ABSTRACT
Over the past decade, episodic memory has been reconceptualised as future-oriented. In 2007, Schacter and I proposed the ‘constructive episodic simulation hypothesis’ to account for emerging findings that past and future thinking share overlapping cognitive and neural substrates, arguing that the constructive nature of episodic memory provides the perfect neurocognitive architecture for future simulation. Here, I update and refine this theoretical position, arguing that, fundamentally, episodic memories are not special. I shift the emphasis from conceptualising memory as the basis of imagination, instead proposing that both are instantiations of the same constructive simulation process. I synthesise emerging neuroscientific perspectives to show how the brain’s default mode network underpins the remembering, imagining and perception of event representations via a common simulation process that is constructive and iterative, involving the interaction of pre-existing knowledge and reinstated perceptual content with incoming experiential information to create and refine online mental simulations of experience.
Donna Rose Addis.
Introduction: back to the future
Looking only at the 130 years of research since Ebbinghaus (Citation1885/Citation1964) launched the modern study of memory, one would be convinced that memory is conceptualised as the mental remnants of past experience. Looking back further, however, ancient and medieval scholars considered memory to be intrinsically linked with various forms of imagination and future-oriented thinking, including the formation of intentions, plans and expectations, as well as the pursuit of goals (O’Callaghan Citation2016). A century after Ebbinghaus, these ideas resurfaced with Ingvar’s (Citation1985) essay on ‘memory for the future’ (i.e. prospective memory) and Tulving’s (Citation1985) observation that amnesic patient KC could not imagine the future. Tulving coined the term ‘mental time travel’—to describe this ability to mentally re-experience the past and ‘pre-experience’ the future. Thus, 1985 can be seen as the beginning of a zeitgeist that has seen a radical shift in the conceptualisation of memory.
Since then, numerous findings from psychology and cognitive neuroscience have demonstrated strong links between past and future thinking, including similar modulation of phenomenological characteristics with the temporal closeness of events and individual differences in imagery and emotion regulation, similar developmental trajectories during childhood and older age, and parallel declines in depression and amnesia (for reviews, see Schacter & Addis Citation2007; Schacter et al. Citation2012). In 2007, the overlap of past and future thinking was brought to the forefront of psychology and cognitive neuroscience by three concurrent studies reporting that brain regions traditionally linked with memory are also involved in imagining the future; together these studies were named one of Science’s Top Ten Breakthroughs for 2007 (Addis, Wong et al. Citation2007; Hassabis et al. Citation2007; Szpunar et al. Citation2007; see also Okuda et al. Citation2003). Two of these studies developed paradigms to elicit both memories of past events and simulation of novel future events during functional MRI (fMRI) scanning, revealing robust activation of the default mode network (DMN) when thinking about past and future events (see ; Addis, Wong et al. Citation2007; Szpunar et al. Citation2007). Hassabis et al. (Citation2007) found that patients with amnesia were unable to imagine future events with the level of detail and coherence of healthy control participants, a deficit strikingly similar in nature to their loss of memory for past events. That same year, my collaborator Dan Schacter and I proposed the ‘constructive episodic simulation hypothesis’ to account for the overlap between remembering past events and imagining future events. Within our framework, the term ‘simulation’ [verb] refers to the constructive process of building a mental rendering of an externally experienced and/or internally generated episode (i.e. a ‘simulation’ [noun], ‘event representation’ or ‘event/situation model’). To the degree that our experiences are multimodal, so too are these events representations, comprising perceptual and conceptual information about external components of the event such as context (e.g. spatial and/or temporal), entities (e.g. people, objects, etc.), actions, plot and dialogue as well as internal experiences such as emotions, bodily sensations and thoughts (e.g. evaluations, beliefs and activation of associated information); and schematic information that provides a structure to the representation (for a similar terminology and definitions, see Levine et al. Citation2002; Diana et al. Citation2007; Irish & Piguet Citation2013; Szpunar et al. Citation2014; Rubin & Umanath Citation2015; Robin & Moscovitch Citation2017). Our use of the term ‘episodic’ reflects our focus on event representations. Although Tulving’s (Citation1972) definition restricts the usage of ‘episodic’ to specific events that occur in a unique spatiotemporal context, it is likely that representations of repeated experiences are similar, comprising similar types content including perceptual, conceptual and schematic information (Addis et al. Citation2004; Rubin & Umanath Citation2015). We argued that the constructive nature of the episodic memory system provides the perfect neurocognitive architecture to flexibly extract and recombine details from episodic memories to simulate future events (Schacter & Addis Citation2007). In other words, we redefined the function of episodic memory as primarily future-focused (Schacter et al. Citation2007).
Figure 1. Example trials from the fMRI paradigm used by Addis, Wong et al. (Citation2007) are shown in (i). Participants had 20 seconds to recall or imagine an event falling within the specified time period in response to the cue word, or to complete a semantic control task (generating two words related to the cue word, putting all three into a sentence and defining the words), before rating the amount of detail generated. The past and future conditions engaged the default mode network relative to the control task (ii). Example trials from the adapted autobiographical interview used by Addis et al. (Citation2008) are shown in (iii). The tasks were the same as in (i) but participants had 3 minutes to describe the event aloud. Transcripts were then scored according to Levine et al.’s (Citation2002) protocol (iv), where details are parsed (forward slashes) and classified as episodic (black) or non-episodic (grey italics). Panel (ii) is adapted from Addis, Wong et al. (Citation2007) with permission.
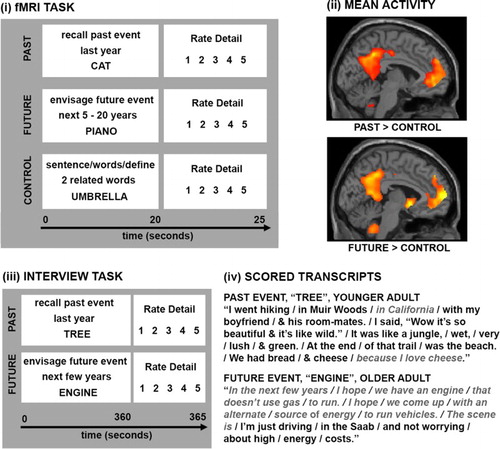
In this article, I draw on historical and contemporary perspectives from philosophy, psychology and cognitive neuroscience to update and refine this theoretical position. I argue for a change in emphasis, from the notion that imagination relies on episodic memory, to considering both abilities as instantiations of the same process: constructive episodic simulation. And while remembered and imagined event representations may differ in some respects, such as temporal direction, content and/or phenomenological properties, I argue that these differences are superficial. At a fundamental level, memories are not special or different from imaginings; both are complex, multimodal event representations constructed via the integration of schema and informational elements stored in content-specific areas of the cortex. Although I focus on memory and imagination herein, later in this article I expand this hypothesis to argue that constructive episodic simulation also underpins the generation of an online representation of current perceptual experience (which is then encoded as an episodic memory). And, as such, remembering, imagining and even perceiving events are subserved by the same cognitive processes and brain networks. In particular, the DMN is the brain’s event simulator: it flexibly interacts with other brain networks representing event content and schemas—and, when required by task or situational demands, with attention and cognitive control networks —to construct, encode and reconstruct all variety of event simulations.
Are memory and imagination the same?
A central tenet of the constructive episodic simulation hypothesis is that remembering past events and imagining future events are linked processes, whereby imagination relies on the episodic memory system (Schacter & Addis Citation2007). However, a subtly different possibility is that both remembering and imagining are instantiations of the same process: constructive episodic simulation. Whether memory and imagination are the same is not a new question, and over millennia it has been approached by examining the similarities and differences between: (1) remembered and imagined event representations; and (2) the cognitive system(s) and process(es) generating these representations.
Philosophical perspectives
Aristotle posited that a core function of the ‘basic perceiving mind’ is to collect discrete ‘imprints’ (mental images) of past perceptual experiences that can then be recombined to construct dreams, creative visualisations and expectations of the future. Nevertheless, Aristotle maintained that memory and imagination are distinct due to differences in content (De Brigard Citation2016), namely that memory is about past experiences that have occurred (and are true) while imagination and expectation are about things that have not happened (and are false). While past and future events vary in their veridicality, the content of the event representations themselves do not differ in any fundamental way, as both remembered and imagined events are grounded in experience. And as De Brigard (Citation2014, Citation2016) points out, we can also imagine the past (i.e. counterfactual thinking) and remember the future (i.e. prospective memory), so temporal direction is an irrelevant difference (see also Schacter et al. Citation2012).
Medieval philosophers Ibn Sīnā and Ibn Rushd (known in the West as Avicenna and Averroes, respectively) and Thomas Aquinas considered the existence of multiple internal faculties (i.e. cognitive systems) that receive and retain perceptual information from external experience, as well as the internal generation of inferences assigned to the perceptual experience (e.g. salience, meaning and even spatiotemporal context; Black Citation2000; De Brigard Citation2016; O’Callaghan Citation2016). Critically, they each conceived of some form of compositive imagination that actively combines stored information to create novel representations (Black Citation2000). However, while Ibn Sīnā posited that compositive imagination is a separate system that interacts with information stored in other faculties, for Thomas Aquinas it was more parsimonious to conceive of the retentive system(s) not only retaining information but also recombining it into novel forms (O’Callaghan Citation2016). In other words, Aquinas believed that the same cognitive system underpins both memory and imagination.
Most scholars, however, have held to the Aristotelian view that imagination and memory are distinct entities. In De Brigard’s (Citation2016) survey of historical philosophical views on memory, he notes that Descartes and Hume agreed that memories of real experiences are more ‘lively’ (i.e. vivid and dynamic) and cohere better with our beliefs than imagined representations. More recently, others have argued that the critical difference between remembered and imagined representations is not phenomenology, but the presence/absence of an accompanying apperception indicating that the representation is a memory: a sense of pastness (Locke Citation1694/Citation1970), consciousness (James Citation1890) or belief (Russell Citation1921) that the remembered event has happened before (cf. autoneotic consciousness, Tulving Citation1985, which he argues is a feature of both past and future events). De Brigard notes that Spinoza thought this inference of pastness is derived from temporal information contained within the memory, such as the duration of perceptual experience. Some modern theorists (e.g. Urmson Citation1967) locate this apperception in metacognition: the awareness of cognitive demands, such as the intention to remember (although it is not clear then how spontaneously-retrieved memories that lack explicit intentions would be tagged as memories). Mahr & Csibra (Citation2017) argue that every episodic memory is connected with a separate meta-representation that the event happened in reality, providing an ‘epistemic attitude of remembering’. Aside from the addition of a mnemonic apperception, memories are considered otherwise identical to imaginations (e.g. Russell Citation1921). Interestingly, however, according to these views the defining characteristic of an imagination is the absence of an apperception linking it to the past. This stands in contrast to the dominant view in contemporary psychology, the source monitoring framework (Johnson et al. Citation1993), which considers the unique aspects of both memories and imaginings as critical to how these representations are distinguished from each other.
Are remembered and imagined representations the same?
The source monitoring framework (Johnson et al. Citation1993) integrates many of the aforementioned ideas into a comprehensive theory of how we determine whether a mental representation is based on a real (external) experience or an imagined (internal) one. Considerable support has accumulated for this framework (for a review, see Mitchell & Johnson Citation2009), including empirical findings demonstrating that memories and imagined events differ not only in content, but also in phenomenology and the subjective experiences associated with bringing these representations to mind. Relative to imagined representations, memories typically have more sensory detail (e.g. D’Argembeau & van der Linden Citation2004; Addis et al. Citation2009), contextual clarity (D’Argembeau & van der Linden Citation2004) and consistency with pre-existing knowledge that makes them more plausible (Roberts, Wiebels et al. Citation2017). These attributes give rise to subjective experiences such as feelings of pastness, re-experiencing and/or familiarity (e.g. see Daselaar et al. Citation2008 for evidence linking reactivation of content to subjective experience). In contrast to memories, imagined events are often novel relative to previous experiences (Addis, Cheng et al. Citation2011), more difficult to construct than memories (van Mulukom Citation2013) and the resulting event representations may also include memory for the imagination process itself (e.g. remembering the cognitive operations of generating an idea, forming novel associations, making inferences, engaging in reasoning and/or changing content to make the representation more coherent and plausible; Johnson et al. Citation1993). Despite these differences between memories and imaginings, Mitchell & Johnson (2009, p. 641) nevertheless acknowledge that ‘memories for events are infused with our knowledge, beliefs, and desires, as well as products of our imagination’.
A recent theory from philosopher Michaelian (Citation2011) expands on this latter point, positing that remembered events do not differ from imagined events at all, in that both are forms of imagination (for similar views, see also Taylor & Schneider Citation1989; De Brigard Citation2014). Michaelian highlights that an episodic memory contains elements of the original experience as well as other ‘imagined’ content that was not part of, but relevant to, the original experience, such as details from other experiences, conceptual information and schemas such as event scripts (e.g. going to a restaurant) and self-schema that aid in the reconstruction process (Bartlett Citation1932; Rubin & Umanath Citation2015; Robin & Moscovitch Citation2017). In this way, Michaelian argues, event memories are identical to imagined representations where episodic details from multiple (and usually related) experiences are brought together with the aid of a relevant event schema (Irish & Piguet Citation2013) to construct an event representation. With respect to subjective experience, Michaelian argues that fluency signals whether or not a representation has been experienced previously (cf. Jacoby & Dallas Citation1981), pointing out that this is the most parsimonious explanation because fluency is an attribute of both remembering and imagining.
Building on this argument (Michaelian Citation2011; De Brigard Citation2014), I suggest that remembered and imagined events are the same type of representation in that both are simulations of experience constructed from the same pool of experiential details and under the guidance of the same schemas. While there may be some differences in the relative proportions of content types, with remembered events typically containing more perceptual content than imagined events for instance (e.g. D’Argembeau & van der Linden Citation2004), this is only a matter of degree because the types of details comprising these representations are overwhelmingly the same. For instance, a spatiotemporal context is a defining feature of an episodic representation (Tulving Citation1972), and it has been argued that, in particular, the spatial scene is fundamental to representations of both memories and hypothetical events (cf. scene construction hypothesis; Hassabis & Maguire Citation2007: for similar views, see also Rubin & Umanath Citation2015; Robin & Moscovitch Citation2017). In addition, an event representation typically contains sensory (particularly visuospatial) and conceptual information about the objects and other people featuring in the event, about the self as an observer of the event and/or as the subject of the experience itself, as well as an unfolding sequence of actions, consequences, dialogue, thoughts and emotions (Levine et al. Citation2002). Indeed, strong correlations between past and future events in terms of the number of episodic or non-episodic details have been consistently observed. For instance, in a series of studies, Addis et al. (Citation2008, Citation2010) used the Autobiographical Interview (Levine et al. Citation2002) to probe the episodic and non-episodic content of past and future events (see ). Specifically, younger (aged 18–35 years) and older adults (aged over 65 years) described past and future events in response to word cues; the transcripts were then scored by parsing event descriptions into informational elements and classifying each element as either episodic or non-episodic. In both studies, older adults generated significantly fewer episodic and more non-episodic details than younger adults, and, importantly, these decreases/increases were of similar magnitude for past and future events. Moreover, the number of episodic details comprising past and future events were significantly correlated, both within and across the age groups.
Failures of source monitoring suggest that these types of representations are, in fact, easily confusable. Misattribution errors typically occur when an imagined representation has the features of a memory, such as being vividly detailed and plausible; in other words, the verisimilitude of the imagined representation causes it to be confused with reality (Johnson et al. Citation1993). The likelihood of these errors is increased by the depth of imagination, which inflates the degree of confidence in that event having actually occurred (i.e. imagination inflation; Garry et al. Citation1996). For example, providing perceptual detail (e.g. in the form of a doctored photograph) and/or opportunities to imagine detail related to the hypothetical scenario can increase later false memory for an entire experience that never occurred (Wade et al. Citation2002). Similarly, we have shown that imagining hypothetical past events based on recombinations of autobiographical details increases the acceptance of autobiographical conjunction errors, a type of memory error whereby a detail from another experience is mistakenly retrieved as part of a memory (Devitt et al. Citation2016). In addition to causing an increase in perceptual detail, repeatedly imagining an event significantly increases the plausibility of that event (Szpunar & Schacter Citation2013), likely by increasing the congruence between the imagined event and relevant schemas.
Does the same cognitive system underpin memory and imagination?
If remembered and imagined events are overwhelmingly similar in terms of representational content and phenomenology, does this mean necessarily that these representations map onto the same neurocognitive system and processes? According to the constructive episodic simulation hypothesis, the answer to this question is (largely) yes; imagination relies on the episodic memory system (Schacter & Addis Citation2007; Addis & Schacter Citation2012). On the basis of cognitive and neural commonalities between past and future events, we proposed that imagined events are built from details in episodic memory using various processes ascribed to the episodic memory system. Specifically, we argued that, while remembering involves re-activation of the details comprising an episode as well as relational processing to re-integrate these details into a representation that is then re-encoded, imagining a hypothetical event requires activation and recombination of relevant episodic details followed by the encoding of the newly-constructed event representation (Addis & Schacter Citation2012).
However, in this article, I now shift the emphasis from the ‘episodic’ in constructive episodic simulation to the ‘constructive’ and ‘simulation’, by suggesting that instead of conceptualising imagination as relying on episodic memory, we should consider memory and imagination as but two products of a constructive simulation system (cf. Taylor & Schneider Citation1989; Michaelian Citation2011; De Brigard Citation2014) that is also central to how we understand—and even perceive—the ongoing stream of experience (Chen et al. Citation2016). In other words, the same simulation process, which involves content activation, integration and encoding, is used to build simulations of internal and/or external experience, although the balance of different forms of content may differ depending on whether we are currently experiencing, remembering or imagining the episode.
From a neurocognitive perspective, it is likely that an event representation is physically instantiated in the brain in exactly the same way irrespective of whether it is remembered or imagined; it is a set of connections between the nodes representing perceptual content, semantic information and related schemas. As aforementioned, remembered representations will typically contain more perceptual elements than imagined events where semantic and schematic information may be pivotal, especially for novel imaginings (Irish & Piguet Citation2013). These differences in proportions of different types of representational content will necessarily result in some differences in the spatial distribution of activity across the brain when remembering and imagining, even though the same simulation process is engaged and the same types of information are drawn upon. For instance, in studies where subjective detail ratings for remembered and imagined events are similar, we have failed to find any differential activity during remembering over and above that evident during imagining (e.g. Addis, Wong et al. Citation2007). However, when past events are rated as more detailed than future events, differential activation of posterior visual cortices during remembering have emerged (Addis et al. Citation2009). In older adults, who tend to generate event representations with more semantic, conceptual detail, we have observed increased activity in anterolateral temporal cortex relative to younger adults (Addis, Roberts et al. Citation2011). Thus, differences in activation patterns between memory and imagination do not necessarily mean that different constructive processes are at play.
We have previously argued that an important difference is that imagination requires the ‘recombination’ of details for the first time to construct a novel event representation and is thus distinct from the process of re-integrating the details comprising a memory (Schacter & Addis Citation2007; Addis & Schacter Citation2012). However, this difference may be better conceptualised as a difference in associative ‘history’ (i.e. whether the details comprising the representation have been associated previously) rather than distinct processes per se. I suggest that the degree of associative strength between constituent details (Horner & Burgess Citation2013) is manifest as the fluency of the event. Indeed, consistent with higher levels of associative strength, memories are brought to mind more easily and quickly than imagined events being constructed de novo (Anderson et al. Citation2012; van Mulukom Citation2013). Thus, it is unlikely that imagination requires engagement of unique cognitive processes and brain networks but that the same neurocognitive architecture (i.e. the brain's simulation system) can support both the construction and reconstruction of event representations.
Constructive episodic memory: a framework for understanding event simulation
Bartlett (Citation1932) observed that memory is not a literal replay of a past event; remembered information can be incomplete, or may contain additional information that often times reflects dominant schema, current concerns and beliefs, and/or newly encountered information (Schacter et al. Citation1998). Our understanding of the role of episodic memory in constructive episodic simulation developed out of a re-interpretation of these memory errors as a valuable and adaptive by-product of the constructive nature of memory that enables it to facilitate not only retrieval but also imagination (Schacter & Addis Citation2007).
Within cognitive neuroscience it is well-established that a representation of a past experience is stored as a pattern of features distributed across the brain (Fuster Citation1997; Mitchell & Johnson Citation2009; Rugg & Vilberg Citation2013), such that ‘no single [brain] location contains a complete record of the trace or engram of a specific experience’ (Schacter et al. Citation1998, p. 291). Each of the nodes that process an aspect of an experience in the present moment also go on to store the respective features of the representation. For instance, early sensory areas store the most fine-grained elements of experience, while higher level association areas store more integrated, multimodal representations of longer ‘chunks’ of experience (Chen et al. Citation2016). The hippocampus is at the apex of this hierarchy; having reciprocal connections with virtually all of the cortex, it is well-positioned anatomically to represent highly-integrated records of experience, in line with its established capacity for relational processing (Eichenbaum Citation2001). Thus it is not surprising that the hippocampus plays a critical role in both the encoding of event representations, when the features of a specific experience are bound together into a unique representation (i.e. pattern separation), and during retrieval, when the constituent features are reactivated and reintegrated to reconstruct the memory (i.e. pattern completion) (McClelland et al. Citation1995).
The reinstatement of event features is dependent on interactions between the hippocampus and the particular cortical regions mediating the content of a given memory. Damage to content-specific regions, such as the visual cortex, can lead to retrograde amnesia, as critical parts of the memory representation are lost (Rubin & Umanath Citation2015). Moreover, neuroimaging research has provided compelling evidence to support the notion of distributed event representations at the level of the cortex (Danker & Anderson Citation2010; Rugg & Vilberg Citation2013). For instance, Woodruff et al. (Citation2005) showed that during episodic retrieval, if participants are presented with one fragment of a multi-sensory experience (e.g. the picture from a picture-word pairing), there was associated reactivation of cortex mediating the other aspect of the memory (fusiform ‘visual word area’) even though that category of stimuli was not presented. Not only are reinstatement effects evident for perceptual content, but also for associated stimuli, emotional responses and strategic processing (see Danker & Anderson Citation2010 for a comprehensive review). Recent work leveraging multivariate techniques, such as representational similarity analyses, has demonstrated that the patterns of activity evident during encoding are significantly correlated with those evident at retrieval, even for representations of complex life-like events, and that the degree of reinstatement in the brain correlates with the vividness of re-experiencing (Bird et al. Citation2015; St-Laurent et al. Citation2015).
Episodic retrieval is a dynamic and flexible process and the raft of memory distortions and errors that can potentially occur provide insight into constructive retrieval processes. Being stored as a collection of associated features means that a memory representation can be retrieved in a different form each and every time it is remembered, depending on the specific features that are reinstated. In turn, the features retrieved are likely influenced by the availability and/or weighting of content features in accordance with retrieval goals (Mitchell & Johnson Citation2009) and schema (Bartlett Citation1932). For instance, while schemas can facilitate the process of reconstructing event representations, particularly when cues are impoverished (Robin & Moscovitch Citation2017), schemas can also bias it. Memories may be modified to fit with our general beliefs about the world (e.g. cultural schemas; Bartlett Citation1932) and with what Conway & Pleydell-Pearce (Citation2000) call the ‘working self’, a self schema that includes active personal goals, self-beliefs and trait self-knowledge. For instance, a memory that is incompatible with our schema may be edited or false memories may be generated to maintain the coherence of the self (Ross & Wilson Citation2003). In addition to bias, newly encountered information (Loftus Citation2005) or related memories (Morton et al. Citation2017) may be incorporated into the event representation. Autobiographical conjunction errors, for instance, are a relatively common memory error (Devitt et al. Citation2016), and if one encounters misinformation following an experience (e.g. during eye-witness questioning), it can become incorporated into the representation of the original experience (for a review on the misinformation effect, see Loftus Citation2005). Moreover, Carpenter & Schacter (Citation2017) recently demonstrated that the successful recombination of event details during an associative inference task resulted in an increase of false memories on a source memory test.
These errors may persist over time because during retrieval, the reactivated representation becomes temporarily labile (and thus modifiable) before it is reconsolidated (e.g. Hupbach et al. Citation2007); a related concept is re-encoding, whereby the reactivated memory as well as one’s internal and external context are encoded as a new (but distinct) memory representation resulting in multiple traces of the same experience (Nadel & Moscovitch Citation1998). Therefore, an a similar vein, if a representation of a past event is activated without particular elements, this content may be lost from the memory representation when it is reconsolidated, as has been demonstrated when the emotional component of a traumatic memory is blocked with propranolol during retrieval (Brunet et al. Citation2008). Nevertheless, reconsolidation is generally viewed as adaptive in that it allows a memory representation to be updated with relevant information encountered after the event, and enables the formation of linkages between related events that contribute to the formation and ongoing refinement of broader knowledge structures such as schemas (Schlichting & Preston Citation2016; Morton et al. Citation2017).
From an evolutionary standpoint, that a cognitive system designed to hold a veridical record of the past is so error-prone appears unfavourable. However, we have argued that the function of memory is not archival or retrospective, but rather constructive and prospective (Schacter & Addis Citation2007). The fact that the constituent elements of episodic memories are stored independently actually facilitates mental simulation: details are easily extractable from different episodic memories, and can then be recombined to construct simulations of hypothetical events, including future events. Thus, a central tenet of our constructive episodic simulation hypothesis is that, although the episodic memory system is error-prone with respect to remembering the past, it is nevertheless aptly designed to support the mental simulation of events (Schacter & Addis Citation2007). When used with a view to the future, this simulation ability confers many adaptive benefits such as increased problem solving particularly for open-ended or ill-defined problems (e.g. Sheldon et al. Citation2011), creativity (e.g. Madore et al. Citation2017), enhanced coping with stressful upcoming situations (e.g. Taylor & Schneider Citation1989) and more advantageous decision-making (e.g. Benoit et al. Citation2011) raising the intriguing possibility that the human brain, or at least episodic memory, was designed for prospection (Schacter et al. Citation2007; see also Klein Citation2013). Our focus on the error-prone nature of episodic memory does not imply, however, that there is no need for accuracy in episodic memory (Mahr & Csibra Citation2017); to be useful, simulations need to draw on representations of past experience that have some basis in reality (Schacter et al. Citation2018). Moreover, we do not exclude a role for semantic memory in simulation (Irish & Piguet Citation2013). Indeed, as discussed later, schematic and semantic memory play a critical role in constructive episodic simulation.
The default mode network is the brain’s simulation system
Default mode engagement during simulation
The brain’s DMN includes the medial temporal lobes, medial aspects of the frontal and parietal cortices, inferior lateral parietal cortex and lateral temporal cortex. Although this same set of brain regions has been described previously as the autobiographical retrieval network (Maguire Citation2001), the recollection network (Rugg & Vilberg Citation2013) and the common core network (i.e. common to both remembering past events and imagining future events; Schacter & Addis Citation2007), it is now widely known as the DMN because of its ubiquitous activity when individuals are not engaged in a cognitive task requiring external attention (e.g. reading words). It has become increasingly apparent, however, that DMN activity is not really about the absence of a cognitive task, but rather the mind wandering to the past and the future when not otherwise occupied (Spreng Citation2012). In other words, our simulation system is engaged when we are in our default mode and mind-wandering, and this network can be modulated according to whether the cognitive task at hand requires simulation (e.g. episodic memory tasks; Spreng Citation2012).
Over the past decade, numerous neuroimaging studies have examined the boundary conditions of DMN engagement, using experimental paradigms that elicit the remembering or the online imagining of events (see for an example). The results of these studies indicate that simulating a variety of different events and scenarios engages the DMN, whether remembered or imagined, and irrespective of temporal direction (imagined past versus imagined future events), temporality (past/future events versus atemporal events), spatiotemporal dynamics (static scenes versus navigation) and perspective (self versus other) (for reviews, see Buckner & Carroll Citation2007; Schacter et al. Citation2012). Interestingly, even the passive perception of stories, through reading narratives (e.g. fiction) or watching films, engages the DMN (e.g. Mar Citation2004; Hasson et al. Citation2015). Simulation in service of autobiographical planning (Spreng et al. Citation2010), problem solving (Gerlach et al. Citation2011) and creativity (Ellamil et al. Citation2012; Madore et al. Citation2017) also engages the DMN, in conjunction with other networks (e.g. dorsal attention network, frontoparietal control network), depending on the nature of the task. For example, in a creativity study that involved artists sketching book covers in the scanner, the generation of ideas (divergent thinking) was associated with activity in DMN regions while the evaluation of ideas (convergent thinking) recruited aspects of the frontoparietal control network, demonstrating the interplay between these networks.
Moreover, the level of engagement of particular regions in the DMN is influenced by the content of the simulation, such as the amount and familiarity of details comprising event representations (Addis & Schacter Citation2008; Szpunar et al. Citation2009) and the relative amounts of perceptual, conceptual and goal-related content (Addis et al. Citation2009; D’Argembeau et al. Citation2010; Addis, Cheng et al. Citation2011). For instance, we included in our fMRI analyses subjective ratings of the amount of detail comprising events remembered and imagined in the scanner, and found that posterior hippocampal activity during event construction increased with the detail ratings comprising past and future simulations (Addis & Schacter Citation2008). In contrast, while activity in the anterior hippocampus was also modulated by detail, it was limited to future events. We interpreted this future-specific modulation as reflecting the increased recruitment of detail recombination processes when imagining events relative to remembering events where the details have occurred together previously (i.e. where the associative strength is higher). In line with this notion, imagining events that load more highly on detail recombination, such as novel future events (i.e. that have never been previously imagined; e.g. van Mulukom et al. Citation2013), implausible future events (Weiler et al. Citation2010) and specific future events (i.e. that are specific in place and time; Addis, Cheng et al. Citation2011), have also been associated with increased activity in the anterior hippocampus.
That the DMN plays a role in simulation is thus well-established. But what are the functional contributions of the DMN to simulation? The hippocampus has featured prominently in extant explanations as a key region mediating critical episodic and constructive processes. Two pieces of evidence have contributed to this hippocampal focus: the observation that hippocampal amnesics are significantly impaired in imagining detailed and integrated scenes (e.g. Hassabis et al. Citation2007; but see Squire et al. Citation2010), as well as neuroimaging findings that the anterior hippocampus is significantly more engaged during the construction of future events relative to the reconstruction of episodic memories (Addis, Wong et al. Citation2007; Addis, Cheng et al. Citation2011) and responds to the amount of detail comprising future not past events (Addis & Schacter Citation2008). Maguire and colleagues have argued that the critical role of the hippocampus lies in building a spatially-coherent scene within which a simulated event unfolds (cf. scene construction theory, Hassabis & Maguire Citation2007). From the perspective of the constructive episodic simulation hypothesis (Schacter & Addis Citation2007), we have offered a more generalised account, arguing that this hippocampal mechanism reflects flexible relational processing that supports the construction of a range of simulations (including scenes), and that can integrate disparate forms of information (including scenes along with other forms of content) to construct complex simulations of temporally-extended episodes (Roberts, Schacter et al. Citation2017). Similar ideas have been proposed by others; for instance, Graham et al. (Citation2010) argued that the hippocampus creates complex conjunctions of scenes, objects and self, and Diana et al. (Citation2007) suggest that the hippocampus binds items with contexts to create ‘situation models’ (i.e. simulations of events).
Outside of the hippocampus, some DMN regions are thought to mediate specific types of representational content comprising simulations, such as general and autobiographical semantic knowledge (lateral temporal cortex; Binder et al. Citation2009), schemas including self-schemas (medial prefrontal cortex; Gilboa & Moscovitch Citation2017), spatial and contextual information (retrosplenial and parahippocampal cortices; Bar & Aminoff Citation2003) and the DMN also couples with other networks depending on content, including but not limited to the visual network (e.g. cuneus, fusiform and lingual gyri; Binder et al. Citation2009). Other DMN regions are thought to be more process-oriented: the precuneus supports episodic and visuospatial imagery (Cavanna & Trimble Citation2006), while the inferior lateral parietal cortex (e.g. angular gyrus) mediates the retrieval and binding of details into complex simulations (e.g. Thakral, Madore et al. Citation2017) and/or attention to these inner representations (Cabeza et al. Citation2012). Additionally, specific task demands may require DMN connectivity with the frontoparietal control and dorsal attention networks, such as when using simulations for autobiographical planning (Spreng et al. Citation2010) and problem solving (Gerlach et al. Citation2011); and with the ventral attention (salience) network when simulations are emotionally- or cognitively-salient (e.g. theory of mind; Spreng & Grady Citation2010: e.g. creative cognition; Ellamil et al. Citation2012; Beaty et al. Citation2015; Madore et al. Citation2017).
Emerging perspectives relevant to the DMN’s contributions to simulation
Two emerging perspectives cast new light on the functional contributions of the DMN to constructive episodic simulation. One perspective regards the functional segregation of the hippocampus along its long axis. Accumulating evidence suggests its functions are organised along an anterior–posterior gradient according to the resolution of the information being processed (Sheldon & Levine Citation2016; Robin & Moscovitch Citation2017). Specifically, neuroimaging evidence indicates that the anterior portion of the hippocampus processes coarse and large-scale informational units, such as extended event narratives (Collin et al. Citation2017), semantic concepts (Sheldon & Levine Citation2016) and schemas (Mack et al. Citation2016). In contrast, the posterior hippocampus processes fine-grained perceptual, spatial and temporal details (Collin et al. Citation2017).
Indeed, these portions of the hippocampus exhibit distinct profiles of anatomical and functional connectivity that reflect this purported division of labour (Kahn et al. Citation2008; Adnan et al. Citation2016). The anterior hippocampus is strongly connected with anterolateral temporal and medial prefrontal cortices that mediate abstract, high-level knowledge structures such as semantic concepts and schemas. For instance, neuroimaging and animal research indicates that the bidirectional interactions between schemas and memory representations during encoding and retrieval are mediated by reciprocal anatomical pathways between the anterior hippocampus and medial prefrontal cortex (Eichenbaum Citation2017; Morton et al. Citation2017), including evidence of increased functional connectivity between these regions during episodic encoding that results in schema formation (Mack et al. Citation2016; Schlichting & Preston Citation2016), during the initial stage of episodic retrieval where schema are critical for memory search (McCormick et al. Citation2015) and during the retrieval of goal-relevant memories in rodents (Place et al. Citation2016). In contrast, the posterior hippocampus has strong connections with posterior cortical regions mediating perceptual information and processes, particularly those in the visuospatial domain, such as the parahippocampal cortex, medial parietal cortex, ventral temporal cortex and early visual areas. Posterior hippocampal connectivity with these regions is associated with reinstatement of fine-grained sensory details, particularly when the event representation is being elaborated in detail (McCormick et al. Citation2015; Adnan et al. Citation2016).
The second perspective comes from two related theories of how the continuous stream of experience is processed in the present moment: event segmentation theory (Zacks et al. Citation2007) and the hierarchical process memory framework (Hasson et al. Citation2015; Chen et al. Citation2016; Baldassano et al. Citation2017). These theories have been built on cutting-edge research examining the neurocognitive correlates of processing of narratives/stories and movies, arguably experimentally-controlled versions of internally-generated event representations (e.g. imagining scenarios when listening to a story) and externally-experienced events (e.g. seeing events unfold in a movie). By these accounts, present-moment experience is perceived and processed in a hierarchical manner and in relation to relevant schemas, and results in the construction of an ‘event model’ (cf. ‘situation model’; Diana et al. Citation2007)—an inner representation of current experience. Specifically, as incoming unimodal information moves from early sensory regions to higher-order regions, it is associated with related content from other modalities and from across longer durations of experience (e.g. discrete phonemes are inherently shorter than more integrated representations of sentences or narratives). Interestingly, the higher-level regions that represent longer stretches of ongoing experience have slower neural dynamics, and overlap considerably with the DMN (Chen et al. Citation2016; Baldassano et al. Citation2017), and thus proponents of this perspective suggest that the DMN accumulates and holds ongoing experience online as an integrated event representation until the context changes (i.e. an event boundary; e.g. a shift in attention from inner thoughts to the external environment, a move in location, an entry of someone new into current experience, etc.). This idea is conceptually similar to Baddeley’s (Citation2000) episodic buffer, the component of working memory that he argued integrated all currently-perceived multimodal information into a coherent representation. And all the while perceptual information from current experience is accumulated and integrated to form an event representation, this representation is also being refined, filled in and updated by inputs from past experience and learning (e.g. activation of pre-existing schemas, associated semantic information and event representations) as well as newly perceived information, in an iterative manner.
The initial formation of an online event representation is thought to be mediated by the higher-order associative cortices comprising the DMN, specifically the angular gyrus, posterior cingulate, medial parietal and prefrontal cortices (Chen et al. Citation2016; Baldassano et al. Citation2017). These regions represent different forms of integrated content, including multimodal perceptual content and some degree of spatial and contextual information (as mediated by the posterior parietal regions) as well as schematic information (mediated by medial prefrontal cortex). While considerable research implicates the hippocampus in binding the components of an event representation (Eichenbaum Citation2001) there is evidence to suggest that these other DMN regions can form an event representation independently of the hippocampus. The observation that a hippocampal amnesic can sustain a conversation until the context changes (i.e. evidence of the formation of an online representation that captures the duration of the conversation which is then never encoded into episodic memory) is particularly compelling, and consistent with neuroimaging findings of recollection effects in these extra-hippocampal regions (Rugg & Vilberg Citation2013).
However, it is likely that, under most circumstances, the construction of coherent event representations also involves the hippocampus. At the apex of an associative hierarchy, the hippocampus is positioned to integrate the multimodal event model built from perceptual and contextual inputs with other core event information, including spatial information (Zeidman et al. Citation2015), temporal information (Eichenbaum Citation2017) and with relevant pre-existing information including related associations (both episodic and semantic) and schemas (Kroes & Fernández Citation2012; Morton et al. Citation2017; Robin & Moscovitch Citation2017). Critically, I propose that it is this interaction of current experience with pre-existing information that underpins the constructive nature of event simulation, enabling the filling in of missing information, the re-organising of content so that it is schema-consistent, coherent and easily encoded.
Neuroimaging research has demonstrated that hippocampally-mediated encoding occurs after a change in context (i.e. an event boundary; Zacks et al. Citation2007; Baldassano et al. Citation2017), and it is likely that the fully-integrated event representation is transmitted from the hippocampus back to cortical regions representing relevant schema (where it has the potential to update schema; Morton et al. Citation2017) and to regions representing content (where it can influence storage of content, as well as the ongoing processing of current and/or future experience; Fuster, Citation1997; Hasson et al. Citation2015).
Critically, these theories consider most cortical regions as having not only the capacity to process particular forms of information but also to represent and store the processed information over the long term (cf. Fuster Citation1997), and that the associations between the constituent elements of an experience are mediated by reciprocal connectivity between these content-specific cortices and the hippocampus (e.g. McCormick et al. Citation2015). Baldassano et al. (Citation2017) examined neural activity during the perception of life-like stimuli (e.g. a movie and/or narrative), and found that, in particular, the angular gyrus and posterior cingulate represented longer-duration event representations (built from shorter-duration event representations in lower sensory-perceptual regions), exhibited relationships with hippocampal activity following event boundaries (i.e. when encoding is most likely to occur) and reinstated similar activation patterns during retrieval. Additionally, Baldassano et al.’s (Citation2017) finding that angular gyrus, medial parietal and frontal regions exhibited anticipatory reinstatement of relevant event representations when listening to a familiar narrative demonstrates that pre-existing associations and event representations are brought back online, which can then act to influence current perception.
Constructive episodic simulation: a refined model
The synthesis of these emerging perspectives with our current understandings of DMN contributions to event simulation provides the basis for a new theory of constructive episodic simulation under various conditions: perception and encoding of ongoing experience; retrieval of previous experience (i.e. event memory); and imagining hypothetical experience (e.g. future events).
Event perception. While experiencing an episode, incoming perceptual information is processed by sensory cortices and different experiential elements are integrated online to build an event representation in posterior DMN regions (e.g. angular gyrus and posterior cingulate). The hippocampus provides high-level coherence by mediating integration of fine-grained perceptual information from content-specific cortices (provided via posterior hippocampal connections with posterior DMN regions such as posterior cingulate and angular gyrus) with large-scale conceptual information from schemas and semantic memory (provided via anterior hippocampal connectivity with anterior DMN regions such as medial prefrontal cortex and anterolateral temporal cortex). I suggest that the event representation is iteratively constructed and refined online, according to the ongoing stream of experience as well as pre-existing knowledge, via the reciprocal connectivity of the hippocampus with both anterior and posterior DMN, until such time as the event ends (as signalled by a contextual shift) and the current version of the event simulation is encoded by strengthening the active set of associations.
Event memory. Episodic retrieval involves the reconstruction of a previously-encoded simulation by reactivating the relevant associations between the hippocampus and content-specific regions. An event memory can be retrieved in a bottom-up fashion, where incoming perceptual information that overlaps with the event representation can trigger pattern completion when it reaches the posterior hippocampus (‘direct retrieval’; Conway & Pleydell-Pearce Citation2000). In the absence of direct cues, an event memory can be retrieved via a top-down process whereby relevant schema(s) based in medial prefrontal cortex interact with the anterior hippocampus to guide reactivation (‘generative retrieval’; Conway & Pleydell-Pearce Citation2000). Whether accessed via a direct or generative route, the hippocampus then interacts with content-specific cortices to reconstruct the event representation in its entirety: to guide the reinstatement of perceptual elements as well as to re-integrate the various elements once reinstated and brought into current conscious experience. Specifically, the anterior hippocampus interacts with DMN regions representing relevant schema and semantic information (e.g. medial prefrontal and anterolateral temporal cortices), while the posterior hippocampus interacts with regions in sensory networks representing perceptual content. As content is reactivated, higher-level DMN association cortices (e.g. angular gyrus, medial parietal and posterior cingulate cortices) support the accumulation of this internally-generated multimodal information and, in conjunction with the hippocampus, integrate these elements into a coherent event representation. Again, the simulation process is iterative and constructive, with the event representation being refined online by the influence of schema and other event representations as well as current perceptual experience. For instance, retrieved content is assessed against schemas to identify inaccuracies or missing information, in which case related content (e.g. other associations accessed via posterior hippocampus and/or semantic information accessed via anterior hippocampus) is used to fill in gaps in the simulation. Schemas will also guide the modification of content so that the event representation is plausible and meaningful given current goals. Once the context shifts, for instance, one’s attention moves from the retrieved event memory, encoding of the current (retrieved) event representation occurs, which in the case of retrieved representations constitutes reconsolidation.
Event imagination. The simulation of hypothetical events follows a largely similar trajectory to generative event retrieval, starting with the activation of schemas (e.g. a script for the type of event such as going to a restaurant) that provides a framework for the to-be-imagined event. Pertinent semantic information (e.g. knowledge of my favourite restaurants; generalised representations of repeated events such as ‘lunch on Tuesdays with Nanna at Hollywood Café’), as well as relevant perceptual content, is used to populate the event framework provided by the schema. As it is constructed, the event representation is iteratively refined online to increase its coherence and its relevance to current goals, and may also be modified by incoming perceptual information. Again, the simulation is encoded once the context shifts.
This refined model of constructive episodic simulation is consistent with much of the extant evidence, such as widespread DMN engagement for both remembering past events and imagining hypothetical events (Schacter et al. Citation2012). However, instead of limiting the interpretation of this overlapping activity to the retrieval of episodic details necessary when both remembering and imagining, as we have done previously (Schacter & Addis Citation2007), this refined model suggests that the common process is simulation: the online construction of an event representation. This change in emphasis does not mean that the retrieval of episodic (i.e. perceptual) details is not an important component of both remembering and imagining. Indeed, the retrieval of episodic details is tightly associated with the incorporation of episodic details in future events (Addis et al. Citation2008) and the amount of detail comprising both past and future events modulates posterior hippocampal activity (Addis & Schacter Citation2008). Moreover, Schacter & Madore (Citation2016) discuss recent findings that an experimental induction designed to increase access to episodic details impacts an event construction process that is common to both memory and imagination.
Critically, this refined model can also account for apparent differences between imagination and remembering. Previously, we have attributed differential anterior hippocampal activity during the imagining of future events with the increased demands on relational processing when forming novel recombinations of details, as well as the encoding of novel events (Martin et al. Citation2011; van Mulukom et al. Citation2013). Moreover, differential ventromedial prefrontal cortex activation during future thinking has been linked with prospective processes such as forming intentions (Okuda et al. Citation2003; Addis, Wong et al. Citation2007; Addis et al. Citation2009). According to this revised model, however, differential activity in anterior hippocampus and medial prefrontal cortex reflects the heightened dependence on schemas when constructing an imagined event de novo, relative to reinstating a representation of a past event. Specifically, pre-existing knowledge is used to form a large-scale framework for the event (e.g. an event script) that guides the selection and integration of relevant episodic and semantic content to flesh out the simulation (Irish et al. Citation2012). As such, the schema itself becomes an integral component of the event representation and is integrated with information from other modalities existing at different scales (e.g. fine-grained perceptual detail). This ‘scaffolding hypothesis’ arose from our findings from semantic dementia patients who, in the absence of semantic information to create an event framework, cannot imagine specific future events despite the preserved ability to remember past events (Irish et al. Citation2012). Interestingly, however, these patients can recast past events into the future (Irish & Piguet Citation2013). It is likely, then, that reliance on schemas during simulation exists along a continuum whereby it is least necessary for retrieval of event memories, other than to fill in gaps in content or identify inaccuracies in the retrieved representation. Similarly, recasting an event memory as a hypothetical event does not require additional interactivity with schemas and/or semantic information as this is essentially akin to remembering an event memory except with the addition of a belief that the event will reoccur in future. Next on this continuum are imagined general events (e.g. future routines); typically, these event representations are highly similar to past routines and should therefore be less reliant on schemas than constructing specific—and highly novel—future event representations, in line with our finding that anterior hippocampal activity is greater for specific than general future events (Addis, Cheng et al. Citation2011). Interactivity with schemas is arguably most important when generating novel events that fall outside of the realm of current or previous experience (Irish & Piguet Citation2013), providing an explanation for increased anterior hippocampal activity when imagining implausible versus plausible specific events (e.g. Weiler et al. Citation2010). Moreover, during the construction of a simulation, ongoing interactions between the emerging event representation and schemas enable monitoring of the construction process to ensure plausibility and accuracy of the representation with reference to pre-existing knowledge, including personal goals (D’Argembeau et al. Citation2010)—processes that may be more greatly taxed when constructing more unlikely or novel events.
Thus, an increased reliance on schemas when constructing simulations of hypothetical events can account for increased anterior hippocampal and ventromedial prefrontal activity during imagination. In line with this idea, Benoit et al. (Citation2014) found that when imagined events were integrated with richer levels of prior knowledge, ventromedial prefrontal cortex was more engaged and exhibited stronger effective connectivity with content-specific regions. Interestingly, Thakral, Benoit et al. (Citation2017) found that posterior hippocampal activity during future simulation is transient, while anterior hippocampal activity is sustained. Given that regions with slower dynamics have been found to mediate longer-scale representations and vice versa (Chen et al. Citation2016), these findings dovetail with the idea that anterior hippocampus supports the integration of larger-scale schematic frameworks into the event representation, while posterior hippocampus integrates fine-grained perceptual details (see also McCormick et al. Citation2015, Citation2018).
This refined mode of constructive episodic simulation proposes that rapid, iterative interactions between the anterior and posterior portions of the DMN occur via the hippocampus, and that this enables the fine-tuning of the simulation online, according to incoming perceptual information and pre-existing information. This notion fits well with the finding that intra- and inter-hippocampal connectivity can reconfigure dynamically during simulation (McCormick et al. Citation2018). Interestingly, when the construction process is slowed down, such that participants add one feature to the simulation at a time, the anterior hippocampus exhibits phasic activity (Summerfield et al. Citation2010). Fluctuating patterns of activation and connectivity likely reflect small, transient networks (‘process-specific alliances’) that are assembled and disassembled as needed (Cabeza & Moscovitch Citation2013). It is possible that these assemblies shift rapidly enough to support an iterative constructive process, although the frequency of these dynamics remains to be determined with imaging techniques possessing finer temporal resolution than fMRI (e.g. magnetoencephalography).
The focus of the proposed model on anterior and posterior portions of the DMN, connected via the hippocampus, is consistent with resting-state connectivity data demonstrating that medial prefrontal and posterior cingulate cortices are critical hubs of the DMN (Andrews-Hanna et al. Citation2010). In the context of hippocampal damage, it may be that direct (albeit suboptimal) connections between these hubs provide a mechanism for constructing simulations independently of the hippocampus. For instance, while posterior-to-anterior connectivity normally passes through the hippocampus during the retrieval of event memories, patients with hippocampal damage show stronger effective connectivity between medial prefrontal and parietal cortices (Addis, Moscovitch et al. Citation2007). Even so, event representations constructed in the context of hippocampal dysfunction will nevertheless be reduced in detail, and lack coherence (Hassabis et al. Citation2007; Addis et al. Citation2010). Given these observations, the finding that some hippocampal amnesic patients who cannot remember past events nevertheless remain capable of imagining scenes and events (e.g. Squire et al. Citation2010) appears striking. Two possible explanations for this anomalous finding are that patients are recasting past events into the future in lieu of constructing novel imaginings (Irish & Piguet Citation2013), and/or that their event representations are weighted towards their spared schematic and semantic information. The proposed model would suggest that anterior DMN regions could support the provision of semantic details (i.e. lateral temporal cortices) and schemas (medial prefrontal cortex) which could be integrated with multimodal perceptual representations mediated by higher-level posterior DMN regions (e.g. medial parietal and angular gyrus) via direct connections. Therefore, an event representation with some degree of integration, albeit less than what would be yielded by hippocampal involvement, could be generated. This is one of many hypotheses from the proposed model that can be tested in future research.
How is this refined version of the constructive episodic simulation hypothesis different to other recent theoretical perspectives? The proposed model expands the event segmentation theory (Zacks et al. Citation2007) and the hierarchical process memory framework (Hasson et al. Citation2015) to consider: (1) iterative interactions between the event representation under construction and schemas and semantic information represented in anterior DMN regions; and (2) the processes occurring during imagination and how these are inherently similar to the processes occurring during retrieval as well as perception. In Sheldon & Levine’s (Citation2016) model, the anterior hippocampus is argued to be engaged primarily during open-ended tasks (e.g. imagining future events, means-ends problem-solving) as opposed to remembering, due to an increased reliance on conceptual information. However, I argue that schemas are always interacting with event representations, during perception, retrieval, imagination and during open-ended tasks requiring simulation such as problem-solving, though the degree to which this occurs may vary; and that these interactions are bidirectional, enabling both schemas and event simulations to be constantly refined and updated. More research is required, however, to fully explore this iterative simulation process and test the hypotheses presented here. I also suggest that the defining characteristic of simulation in service of cognitive tasks (e.g. planning, problem-solving) is the additional recruitment of the dorsal attention and frontoparietal control networks (e.g. Spreng et al. Citation2010) to direct the simulation process with respect to task demands. We recently found that coupling between these networks emerged when participants imagined future events under challenging demands, such as when the imagined event had to integrate incongruent details (e.g. ‘Dad’, ‘conference poster’, ‘gym’) relative to congruent details (e.g. ‘Mum’, ‘living room’, ‘handbag’) (Roberts, Wiebels et al. Citation2017). Interestingly, frontoparietal regions included anterolateral prefrontal cortex, an area involved in relational reasoning and conceptual expansion that likely assists the creation of a plausible event framework around incongruent details by creating a larger ‘simulation space’ to work within.
Conclusions
In this article I have contextualised contemporary cognitive neuroscience research on episodic memory and imagination within historical perspectives from philosophy and psychology. In doing so, I hope to highlight that the recent reconceptualisation of memory as future-oriented is but a return to very old ideas that have come full circle. Thus, an emergent theme of this review is that, like with simulation, no idea is ever really new; it is rather a novel assemblage of elements from pre-existing ideas. And, as such, I have brought together ideas from various contemporary theories to yield a novel refinement of the constructive episodic simulation hypothesis (Schacter & Addis Citation2007): that both memories and imaginings are event representations produced by the same constructive process and subserved by the same brain networks, primarily the DMN. There is evidence to support the proposed model of constructive episodic simulation, where pre-existing knowledge iteratively interacts with incoming and reinstated perceptual content to construct lifelike renderings of reality with in the mind. Nevertheless, there is much work to do in further elaborating this model and testing specific predictions. And thus, perhaps, it is time for the focus of this field to move beyond the ‘episodic’ to the ‘constructive’ and ‘simulation’, to now explore fully the iterative simulation process by which event representations are constructed and refined, and the ways in which this simulation process is commonly utilised for event perception, memory and imagination. This refined theory has and will generate numerous hypotheses ripe for testing, and I predict that the next decade of research on constructive episodic simulation will be as fruitful and as enlightening as the last.
Acknowledgements
I am deeply grateful and humbled to have been named a Fellow of the Royal Society of New Zealand. The research behind this Fellowship, like the ideas described in this paper, reflect the many rewarding and fruitful collaborations I have been lucky to enjoy over my career, with my mentors, peers and students, all of whom have helped, inspired and challenged me in my thinking about autobiographical memory and imagination. I gratefully acknowledge Daniel Schacter, Mary Pat McAndrews and Reece Roberts for their helpful comments on this manuscript, as well as anonymous reviewers.
Disclosure statement
No potential conflict of interest was reported by the author.
ORCID
Donna Rose Addis http://orcid.org/0000-0002-6231-1491
Additional information
Funding
References
- Addis DR, Cheng T, Roberts RP, Schacter DL. 2011. Hippocampal contributions to the episodic simulation of specific and general future events. Hippocampus. 21:1045–1052. doi: 10.1002/hipo.20870
- Addis DR, Moscovitch M, Crawley AP, McAndrews MP. 2004. Recollective qualities modulate hippocampal activation during autobiographical memory retrieval. Hippocampus. 14:752–762. doi: 10.1002/hipo.10215
- Addis DR, Moscovitch M, McAndrews MP. 2007. Consequences of hippocampal damage across the autobiographical memory retrieval network in patients with left temporal lobe epilepsy. Brain. 130:2327–2342. doi: 10.1093/brain/awm166
- Addis DR, Musicaro R, Pan L, Schacter DL. 2010. Episodic simulation of past and future events in older adults: evidence from an experimental recombination task. Psychology and Aging. 25:369–376. doi: 10.1037/a0017280
- Addis DR, Pan L, Vu M-A, Laiser N, Schacter DL. 2009. Constructive episodic simulation of the future and the past: distinct subsystems of a core brain network mediate imagining and remembering. Neuropsychologia. 47:2222–2238. doi: 10.1016/j.neuropsychologia.2008.10.026
- Addis DR, Roberts RP, Schacter DL. 2011. Age-related neural changes in autobiographical remembering and imagining. Neuropsychologia. 49:3656–3669. doi: 10.1016/j.neuropsychologia.2011.09.021
- Addis DR, Schacter DL. 2008. Constructive episodic simulation: temporal distance and detail of past and future events modulate hippocampal engagement. Hippocampus. 18:227–237. doi: 10.1002/hipo.20405
- Addis DR, Schacter DL. 2012. The hippocampus and imagining the future: where do we stand? Frontiers in Human Neuroscience. 5: Article 173. doi: 10.3389/fnhum.2011.00173
- Addis DR, Wong AT, Schacter DL. 2007. Remembering the past and imagining the future: common and distinct neural substrates during event construction and elaboration. Neuropsychologia. 45:1363–1377. doi: 10.1016/j.neuropsychologia.2006.10.016
- Addis DR, Wong AT, Schacter DL. 2008. Age-related changes in the episodic simulation of future events. Psychological Science. 19:33–41. doi: 10.1111/j.1467-9280.2008.02043.x
- Adnan A, Barnett A, Moayedi M, McCormick C, Cohn M, McAndrews MP. 2016. Distinct hippocampal functional networks revealed by tractography-based parcellation. Brain Structure and Function. 221:2999–3012. doi: 10.1007/s00429-015-1084-x
- Anderson RJ, Dewhurst SA, Nash RA. 2012. Shared cognitive processes underlying past and future thinking: the impact of imagery and concurrent task demands on event specificity. Journal of Experimental Psychology: Learning, Memory, and Cognition. 38:356–365.
- Andrews-Hanna JR, Reidler JS, Sepulcre J, Poulin R, Buckner RL. 2010. Functional-anatomic fractionation of the brain’s default network. Neuron. 65:550–562. doi: 10.1016/j.neuron.2010.02.005
- Baddeley AD. 2000. The episodic buffer: a new component of working memory? Trends in Cognitive Sciences. 4:417–423. doi: 10.1016/S1364-6613(00)01538-2
- Baldassano C, Chen J, Zadbood A, Pillow JW, Hasson U, Norman KA. 2017. Discovering event structure in continuous narrative perception and memory. Neuron. 95:709–721.e5. doi: 10.1016/j.neuron.2017.06.041
- Bar M, Aminoff E. 2003. Cortical analysis of visual context. Neuron. 38:347–358. doi: 10.1016/S0896-6273(03)00167-3
- Bartlett FC. 1932. Remembering. Cambridge: Cambridge University Press.
- Beaty RE, Benedek M, Kaufman SB, Silvia PJ. 2015. Default and executive network coupling supports creative idea production. Scientific Reports. 5:10964. doi: 10.1038/srep10964
- Benoit RG, Gilbert SJ, Burgress PW. 2011. A neural mechanism mediating the impact of episodic prospection on farsighted decisions. Journal of Neuroscience. 31:6771–6779. doi: 10.1523/JNEUROSCI.6559-10.2011
- Benoit RG, Szpunar KK, Schacter DL. 2014. Ventromedial prefrontal cortex supports affective future simulation by integrating distributed knowledge. Proceedings of the National Academy of Sciences. 111:16550–16555. doi: 10.1073/pnas.1419274111
- Binder JR, Desai RH, Graves WW, Conant LL. 2009. Where is the semantic system? A critical review and meta-analysis of 120 functional neuroimaging studies. Cerebral Cortex. 19:2767–2796. doi: 10.1093/cercor/bhp055
- Bird CM, Keidel JL, Ing LP, Horner AJ, Burgess N. 2015. Consolidation of complex events via reinstatement in posterior cingulate cortex. Journal of Neuroscience. 35:14426–14434. doi: 10.1523/JNEUROSCI.1774-15.2015
- Black DL. 2000. Estimation and imagination: Western divergences from an Arabic paradigm. Topoi. 19:59–75. doi: 10.1023/A:1006399407731
- Brunet A, Orr SP, Tremblay J, Robertson K, Nader K, Pitman RK. 2008. Effect of post-retrieval propranolol on psychophysiologic responding during subsequent script-driven traumatic imagery in post-traumatic stress disorder. Journal of Psychiatric Research. 42:503–506. doi: 10.1016/j.jpsychires.2007.05.006
- Buckner RL, Carroll DC. 2007. Self-projection and the brain. Trends in Cognitive Sciences. 11:49–57. doi: 10.1016/j.tics.2006.11.004
- Cabeza R, Ciaramelli E, Moscovitch M. 2012. Cognitive contributions of the ventral parietal cortex: an integrative theoretical account. Trends in Cognitive Sciences. 16:338–352. doi: 10.1016/j.tics.2012.04.008
- Cabeza R, Moscovitch M. 2013. Memory systems, processing modes, and components: functional neuroimaging evidence. Perspectives on Psychological Science. 8:49–55. doi: 10.1177/1745691612469033
- Carpenter AC, Schacter DL. 2017. Flexible retrieval: when true inferences produce false memories. Journal of Experimental Psychology: Learning, Memory, and Cognition. 43:335–349.
- Cavanna AE, Trimble MR. 2006. The precuneus: a review of its functional anatomy and behavioural correlates. Brain. 129:564–583. doi: 10.1093/brain/awl004
- Chen J, Honey CJ, Simony E, Arcaro MJ, Norman KA, Hasson U. 2016. Accessing real-life episodic information from minutes versus hours earlier modulates hippocampal and high-order cortical dynamics. Cerebral Cortex. 26:3428–3441. doi: 10.1093/cercor/bhv155
- Collin SHP, Milivojevic B, Doeller CF. 2017. Hippocampal hierarchical networks for space, time, and memory. Current Opinion in Behavioral Sciences. 17(Supplement C):71–76. doi: 10.1016/j.cobeha.2017.06.007
- Conway MA, Pleydell-Pearce CW. 2000. The construction of autobiographical memories in the self-memory system. Psychological Review. 107:261–288. doi: 10.1037/0033-295X.107.2.261
- D’Argembeau A, Stawarczyk D, Majerus S, Collette F, Van der Linden M, Feyers D, Maquet P, Salmon E. 2010. The neural basis of personal goal processing when envisioning future events. Journal of Cognitive Neuroscience. 22:1701–1713. doi: 10.1162/jocn.2009.21314
- D’Argembeau A, van der Linden M. 2004. Phenomenal characteristics associated with projecting oneself back into the past and forward into the future: influence of valence and temporal distance. Consciousness and Cognition. 13:844–858. doi: 10.1016/j.concog.2004.07.007
- Danker JF, Anderson JR. 2010. The ghosts of brain states past: remembering reactivates the brain regions engaged during encoding. Psychological Bulletin. 136:87–102. doi: 10.1037/a0017937
- Daselaar SM, Rice HJ, Greenberg DL, Cabeza R, LaBar KS, Rubin DC. 2008. The spatiotemporal dynamics of autobiographical memory: neural correlates of recall, emotional intensity, and reliving. Cerebral Cortex. 18:217–229. doi: 10.1093/cercor/bhm048
- De Brigard F. 2014. Is memory for remembering? Recollection as a form of episodic hypothetical thinking. Synthese. 191:155–185. doi: 10.1007/s11229-013-0247-7
- De Brigard F. 2016. Memory and imagination. In: Bernecker S, Michaelian K, editor. The Routledge handbook of philosophy of memory. London: Routledge; p. 127–140.
- Devitt AL, Monk-Fromont E, Schacter DL, Addis DR. 2016. Factors that influence the generation of autobiographical memory conjunction errors. Memory. 24:204–222. doi: 10.1080/09658211.2014.998680
- Diana RA, Yonelinas AP, Ranganath C. 2007. Imaging recollection and familiarity in the medial temporal lobe: a three-component model. Trends in Cognitive Sciences. 11:379–386. doi: 10.1016/j.tics.2007.08.001
- Ebbinghaus H. 1885/1964. Ruger HA, Bussenius CE, translators. Memory: a contribution to experimental psychology. New York: Dover Publications.
- Eichenbaum H. 2001. The hippocampus and declarative memory: cognitive mechanisms and neural codes. Behavioural Brain Research. 127:199–207. doi: 10.1016/S0166-4328(01)00365-5
- Eichenbaum H. 2017. Prefrontal-hippocampal interactions in episodic memory. Nature Reviews Neuroscience. 18:547–558. doi: 10.1038/nrn.2017.74
- Ellamil M, Dobson C, Beeman M, Christoff K. 2012. Evaluative and generative modes of thought during the creative process. Neuroimage. 59:1783–1794. doi: 10.1016/j.neuroimage.2011.08.008
- Fuster JM. 1997. Network memory. Trends in Neurosciences. 20:451–459. doi: 10.1016/S0166-2236(97)01128-4
- Garry M, Manning CG, Loftus EF, Sherman SJ. 1996. Imagination inflation: imagining a childhood event inflates confidence that it occurred. Psychonomic Bulletin & Review. 3(2):208–214. doi: 10.3758/BF03212420
- Gerlach KD, Spreng RN, Gilmore AW, Schacter DL. 2011. Solving future problems: default network and executive activity associated with goal-directed mental simulations. Neuroimage. 55:1816–1824. doi: 10.1016/j.neuroimage.2011.01.030
- Gilboa A, Moscovitch M. 2017. Ventromedial prefrontal cortex generates pre-stimulus theta coherence desynchronization: a schema instantiation hypothesis. Cortex. 87:16–30. doi: 10.1016/j.cortex.2016.10.008
- Graham KS, Barense MD, Lee ACH. 2010. Going beyond LTM in the MTL: a synthesis of neuropsychological and neuroimaging findings on the role of the medial temporal lobe in memory and perception. Neuropsychologia. 48:831–853. doi: 10.1016/j.neuropsychologia.2010.01.001
- Hassabis D, Kumaran D, Vann SD, Maguire EA. 2007. Patients with hippocampal amnesia cannot imagine new experiences. Proceedings of the National Academy of Sciences of the United States of America. 104:1726–1731. doi: 10.1073/pnas.0610561104
- Hassabis D, Maguire EA. 2007. Deconstructing episodic memory with construction. Trends in Cognitive Sciences. 11:299–306. doi: 10.1016/j.tics.2007.05.001
- Hasson U, Chen J, Honey CJ. 2015. Hierarchical process memory: memory as an integral component of information processing. Trends in Cognitive Sciences. 19:304–313. doi: 10.1016/j.tics.2015.04.006
- Horner AJ, Burgess N. 2013. The associative structure of memory for multi-element events. Journal of Experimental Psychology: General. 142:1370–1383. doi: 10.1037/a0033626
- Hupbach A, Gomez R, Hardt O, Nadel L. 2007. Reconsolidation of episodic memories: a subtle reminder triggers integration of new information. Learning and Memory. 14:47–53. doi: 10.1101/lm.365707
- Ingvar DH. 1985. ‘Memory of the future’: an essay on the temporal organization of conscious awareness. Hum Neurobiol. 4:127–136.
- Irish M, Addis DR, Hodges J, Piguet O. 2012. Considering the role of semantic memory in episodic future thinking: evidence from semantic dementia. Brain. 135:2178–2191. doi: 10.1093/brain/aws119
- Irish M, Piguet O. 2013. The pivotal role of semantic memory in remembering the past and imagining the future. Frontiers in Behavioral Neuroscience. 7:27. doi: 10.3389/fnbeh.2013.00027
- Jacoby LL, Dallas M. 1981. On the relationship between autobiographical memory and perceptual learning. Journal of Experimental Psychology: General. 110(3):306–340. doi: 10.1037/0096-3445.110.3.306
- James W. 1890. The principles of psychology. New York, NY: Holt.
- Johnson MK, Hashtroudi S, Lindsay DS. 1993. Source monitoring. Psychological Bulletin. 114:3–28. doi: 10.1037/0033-2909.114.1.3
- Kahn I, Andrews-Hanna JR, Vincent JL, Snyder AZ, Buckner RL. 2008. Distinct cortical anatomy linked to subregions of the medial temporal lobe revealed by intrinsic functional connectivity. Journal of Neurophysiology. 100:129–139. doi: 10.1152/jn.00077.2008
- Klein SB. 2013. The temporal orientation of memory: it’s time for a change of direction. Journal of Applied Research in Memory and Cognition. 2:222–234. doi: 10.1016/j.jarmac.2013.08.001
- Kroes MC, Fernández G. 2012. Dynamic neural systems enable adaptive, flexible memories. Neuroscience and Biobehavioral Reviews. 36:1646–1666. doi: 10.1016/j.neubiorev.2012.02.014
- Levine B, Svoboda E, Hay JF, Winocur G, Moscovitch M. 2002. Aging and autobiographical memory: dissociating episodic from semantic retrieval. Psychology and Aging. 17:677–689. doi: 10.1037/0882-7974.17.4.677
- Locke J. 1694/1970. An essay concerning human understanding, 2nd ed. Yorkshire, UK: The Scholar Press.
- Loftus EF. 2005. Planting misinformation in the human mind: a 30-year investigation of the malleability of memory. Learning & Memory. 12:361–366. doi: 10.1101/lm.94705
- Mack ML, Love BC, Preston AR. 2016. Dynamic updating of hippocampal object representations reflects new conceptual knowledge. Proceedings of the National Academy of Sciences of the United States of America. 113:13203–13208. doi: 10.1073/pnas.1614048113
- Madore KP, Preston PT, Beaty RE, Addis DR, Schacter DL. 2017. Neural mechanisms of episodic retrieval support divergent creative thinking. Cerebral Cortex. In press.
- Maguire EA. 2001. Neuroimaging studies of autobiographical event memory. Philosophical Transactions of the Royal Society B: Biological Sciences. 356:1441–1451. doi: 10.1098/rstb.2001.0944
- Mahr J, Csibra G. 2017. Why do we remember? The communicative function of episodic memory. Behavioral and Brain Sciences. 41:E1. https://www.cambridge.org/core/journals/behavioral-and-brain-sciences/article/why-do-we-remember-the-communicative-function-of-episodic-memory/CC092B430A7213B4C9BF2C4B48415B64.
- Mar RA. 2004. The neuropsychology of narrative: story comprehension, story production and their interrelation. Neuropsychologia. 42:1414–1434. doi: 10.1016/j.neuropsychologia.2003.12.016
- Martin VC, Schacter DL, Corballis MC, Addis DR. 2011. A role for the hippocampus in encoding simulations of future events. Proceedings of the National Academy of Sciences of the United States of America. 108:13858–13863. doi: 10.1073/pnas.1105816108
- McClelland JL, McNaughton BL, O'Reilly RC. 1995. Why there are complementary learning systems in the hippocampus and neocortex: insights from the successes and failures of connectionist models of learning and memory. Psychological Review. 102:419–457. doi: 10.1037/0033-295X.102.3.419
- McCormick C, Moscovitch M, Valiante TA, Cohn M, McAndrews MP. 2018. Different neural routes to autobiographical memory recall in healthy people and individuals with left medial temporal lobe epilepsy. Neuropsychologia. 110:26–36. doi: 10.1016/j.neuropsychologia.2017.08.014
- McCormick C, St-Laurent M, Ty A, Valiante TA, McAndrews MP. 2015. Functional and effective hippocampal-neocortical connectivity during construction and elaboration of autobiographical memory retrieval. Cerebral Cortex. 25:1297–1305. doi: 10.1093/cercor/bht324
- Michaelian K. 2011. Generative memory. Philosophical Psychology. 24:323–342. doi: 10.1080/09515089.2011.559623
- Mitchell KJ, Johnson MK. 2009. Source monitoring 15 years later: what have we learned from fMRI about the neural mechanisms of source memory? Psychological Bulletin. 135(4):638–677. doi: 10.1037/a0015849
- Morton NW, Sherrill KR, Preston AR. 2017. Memory integration constructs maps of space, time, and concepts. Current Opinion in Behavioral Sciences. 17:161–168. doi: 10.1016/j.cobeha.2017.08.007
- Nadel L, Moscovitch M. 1998. Hippocampal contributions to cortical plasticity. Neuropharmacology. 37(4-5):431–439. doi: 10.1016/S0028-3908(98)00057-4
- O’Callaghan J. 2016. Thomas Aquinas. In: Bernecker S, Michaelian K, editor. The Routledge handbook of philosophy of memory. London: Routledge; p. 461–479.
- Okuda J, Fujii T, Ohtake H, Tsukiura T, Tanji K, Suzuki K, Kawashima R, Fukuda H, Itoh M, Yamadori A. 2003. Thinking of the future and past: the roles of the frontal pole and the medial temporal lobes. Neuroimage. 19:1369–1380. doi: 10.1016/S1053-8119(03)00179-4
- Place R, Farovik A, Brockmann M, Eichenbaum H. 2016. Bidirectional prefrontal-hippocampal interactions support context-guided memory. Nature Neuroscience. 19:992–994. doi: 10.1038/nn.4327
- Roberts RP, Schacter DL, Addis, DR. 2017. Scene construction and relational processing: separable constructs? Cerebral Cortex. In press.
- Roberts RP, Wiebels K, Sumner RL, van Mulukom V, Grady CL, Schacter DL, Addis DR. 2017. An fMRI investigation of the relationship between future imagination and cognitive flexibility. Neuropsychologia. 95:156–172. doi: 10.1016/j.neuropsychologia.2016.11.019
- Robin J, Moscovitch M. 2017. Details, gist and schema: hippocampal–neocortical interactions underlying recent and remote episodic and spatial memory. Current Opinion in Behavioral Sciences. 17:114–123. doi: 10.1016/j.cobeha.2017.07.016
- Ross M, Wilson AE. 2003. Autobiographical memory and conceptions of self: getting better all the time. Current Directions in Psychological Science. 12:66–69. doi: 10.1111/1467-8721.01228
- Rubin DC, Umanath S. 2015. Event memory: a theory of memory for laboratory, autobiographical, and fictional events. Psychological Review. 122:1–23. doi: 10.1037/a0037907
- Rugg MD, Vilberg KL. 2013. Brain networks underlying episodic memory retrieval. Current Opinion in Neurobiology. 23:255–260. doi: 10.1016/j.conb.2012.11.005
- Russell B. 1921. The analysis of mind. London: George Allen and Unwin.
- Schacter DL, Addis DR. 2007. The cognitive neuroscience of constructive memory: remembering the past and imagining the future. Philosophical Transactions of the Royal Society B: Biological Sciences. 362:773–786. doi: 10.1098/rstb.2007.2087
- Schacter DL, Addis DR, Buckner RL. 2007. Remembering the past to imagine the future: the prospective brain. Nature Reviews Neuroscience. 8:657–661. doi: 10.1038/nrn2213
- Schacter DL, Addis DR, Hassabis D, Martin VC, Spreng RN, Szpunar KK. 2012. The future of memory: remembering, imagining, and the brain. Neuron. 76:677–694. doi: 10.1016/j.neuron.2012.11.001
- Schacter DL, Carpenter AC, Devitt AL, Roberts RP, Addis DR. 2018. Constructive episodic simulation, flexible recombination, and memory errors. Behavioral and Brain Sciences. 41:335. doi: 10.1017/S0140525X17001510
- Schacter DL, Madore KP. 2016. Remembering the past and imagining the future: identifying and enhancing the contribution of episodic memory. Memory Studies. 9:245–255. doi: 10.1177/1750698016645230
- Schacter DL, Norman KA, Koutstaal W. 1998. The cognitive neuroscience of constructive memory. Annual Review of Psychology. 49:289–318. doi: 10.1146/annurev.psych.49.1.289
- Schlichting ML, Preston AR. 2016. Hippocampal-medial prefrontal circuit supports memory updating during learning and post-encoding rest. Neurobiology of Learning and Memory. 134:91–106. doi: 10.1016/j.nlm.2015.11.005
- Sheldon S, Levine B. 2016. The role of the hippocampus in memory and mental construction. Annals of the New York Academy of Sciences. 1369:76–92. doi: 10.1111/nyas.13006
- Sheldon S, McAndrews MP, Moscovitch M. 2011. Episodic memory processes mediated by the medial temporal lobes contribute to open-ended problem solving. Neuropsychologia. 49:2439–2447. doi: 10.1016/j.neuropsychologia.2011.04.021
- Spreng RN. 2012. The fallacy of a “task-negative” network. Frontiers in Psychology. 3:145. doi: 10.3389/fpsyg.2012.00145
- Spreng RN, Grady CL. 2010. Patterns of brain activity supporting autobiographical memory, prospection, and theory-of-mind and their relationship to the default mode network. Journal of Cognitive Neuroscience. 22:1112–1123. doi: 10.1162/jocn.2009.21282
- Spreng RN, Stevens WD, Chamberlain JP, Gilmore AW, Schacter DL. 2010. Default network activity, coupled with the frontoparietal control network, supports goal-directed cognition. Neuroimage. 53:303–317. doi: 10.1016/j.neuroimage.2010.06.016
- Squire LR, van der Horst AS, McDuff SGR, Frascino JC, Hopkinse RO, Mauldin KN. 2010. Role of the hippocampus in remembering the past and imagining the future. Proceedings of the National Academy of Sciences of the United States of America. 107:19044–19048. doi: 10.1073/pnas.1014391107
- St-Laurent M, Abdi H, Buchsbaum BR. 2015. Distributed patterns of reactivation predict vividness of recollection. Journal of Cognitive Neuroscience. 27:2000–2018. doi: 10.1162/jocn_a_00839
- Summerfield JJ, Hassabis D, Maguire EA. 2010. Differential engagement of brain regions within a ‘core’ network during scene construction. Neuropsychologia. 48:1501–1509. doi: 10.1016/j.neuropsychologia.2010.01.022
- Szpunar KK, Chan JCK, McDermott KB. 2009. Contextual processing in episodic future thought. Cerebral Cortex. 19:1539–1548. doi: 10.1093/cercor/bhn191
- Szpunar KK, Schacter DL. 2013. Get real: effects of repeated simulation and emotion on the perceived plausibility of future experiences. Journal of Experimental Psychology: General. 142:323–327. doi: 10.1037/a0028877
- Szpunar KK, Spreng RN, Schacter DL. 2014. A taxonomy of prospection: introducing an organizational framework for future-oriented cognition. Proc Natl Acad Sci USA. 111:18414–18421. doi: 10.1073/pnas.1417144111
- Szpunar KK, Watson JM, McDermott KB. 2007. Neural substrates of envisioning the future. Proceedings of the National Academy of Sciences of the United States of America. 104:642–647. doi: 10.1073/pnas.0610082104
- Taylor SE, Schneider SK. 1989. Coping and the simulation of events. Social Cognition. 7:174–194. doi: 10.1521/soco.1989.7.2.174
- Thakral PP, Benoit RG, Schacter DL. 2017. Imagining the future: the core episodic simulation network dissociates as a function of timecourse and the amount of simulated information. Cortex. 90:12–30. doi: 10.1016/j.cortex.2017.02.005
- Thakral PP, Madore KP, Schacter DL. 2017. A role for the left angular gyrus in episodic simulation and memory. The Journal of Neuroscience. 37:8142–8149. doi: 10.1523/JNEUROSCI.1319-17.2017
- Tulving E. 1972. Episodic and semantic memory. In: Tulving E, Donaldson W, editor. Organization of memory. New York, NY: Academic Press; p. 381–403.
- Tulving E. 1985. Memory and consciousness. Canadian Psychologist. 25:1–12.
- Urmson JO. 1967. VII.—Memory and imagination. Mind. LXXVI(301):83–91. doi: 10.1093/mind/LXXVI.301.83
- van Mulukom V. 2013. Imagining a brave new future: the effects of novelty and plausibility on episodic simulation. Auckland: The University of Auckland.
- van Mulukom V, Schacter DL, Corballis MC, Addis DR. 2013. Re-imagining the future: repetition decreases hippocampal involvement in future simulation. PLoS ONE. 8:e69596. doi: 10.1371/journal.pone.0069596
- Wade KA, Garry M, Read JD, Lindsay DS. 2002. A picture is worth a thousand lies: using false photographs to create false childhood memories. Psychonomic Bulletin & Review. 9(3):597–603. doi: 10.3758/BF03196318
- Weiler JA, Suchan B, Daum I. 2010. Foreseeing the future: occurrence probability of imagined future events modulates hippocampal activation. Hippocampus. 20:685–690.
- Woodruff CC, Johnson JD, Uncapher MR, Rugg MD. 2005. Content-specificity of the neural correlates of recollection. Neuropsychologia. 43:1022–1032. doi: 10.1016/j.neuropsychologia.2004.10.013
- Zacks JM, Speer NK, Swallow KM, Braver TS, Reynolds JR. 2007. Event perception: a mind-brain perspective. Psychological Bulletin. 133:273–293. doi: 10.1037/0033-2909.133.2.273
- Zeidman P, Mullally SL, Maguire EA. 2015. Constructing, perceiving, and maintaining scenes: hippocampal activity and connectivity. Cerebral Cortex. 25:3836–3855. doi: 10.1093/cercor/bhu266