be observed to be absent in K. grebneffi and K. waitaki.
The right tibiotarsus of Kairuku sp. is slightly larger than the left and right tibiotarsi reported for K. grebneffi (Ksepka et al. Citation2012). The maximum mediolateral width at the distal end is 48 mm, compared with 42 mm for the right tibiotarsus of K. grebneffi (OU22065) and 41 mm for the left tibiotarsus of K. grebneffi (OU12652). Damage to the proximal end prevents a comparison of maximum length; however, the tibiotarsi have similar distal proportions and the craniocaudal compression reported for the South Island Kairuku specimens is evident in Kairuku sp. In distal view, the condylus medialis has a strongly convex medial edge in K. grebneffi, whereas the tibiotarsus is both crushed and eroded in the holotype of K. waitaki but can be observed to have at least a moderately pronounced medial edge (). The medial edge of the tibiotarsus in Kairuku sp. is also convex and has a moderate projection. In K. grebneffi, K. waitaki and Kairuku sp., the condylus medialis projects medially beyond the epicondylus medialis. This is unlike Palaeeudyptes marplesi (OM GL429 C50:25-47) and Burnside ‘Palaeeudyptes’ (OM GL435 C48:73-81), where the medial margin does not have a strong medial projection. The diagnostic projection of the cnemial crests (e.g. crista cnemialis cranialis) in Inkayacu cannot be compared with Kairuku sp. (Clarke et al. Citation2010).
The distal tarsometatarsus of Kairuku sp. is wider than in K. waitaki, Palaeeudyptes antarcticus and Palaeeudyptes klekowskii (Ksepka et al. Citation2012; Acosta Hospitaleche Citation2014). A large foramen vasculare proximale laterale and a reduced foramen vasculare proximale mediale are traits shared by Kairuku sp., K. grebneffi, K. waitaki, Palaeeudyptes, Inkayacu, Palaeospheniscus and Eretiscus Olson Citation1986 (Wiman Citation1905; Simpson Citation1971b; Myrcha et al. Citation2002; Clarke et al. Citation2010; Ksepka et al. Citation2012). However, the large interosseus space continuous with the foramen vasculare proximale laterale is otherwise observed only in some specimens of Spheniscus Brisson, 1760 (e.g. Chávez-Hoffmeister Citation2014, ). The projection of trochlea metatarsi II to nearly the level of trochlea metatarsi III in dorsal view (i.e. distal extension of trochlea II) is a common trait for Kairuku sp., K. grebneffi and K. waitaki. The hypotarsus has not been preserved and cannot be examined for the presence/absence of the crista medialis hypotarsi that is considered an autapomorphy for Kairuku (Ksepka et al. Citation2012).
Discussion
The Glen Murray penguin shares two of the three autapomorphies identified for Kairuku in the analysis by Ksepka et al. (Citation2012), but differs from K. grebneffi and K. waitaki in several discrete features. We therefore refer the Glen Murray penguin to Kairuku, although we consider erecting a new species to be premature pending the discovery of more complete material. Although Kairuku sp. and Pachydyptes ponderosus do not share common skeletal elements, we consider the difference in geological age between these species and the identification of autapomorphies for Kairuku in the former as evidence that these giant penguins are not conspecific.
Our best estimate for the body size of Kairuku sp. is through a comparison with K. grebneffi, as both species have similarly sized and proportioned hindlimb bones. The standing height of K. grebneffi has been estimated at c. 1.28 m and Kairuku sp. was likely taller (from as little as 2% to as much as 20% based on comparable limb measurements). The correlation between femur cross section and total body mass suggests that the hindlimb bones of Kairuku sp. may be sensitive predictors of body mass in future analyses (Habib Citation2010). Larger-bodied penguins today forage in deep waters and may be ecological models for extinct giant penguins (Davis & Renner Citation2004). The Dunphail Siltstone host lithology for Kairuku sp. has been interpreted as a mid-shelf depositional environment, potentially indicating an offshore foraging site for the ancient penguin (Kear & Schofield Citation1959; White & Waterhouse Citation1993; Davis & Renner Citation2004; Kamp et al. Citation2014a).
Zealandia became increasingly coastal across the Oligocene and the image emerging for penguins on this drowning subcontinent is one of higher diversity (Fordyce & Jones Citation1990; Ksepka et al. Citation2012). There are 10 species of ancient penguin described from Zealandia inside the 11 million year span of the Oligocene, and several other known fossils that may represent additional penguin species (Fordyce & Jones Citation1990; Ksepka & Ando Citation2011; Ksepka et al. Citation2012). The Glen Murray penguin documents an additional distinct species, further increasing this diversity estimate. Oligocene rocks in New Zealand have been productive for marine vertebrates with the majority of published discoveries from South Island localities. Kairuku sp. is significant for showing that giant Oligocene penguins occur in North Island sediments. Reconstructions of Zealandia during the Oligocene hypothesise that regions of the modern-day North Island and South Island were once joined into a large central landmass (King Citation2000). The Kairuku sp. fossil locality was offshore along the northwestern edge this landmass, compared with the southeastern locations of K. grebneffi and K. waitaki that were offshore from the same drowning landmass. As we continue to resolve the species richness of Oligocene penguins in Zealandia, we will discover how the diversity of this group responded to the changing landscape.
Acknowledgements
We thank N Hudson and JA Grant-Mackie for discussion and access to the specimen. P Jadwiszczak, M Richards and Otago Museum provided images for this study. H James and the National Museum of Natural History, Smithsonian Institution provided access to modern comparative material.
Disclosure statement
No potential conflict of interest was reported by the authors.
ORCiD
DB Thomas http://orcid.org/0000-0001-7679-0991
Additional information
Funding
References
- Acosta Hospitaleche C. 2014. New giant penguin bones from Antarctica: systematic and paleobiological significance. Comptes Rendus Palevol. 13:555–560. doi: 10.1016/j.crpv.2014.03.008
- Acosta Hospitaleche C, Tambussi CP, Donato M, Cozzuol M. 2007. A new Miocene penguin from Patagonia and its phylogenetic relationships. Acta Palaeontologica Polonica. 52:299–314.
- Ameghino F. 1905. Enumeración de los impennes fósiles de Patagonia y de la isla Seymour. Anales del Museo Nacional de Historia Natural de Buenos Aires. 3:97–167.
- Ancel A, Kooyman GL, Ponganis PJ, Gendner JP, Lignon J, Mestre X, Huin N, Thorson PH, Robisson P, Le Maho Y. 1992. Foraging behaviour of emperor penguins as a resource detector in winter and summer. Nature. 360:336–339. doi: 10.1038/360336a0
- Ando T, Fordyce RE. 2014. Evolutionary drivers for flightless, wing-propelled divers in the Northern and Southern Hemispheres. Palaeogeography, Palaeoclimatology, Palaeoecology. 400:50–61. doi: 10.1016/j.palaeo.2013.08.002
- Ayress MA. 1993. Ostracod biostratigraphy and palaeoecology of the Kokoamu Greensand and Otekaike Limestone (Late Oligocene to Early Miocene), North Otago and South Canterbury, New Zealand. Alcheringa. 17:125–151. doi: 10.1080/03115519308619491
- Baumel JJ, Witmer LM. 1993. Osteologia. In: Baumel JJ, King AS, Breazile JE, Evans HE, Van den Berge JC, editors. Handbook of avian anatomy: nomina anatomica avium. 2nd ed. Cambridge, MA: Publications of the Nuttall Ornithological Club; p. 45–132.
- Bolli HM. 1954. Note on Globigerina concinna Reuss 1850 (Point-a-Pierre, Trinidad). Contributions from the Cushman Foundation for Foraminiferal Research. 5:1–3.
- Brady HB. 1881. Notes on some reticularian Rhizopoda of the Challenger expedition, Part 3. Q J Microsc Sci. 21:31–71.
- Brodkorb P. 1963. Catalogue of fossil birds. Part 1 (Archaeopterygiformes through Ardeiformes). Bull Florida State Mus, Biol Sci. 7:179–293.
- Browne GH, Field BD. 1985. The lithostratigraphy of Late Cretaceous to Early Pleistocene rocks of northern Canterbury, New Zealand. Lower Hutt: New Zealand Geological Survey. 63 p.
- Chávez-Hoffmeister, MF. 2014. The humerus and stratigraphic range of Palaeospheniscus (Aves, Sphenisciformes). Ameghiniana. 51:159–172.
- Clarke JA, Ksepka DT, Salas-Gismondi R, Altamirano AJ, Shawkey MD, D’Alba L, Vinther J, DeVries TJ, Baby P. 2010. Fossil evidence for evolution of the shape and color of penguin feathers. Science. 330:954–957. doi: 10.1126/science.1193604
- Clarke JA, Ksepka DT, Stucchi M, Urbina M, Giannini N, Bertelli S, Narváez Y, Boyd CA. 2007. Paleogene equatorial penguins challenge the proposed relationship between biogeography, diversity, and Cenozoic climate change. P Natl Acad Sci USA. 104:11545–11550. doi: 10.1073/pnas.0611099104
- Clarke JA, Olivero EB, Puerta P. 2003. Description of the earliest fossil penguin from South America and first Paleogene vertebrate locality of Tierra del Fuego, Argentina. Am Mus Novit. 3423:1–18. doi: 10.1206/0003-0082(2003)423<0001:DOTEFP>2.0.CO;2
- Davis LS, Renner M. 2004. Penguins. New Haven: Yale University Press; 224 p.
- Edbrooke SW. 2001. Geology of the Auckland area: scale 1:250,000. Lower Hutt: Institute of Geological & Nuclear Sciences Limited.
- Elzanowski A. 2008. The avian femur: morphology and terminology of the lateral condyle. Oryctos. 7:1–5.
- Finlay HJ. 1939. New Zealand Foraminifera: key species in stratigraphy — no. 3. Trans Proc Roy Soc New Zeal. 69:309–329.
- Fordyce RE, Jones CM. 1990. Penguin history and new fossil material from New Zealand. In: Davis LS, Darby JT, editors. Penguin biology. San Diego: Academic Press; p. 419–446.
- Fordyce RE, Thomas DB. 2011. Kaiika maxwelli, a new Early Eocene archaic penguin (Sphenisciformes, Aves) from Waihao Valley, South Canterbury, New Zealand. New Zeal J Geol Geop. 54:43–51. doi: 10.1080/00288306.2011.536521
- Forster JR. 1781. Historia aptenodytae, generis avium orbi avstrali proprii. Novi Commentarii Societatis Regiae Scientiarum Gottingensis. 3:121–148.
- Gage M. 1957. The geology of Waitaki subdivision. New Zeal Geol Surv Bull (n.s.). 55:1–135.
- Gauthier JA. 1986. Saurischian monophyly and the origin of birds. In: Padian K, editor. The origin of birds and the evolution of flight. San Francisco: California Academy of Sciences; p. 1–55.
- Grant-Mackie MJA, Simpson DGG. 1973. Tertiary penguins from the North Island of New Zealand. J Roy Soc New Zeal. 3:441–451. doi: 10.1080/03036758.1973.10421867
- Gray GR. 1844. Aptenodytes. Ann Mag Nat Hist. 13:315.
- Habib M. 2010. The structural mechanics and evolution of aquaflying birds. Biol J Linn Soc. 99:687–698. doi: 10.1111/j.1095-8312.2010.01372.x
- Hornibrook NdB. 1961. Tertiary Foraminifera from Oamaru district (NZ). Part 1—Systematics and distribution. Lower Hutt: New Zealand Geological Survey Paleontological Bulletin; pp. 1–192.
- Hornibrook Nd, Strong CP, Brazier RC. 1989. Manual of New Zealand Permian to Pleistocene foraminiferal biostratigraphy. Lower Hutt: New Zealand Geological Survey Paleontological Bulletin.
- Huxley TH. 1859. On a fossil bird and a fossil cetacean from New Zealand. Q J Geol Soc London. 15:670–677. doi: 10.1144/GSL.JGS.1859.015.01-02.73
- Jenkins DG. 1960. Planktonic foraminifera from the Lakes Entrance Oil Shaft, Victoria, Australia. Micropaleontology. 6:345–371. doi: 10.2307/1484217
- Jenkins DG. 1966. Planktonic foraminiferal zones and taxa from Danian to Lower Miocene of New Zealand. New Zeal J Geol Geophys. 10:1064–1078. doi: 10.1080/00288306.1967.10423209
- Jenkins DG. 1971. New Zealand Cenozoic planktonic foraminifera. Lower Hutt: New Zealand Geological Survey Paleontological Bulletin.
- Kamp PJJ, Tripathi ARP, Nelson CS. 2014a. Paleogeography of Late Eocene to earliest Miocene Te Kuiti Group, central-western North Island, New Zealand. New Zeal J Geol Geophys. 57:128–148. doi: 10.1080/00288306.2014.904384
- Kamp PJJ, Tripathi ARP, Nelson CS, Hendy AJW. 2014b. Biostratigraphy, Sr isotope chronology and chronostratigraphy of the Late Eocene – earliest Miocene Te Kuiti Group, Waikato – King Country Basin, New Zealand, Ministry of Business, Innovation and Employment, New Zealand, unpublished Petroleum Report.
- Kear D, Schofield JC. 1959. Te Kuiti group. New Zeal J Geol Geophys. 2:685–717. doi: 10.1080/00288306.1959.10422764
- King PR. 2000. Tectonic reconstructions of New Zealand: 40 Ma to the Present. New Zeal J Geol Geophys. 43:611–638. doi: 10.1080/00288306.2000.9514913
- Ksepka DT, Ando T. 2011. Penguins Past, Present, and Future: Trends in the Evolution of the Sphenisciformes. In: Dyke G, Kaiser G, editors. Living Dinosaurs. Chichester, UK: John Wiley & Sons; p. 155–186.
- Ksepka DT, Bertelli S, Giannini NP. 2006. The phylogeny of the living and fossil Sphenisciformes (penguins). Cladistics. 22:412–441. doi: 10.1111/j.1096-0031.2006.00116.x
- Ksepka DT, Fordyce RE, Ando T, Jones CM. 2012. New fossil penguins (Aves, Sphenisciformes) from the Oligocene of New Zealand reveal the skeletal plan of stem penguins. J Vertebr Paleontol. 32:235–254. doi: 10.1080/02724634.2012.652051
- Linnaeus C. 1758. Systema naturae per regna tria naturae, secundum classes, ordines, genera, species, cum characteribus, differentiis, synonymis, locis. Stockholm: Laurentii Salvii.
- Marples BJ. 1952. Early Tertiary penguins of New Zealand. New Zeal Geol Sur, Paleontol Bull. 20:1–66.
- Mayr G, Scofield RP. 2014. First diagnosable non-sphenisciform bird from the early Paleocene of New Zealand. J Roy Soci New Zeal. 44:48–56. doi: 10.1080/03036758.2013.863788
- Mayr G, Scofield RP. 2016. New avian remains from the Paleocene of New Zealand: the first early Cenozoic Phaethontiformes (tropicbirds) from the Southern Hemisphere. J Vertebr Paleontol. 36:e1031343-1–e1031343-5.
- Mortimer N. 2004. New Zealand’s geological foundations. Gondwana Res. 7:261–272. doi: 10.1016/S1342-937X(05)70324-5
- Myrcha A, Jadwiszczak P, Tambussi CP, Noriega JI, Gaździcki A, Tatur A, Del Valle RA. 2002. Taxonomic revision of Eocene Antarctic penguins based on tarsometatarsal morphology. Polish Polar Res. 23:5–46.
- Myrcha A, Tatur A, Valle RD. 1990. A new species of fossil penguin from Seymour Island, West Antarctica. Alcheringa: An Austral J Palaeontol. 14:195–205. doi: 10.1080/03115519008619055
- Nelson CS, Hume TM. 1987. Paleoenvironmental controls on mineral assemblages in a shelf sequence: Te Kuiti Group, South Auckland, New Zealand. New Zeal J Geol Geophys. 30:343–362. doi: 10.1080/00288306.1987.10427540
- Oliver WRB. 1930. New Zealand birds. Wellington: Fine Arts; 541 p.
- Olson SL. 1986. A replacement name for the fossil penguin Microdytes Simpson (Aves: Spheniscidae). J Paleontol. 60:785–0. doi: 10.1017/S0022336000022332
- Purser BH. 1961. Geology of the Port Waikato region (Onewhero sheet N51). Wellington: Government Print.
- Raine JI, Beu AG, Boyes AF, Campbell HJ, Cooper RA, Crampton JS, Crundwell MP, Hollis CJ, Morgans HEG. 2015. Revised calibration of the New Zealand geological timescale. GNS Science Report 2012/3953.
- Scott JM, Lee DE, Fordyce RE, Palin JM. 2014. A possible Late Oligocene–Early Miocene rocky shoreline on Otago Schist. New Zeal J Geol Geophys. 57:185–194. doi: 10.1080/00288306.2013.814575
- Sharpe RB. 1891. A review of recent attempts to classify birds. Proceedings of the Second International Ornithological Congress. 2:1–90.
- Simpson GG. 1959. A new fossil penguin from Australia. Proc Roy Soc Victoria. 71:113–119.
- Simpson GG. 1971a. A review of the pre-Pleistocene penguins of New Zealand. B Am Mus Nat Hist. 144:319–378.
- Simpson GG. 1971b. Conspectus of Patagonian fossil penguins. Am Mus Novitates. 2488:1–37.
- Slack KE, Jones CM, Ando T, Harrison GL, Fordyce RE, Arnason U, Penny D. 2006. Early penguin fossils, plus mitochondrial genomes, calibrate avian evolution. Mol Biol Evol. 23:1144–1155. doi: 10.1093/molbev/msj124
- Stache G. 1864. Die Foraminiferen der tertiären Mergel des Whaingaroa-Hafens (Provinz Auckland). Novara-Expedition, 1857–1859, Vol. 1 Geologische Theil No. 2:159–304.
- Statistics New Zealand. 2006. Boundary Map of Waikato Region. [cited 2016 June 17]Available from: http://www.stats.govt.nz/Census/2006CensusHomePage/Boundary/BoundaryMap.aspx?id=1000003&type=region&ParentID=
- Strogen DP, Bland KJ, Nicol A, King PR. 2014. Paleogeography of the Taranaki Basin region during the latest Eocene–Early Miocene and implications for the ‘total drowning’ of Zealandia. New Zeal J Geol Geophys. 57:110–127. doi: 10.1080/00288306.2014.901231
- White PJ, Waterhouse BC. 1993. Lithostratigraphy of the Te Kuiti Group: a revision. New Zeal J Geol Geophys. 36:255–266. doi: 10.1080/00288306.1993.9514573
- Wiman C. 1905. Vorläfige Mitteilung über die alttertiären Vertebraten der Seymourinsel. Bull Geol Inst Uppsala. 6:247–253.