ABSTRACT
Epichloë fungal endophytes that systemically colonise cool-season grasses have become an internationally well-researched symbiosis. While sexual species of Epichloë can cause choke disease of grass seed heads, asexual forms are symptomless endophytes that colonise embryos to become seed transmitted. In mutualistic associations, the grass benefits through greater tolerance of abiotic and biotic stresses. In New Zealand, at least 12 species of naturalised and native grasses have been documented as infected with a range of asexual or sexual Epichloë. The impact of endophyte-infected ryegrass and tall fescue has been significant for pastoral farmers, with Epichloë enhancing pasture performance. Production of bioactive secondary metabolites reduces herbivory by invertebrate pests but some are also toxic to grazing animals. Selected strains of Epichloë have been utilised to reduce livestock problems while retaining improved pasture performance. Documented Epichloë-infected native grasses are rare, justifying greater efforts of discovery, description and conservation as taonga (a treasure) for Māori and all New Zealanders. Given their ecological success in New Zealand ecosystems, along with economic and environmental benefits, there are good prospects to further develop both endemic and naturalised Epichloë-grass associations that may benefit other cool-season grasses and potentially deliver new beneficial properties.
Introduction
Grasslands are an important component of the New Zealand landscape, occupying over half the land area. Native tussock grasslands dominate the montane regions of both the North and South Islands, while introduced grasslands dominate our farms and contribute to urban landscapes. Large areas of introduced grasslands underpin the New Zealand economy through a NZ$20 billion annual export industry of pasture-fed meat, milk and fibre products with ryegrass (Lolium spp.) being the most important plant species in the New Zealand economy (Ministry for Primary Industries Citation2016).
Grasslands are composed of a diverse range of grasses, legumes and herbs, with grasses contributing the greatest biomass. With over 12,000 species of grass (Family Poaceae) described globally, considerable genetic diversity enables grasses to colonise numerous ecological habitats across the world (Soreng et al. Citation2015). New Zealand has only a fraction of these with over 400 species, comprising approximately similar numbers of native and introduced taxa () (Edgar and Connor Citation2000). Of these 400+ species, over 60% are cool-season grasses (subfamily Pooideae). Within this subfamily, seven of the 14 tribes have grass species that are known globally to host fungal endophytes from the genus Epichloë (Schardl Citation2010; Tadych et al. Citation2014).
Figure 1. Breakdown of New Zealand’s grass flora (family Poaceae) into native and naturalised species; subfamilies (Pooideae vs. non-Pooideae); and Pooideae tribes known globally to host species infected with Epichloë fungal endophytes. Values in parentheses are approximate percentages of total grass species in New Zealand. Based on Edgar and Connor (Citation2000).
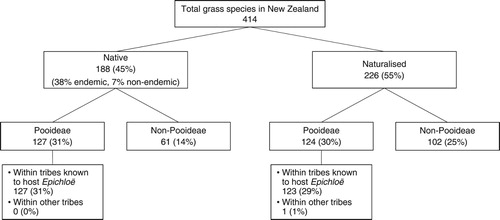
Epichloë form symbiotic associations with their grass host, which range from antagonistic to mutualistic. These Epichloë-grass associations have been widely studied particularly where Epichloë enhance the ability of the grass host to resist a range of abiotic and biotic stresses, and where Epichloë may negatively impact livestock production. This is of prime economic importance in the exotic grasslands of a number of regions, notably the New World countries of New Zealand, Australia and USA (Hume et al. Citation2016; Johnson and Caradus Citation2019).
This review is the first to consider Epichloë endophytes in both native and introduced grasses of New Zealand. Epichloë-grass associations are described within a New Zealand context of discovery, conservation, utilisation in pastoral agriculture and future opportunities to capture value from these unique fungal-grass symbioses.
Biology of Epichloë
Phylogenetic evidence points to a distant origin of Epichloë in fungal pathogens of insects, with interkingdom host jumps believed to have occurred including one to plants such that the fungi have become associated with grasses rather than insects (Spatafora et al. Citation2007). Following the host jump to grasses the fungus has co-evolved with its host (Schardl et al. Citation2008) and there is evidence, in the genome of Epichloë, of genes for host specialisation (Schirrmann et al. Citation2018). Epichloë comprise both sexual and asexual (formerly Neotyphodium) species (Leuchtmann et al. Citation2014). For asexual species, many of which are allopolyploid interspecific hybrids (Leuchtmann et al. Citation2014; Tadych et al. Citation2014), colonisation of the host embryo leads to vertical transmission through the host seed and generally mutualistic interactions (Schardl Citation2010). Some sexual species can also be transmitted vertically but more typically are transmitted horizontally through infectious ascospores and cause choke disease (Schardl Citation2010). Choke disease is so called as the endophytic fungus grows externally, forming a dense hyphal mass or stroma that envelops, or chokes, the incipient inflorescence. Conidia (mitotic spores) are produced on the stroma and when these are transferred, usually by an invertebrate vector, stroma can produce infective meiotic spores (ascospores) that are capable of pathogenically infecting other grass host individuals thus effecting a horizontal transfer. In mutualistic interactions the endophyte confers biotic and abiotic advantages to the host (Malinowski and Belesky Citation2000; Hume et al. Citation2016), many of which are mediated through the production of in planta produced secondary metabolites such as the loline, indole-diterpene, peramine and ergot alkaloids (Schardl et al. Citation2013b).
During colonisation of grass leaves, Epichloë grows asymptomatically within the intercellular spaces between plant cells in a highly synchronised and tightly regulated manner (Christensen et al. Citation2008). Hyphae undergo apical extension in the shoot apical meristem but, as the host cells expand, switch to intercalary hyphal growth along the length of the hypha. Hyphal growth ceases when the plant stops growing but the endophyte remains metabolically active (Tan et al. Citation2001). Loss of this tight regulation of fungal growth has been shown to cause a switch from a mutualistic to pathogenic lifestyle including the extensive colonisation of vascular bundles, stunting of the host plants and even death (Eaton et al. Citation2011). A core set of genes underpinning this symbiosis have been identified (Eaton et al. Citation2011), with examples including genes involved in iron homeostasis (Johnson et al. Citation2013b), generation of reactive oxygen species (Scott et al. Citation2007), and small secreted proteins (Hassing et al. Citation2019).
Native grasses
Of the few studies that have examined New Zealand and Australian native grasses for the presence of Epichloë endophytes, results to date suggest that endophyte infection is rare in the antipodes (Leuchtmann et al. Citation2019). This contrasts with these endophytes infecting a wide range of grasses native to the Northern Hemisphere despite the same grass genera occurring in both hemispheres, and points to a Northern Hemisphere co-evolutionary origin of Epichloë and associated grass species and their dispersal east and south (Inda et al. Citation2008; Schardl Citation2010).
New Zealand has 188 native species of grass, of which the majority (157) are endemic with the remaining species also found in Australia and neighbouring areas of the Pacific (Edgar and Connor Citation2000) (). To date, surveys have examined only 36% of these New Zealand grass species and seldom more than a few populations of each, resulting in just three species (4% of native grasses surveyed) that are Epichloë infected (Miles et al. Citation1998; Davis and Guy Citation2001; Rolston et al. Citation2002; Stewart et al. Citation2004; Leuchtmann et al. Citation2019). The situation is similar in Australia with Aldous et al. (Citation1999) reporting that Epichloë were absent in native grasses of at least 19 species from 13 genera, with the only report of Epichloë being for Echinopogon spp. (Miles et al. Citation1998). These endophyte-infected grasses are species of the grass tribe Poeae within the subfamily Pooideae, a tribe known to host Epichloë (Schardl Citation2010).
Endophyte-infected grasses
The first report of a native grass infected with Epichloë in New Zealand was Echinopogon ovatus (hedgehog grass) () (Miles et al. Citation1998). This grass is the only Echinopogon species in New Zealand and is a species shared with Australia, New Guinea and Indonesia. Ec. ovatus occurs throughout New Zealand with Epichloë-infected plants found widely in the North Island (Miles et al. Citation1998; Moon et al. Citation2002) and at two locations in the north and east of the South Island (Leuchtmann et al. Citation2019) (A.V. Stewart and D.E. Hume, unpublished data). In central regions of the North Island, Miles et al. (Citation1998) recorded a high (90%) average rate of infection from fresh samples. Miles et al. (Citation1998) also examined samples of Echinopogon from sites in New South Wales, Australia. As was found in New Zealand, infection rate was high (87%) in fresh samples of Ec. ovatus and from examination of herbarium samples a further four out of seven Echinopogon species were found to be colonised by Epichloë.
Figure 2. Echinopogon ovatus (hedgehog grass) – the first New Zealand native grass to be identified as a host of Epichloë.
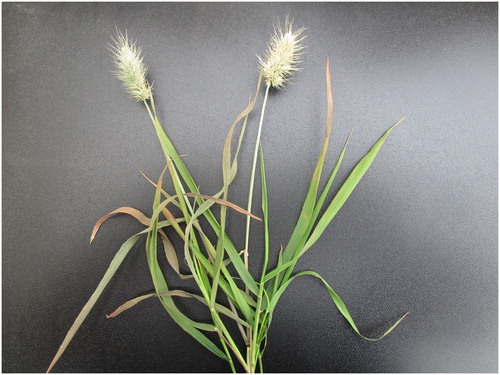
The native grass most intensely surveyed in New Zealand for Epichloë is Poa matthewsii (Matthew’s bluegrass), an endemic grass found in both the North and South Islands. Endophyte-infected populations have been identified (infection rate in parentheses) in the lower North Island (86-100%) and the eastern South Island on Banks Peninsula (60%) and Otago Peninsula (10%) (Leuchtmann et al. Citation2019). There is also evidence of genetic diversity (using simple sequence repeat markers) among Epichloë isolates from these grass populations, as is seen in other asexual species of Epichloë (Kaur et al. Citation2015).
Epichloë-infected Dichelachne micrantha (short-hair plume grass) is the most recently discovered native grass-Epichloë association in New Zealand (Leuchtmann et al. Citation2019). This grass is present throughout Northland, usually in areas close to the coast. Just one population from Hokianga has been examined and with over 80% infection it is likely that other infected populations will be identified. Further work is required to determine if the other three New Zealand native and two naturalised Dichelachne species host Epichloë. While D. micrantha is also found in Australia and some islands of the South Pacific, populations in these locations have not been examined for endophyte (Aldous et al. Citation1999). Assuming it follows a similar pattern to Ec. ovatus, which is endophyte-infected in both New Zealand and Australia (Miles et al. Citation1998), D. micrantha and other species of this genus should be a focus for further surveys of Epichloë in Australia.
Species of endophyte
These three endophyte-infected native grasses (Ec. ovatus, P. matthewsii, D. micrantha) are colonised by three species of asexual Epichloë, and as indicated by the assigned species names, they are unique to New Zealand and Australia () (Moon et al. Citation2002; Leuchtmann et al. Citation2019). E. australiensis is found in both countries and in different host grasses. E. aotearoae colonises one species of grass found in both countries, while E. novae-zelandiae is only found in New Zealand colonising an endemic grass. Through use of molecular phylogenetic methods, their ancestry has been determined (), and along with host evolution and range, theories of evolution and spread have been developed for these and other Epichloë-grass associations (Moon et al. Citation2002; Leuchtmann et al. Citation2019).
Table 1. Native grasses infected with asexual Epichloë endophytes in New Zealand and Australia (Miles et al. Citation1998; Moon et al. Citation2002; Leuchtmann et al. Citation2019).
Two of these native Epichloë are allopolyploid interspecific hybrids (Schardl et al. Citation1994) with two or three parental sexual ancestors. Interspecific hybrid Epichloë are common, contributing to approximately half of the Epichloë described to-date, with the vast majority of these hybrids being asexual (Leuchtmann et al. Citation2014; Tadych et al. Citation2014). Hybridisation is recognised as an important mechanism by which genetic variation can be generated to overcome the lack of genetic recombination in asexual Epichloë and a process by which the genes required for sexual reproduction may be lost (Schardl Citation2010; Tadych et al. Citation2014). Given the prevalence of Epichloë in the northern hemisphere, it is unsurprising that the lineages of these Southern Hemisphere hybrid endophytes are closely aligned with those of the Northern Hemisphere (Moon et al. Citation2002). The exception is E. aotearoae which is a non-hybrid that appears to have developed early in the evolution of Epichloë and can now be found in association with E. ovatus in New Zealand and Australia, and is a component of hybrid endophytes of the Southern Hemisphere (Moon et al. Citation2002).
Drivers for endophyte infection
While the number of species of native grasses infected with Epichloë in the New Zealand (and Australian) landscape is low, the three New Zealand grass species that have been identified as hosting Epichloë are infected at high rates. This suggests that infection with these Epichloë species in their respective host grasses is imparting a selective advantage to infected plants. Invertebrate pests have been the major selective pressure in New Zealand’s cultivated exotic pastures, with Epichloë endophytes able to reduce damage from a range of exotic and native invertebrates (Popay and Hume Citation2011). Evidence for a similar effect has been demonstrated for Epichloë-infected Ec. ovatus and P. matthewsii for Argentine stem weevil (Listronotus bonariensis) (Miles et al. Citation1998; Leuchtmann et al. Citation2019). This is supported through the presence of biosynthetic pathway genes, and expression, of secondary metabolites that are known for their pesticidal properties (Miles et al. Citation1998; Leuchtmann et al. Citation2019).
While these endophytes may provide a selective advantage to these grasses in the context of today’s modified New Zealand ecosystems with its many exotic pests such as Argentine stem weevil, these Epichloë must have imparted an ecological advantage to these native grasses well before humans arrived. Further testing with invertebrates that are native to New Zealand may elucidate the role of pest protection in the evolution of these grass-endophyte associations prior to human habitation. While protection from invertebrate pests may be a prime focus, multiple mechanisms may have favoured endophyte-infected grass plants in New Zealand as Epichloë are known to reduce stresses on grasses from a range of biotic (e.g. fungal disease) and abiotic (e.g. soil water deficit, shade) factors (Malinowski and Belesky Citation2000; Lewis 2004; Hume et al. Citation2016).
Value to New Zealand
The presence and evolutionary origins of Epichloë in New Zealand native grasses is of considerable biological interest, however endeavours to pursue a greater understanding have largely been driven by ecological curiosity and with little funding. Apart from the extensively farmed native tussock grasslands of high country regions of New Zealand (dominated by apparently endophyte-free grass species of Chionochloa, Poa and Festuca), native grasses play no role in New Zealand’s pastoral systems in hill country and lowlands, and just a minor role in landscaping and gardens (Stewart Citation2005a). In addition, there is an absence of reported Epichloë-driven toxicity in farmed animals grazing native grasses despite the possibility of species such as endophyte-infected Ec. ovatus causing mammalian tremors as has been reported in Australia (Miles et al. Citation1998) and the potential for toxicosis based on endophyte alkaloid profiles (Miles et al. Citation1998; Leuchtmann et al. Citation2019). This contrasts with the major advances in understanding Epichloë-grass associations in the agriculturally important, intensively farmed, temperate grasslands of New Zealand, Australia and the USA that occurred once Epichloë endophytes were linked with animal toxicity and plant performance (Hume et al. Citation2016).
The conservation status of New Zealand native plants is declining, which includes many native grasses (de Lange et al. Citation2009). Although commercialisation of native grasses could offer a means to enhance the conservation status of both native grasses and their endophytes, there is currently no commercial seed of any native grass available and prospects for commercial development appear very limited (Stewart Citation2005a). However, there is an opportunity and imperative for agricultural landscapes to at least preserve and enhance native areas that will maintain these unique symbioses in-situ along with other native flora and fauna. This will broaden the base of New Zealand’s biodiversity beyond the designated conservation estate therefore strengthening conservation efforts. In addition, as Epichloë is seed transmitted, collection of seed can be used to preserve both the fungal and plant germplasm ex-situ under well-controlled storage conditions such as the Margot Forde Germplasm Centre at Palmerston North (DOC Citation2000). This repository also provides a resource for use in further characterisation of these symbiotic associations.
These native Epichloë-grass associations contribute to the wealth of native species, many of which are endemic, that have evolved since New Zealand separated from Australia 85 million years ago. As native species, Epichloë-infected grasses represent a taonga (treasure) for Māori and all New Zealanders. In particular, the association of E. novae-zelandiae with P. matthewsii is endemic to New Zealand. While this will drive the need for in-situ and ex-situ conservation, the uniqueness of these Epichloë-grass associations to New Zealand (and Australia) is an opportunity to discover new properties not present in associations elsewhere in the world. These could then be harnessed directly (e.g. new bioactives) and/ or indirectly to benefit our production systems (e.g. transfer of Epichloë to the current, agriculturally important grasses). All stages in such a process would need to acknowledge the tangata whenua (people of the land) as kaitiaki (guardians) and protect and incorporate mātauranga Māori (Māori knowledge) and tikanga-ā-iwi (customary use) (DOC Citation2000).
Naturalised grasses
From the early 1800s onwards, European settlers both deliberately and accidentally introduced exotic grassland species to New Zealand, predominately grasses from Europe (Langer Citation1990). These formed the basis of pastoral agriculture in New Zealand. Exotic grasses that naturalised in New Zealand (226 species) now contribute over half the total grass flora in this country (Edgar and Connor Citation2000) and approximately half of these naturalised grasses are within the cool-season grasses subfamily Pooideae (Edgar and Connor Citation2000) (). Within this subfamily, six of the seven tribes that are known globally to harbour Epichloë are naturalised in New Zealand (). It is therefore not unexpected that naturalised grasses in New Zealand are infected with Epichloë but those identified to date are confined to just a few grass species of Lolium and Festuca within the tribe Poeae (). These include both asexual and sexual forms of Epichloë.
Table 2. Tribes of subfamily Pooideae (cool-season grasses), showing those known to be infected with Epichloë, the number of species of these tribes naturalised in New Zealand and those infected with Epichloë.
The Epichloë-grass associations most studied and understood are those of importance to pastoral agriculture, particularly where cultivars are developed and sold widely in commerce for use in pastures and for some species as turf. These are primarily species of ryegrass (Lolium perenne, L. boucheanum syn. L. hybridum, and L. multiflorum), to a much lesser extent tall fescue (Festuca arundinacea) and a very minor component of meadow fescue (F. pratensis). In most cases, Epichloë colonising these grasses are significant drivers of animal productivity and welfare, and pasture performance (Johnson et al. Citation2013a). In addition, as these grasses are colonised by asexual endophytes, industry can obtain intellectual property protection which empowers commercial development and delivery of endophytic grass products. These provide important economic benefits to farmers and the New Zealand economy (Johnson and Caradus Citation2019) along with delivering improved environmental outcomes through greater pasture persistence, productivity and quality.
Perennial ryegrass
In 1940, perennial ryegrass (L. perenne) and ‘long-term’ perennial x Italian ryegrass hybrids (L. boucheanum) were the first grasses in New Zealand to be identified as infected with Epichloë (Neill Citation1940). Epichloë were intensively surveyed and tested at this time and in the following decades, as researchers investigated if fungal toxins were causing a range of serious ill-health effects in grazing livestock including facial eczema, ryegrass staggers and lameness. In various controlled studies, Epichloë of ryegrass (and tall fescue) was found to be non-toxic to rodents, birds and sheep, have no influence on grazing preference, and not affect plant growth (Neill Citation1941, Citation1952; Cunningham Citation1958). Professional opinion was that ‘a widely distributed fungus such as Lolium endophyte could not be responsible for localized outbreaks of ryegrass staggers’ (Cunningham and Hartley Citation1959). It was not until late in the twentieth century that Epichloë was conclusively linked with ryegrass staggers (Fletcher and Harvey Citation1981) along with a range of other detrimental effects on livestock (di Menna et al. Citation2012), and it was found that Epichloë could also greatly boost the agronomic performance of ryegrass as it provided protection from Argentine stem weevil (Mortimer et al. Citation1982) and other insects (Popay and Hume Citation2011). A similar situation became evident for large areas of ryegrass in south-eastern Australia (Young et al. Citation2013; Hume and Sewell Citation2014; Hume et al. Citation2016).
These findings were of considerable importance for New Zealand agriculture as perennial and long-term hybrid ryegrasses were, and remain, the most widely sown pasture and turf grasses in New Zealand (Langer Citation1990; Stewart et al. Citation2014). Under conditions of high soil fertility and intensive grazing, ryegrass is valued globally for its ease of establishment and subsequent management in pastures and turf, and in pastoral farming for the production of high quality forage in most seasons of the year (Jung et al. Citation1996). In New Zealand, selective pressures of insect predation combined with periodic drought and intensive grazing systems, have strongly favoured Epichloë-infected plants, resulting in high frequencies of Epichloë infection in naturalised ryegrass, old pastures and turf and locally bred cultivars (Stewart Citation2005b; Thom et al. Citation2012).
To address the dilemma of Epichloë being needed to ensure the good agronomic performance of pastures, but with the downside of losses in animal production, researchers searched for variation in endophyte strains. Strains present in New Zealand were genetically very similar (Simpson et al. Citation2012) and had no useful diversity in the production of the endophyte alkaloids that drove pest resistance and animal ill-health (Latch and Tapper Citation1988). However, diversity of Epichloë was abundant in the European, Eurasian and Mediterranean Basin centres of origin of ryegrass (and tall fescue) which led to the development of ‘selected Epichloë endophytes’ (Johnson and Caradus Citation2019). Selected strains offered good protection to the host ryegrass from a range of invertebrate pests, and no or greatly reduced toxicity to livestock. These endophytes were rapidly incorporated into elite New Zealand-adapted cultivars by plant breeders, and the seed industry produced sufficient quantities and quality of Epichloë-infected seed to enable widespread adoption by pastoral farmers. Today an estimated 90% of proprietary perennial ryegrass seed sold is infected with a selected Epichloë endophyte, with AR1 and AR37 being the predominate strains (Caradus et al. Citation2013); (J.R. Caradus, pers. comm.). The same approach has also been successful in addressing a similar dilemma for Epichloë-infected tall fescue.
Annual ryegrass
Of the seven species of annual ryegrass known globally to host the asexual hybrid E. occultans (Moon et al. Citation2000; Schardl Citation2010; Tadych et al. Citation2014), only L. multiflorum, L. rigidum and L. temulentum are naturalised in New Zealand (Edgar and Connor Citation2000). L. multiflorum and its hybrids are widely infected with Epichloë in New Zealand. For plants of New Zealand origin, Neill (Citation1941) and Cunningham (Citation1958) reported Epichloë-infected L. temulentum, while there have been no reports of Epichloë infecting the less common L. rigidum.
Associations of E. occultans with L. multiflorum (commonly referred to as Italian ryegrass and annual ryegrass) and the ‘short-term’ Italian x perennial hybrids (L. boucheanum) have been the most closely studied annual ryegrasses as these species are widely naturalised and are important short-lived grasses of intensive pastoral farming in New Zealand and globally (Jung et al. Citation1996; Stewart et al. Citation2014). In New Zealand, E. occultans infection levels are generally high in ecotypes but variable in commercial cultivars (Forde et al. Citation1988; Latch et al. Citation1988). The variable infection levels in cultivars is likely to arise from little active selection for Epichloë infection by plant breeders, along with practices that fail to maintain viable endophyte during commercial seed multiplication, storage and distribution (Stewart Citation1989; Johnson and Caradus Citation2019).
The high prevalence of Epichloë in L. multiflorum/L. boucheanum ecotypes suggests endophyte infection provides a selective advantage over endophyte-free plants, however the mechanisms involved are less certain than they are for Epichloë-L. perenne associations in New Zealand. In particular, herbage endophyte alkaloid concentrations are very low in E. occultans-ryegrass associations and do not provide effective protection from foliar feeding pest insects such as Argentine stem weevil, except possibly at the early stages of seedling establishment (Prestidge Citation1991). The low alkaloid concentrations can mostly be attributed to E. occultans colonising only the very base of the leaf sheath (1–2 mm zone above the node) in contrast to extensive colonisation of the leaf exhibited by Epichloë festucae var. lolii in L. perenne (Latch et al. Citation1988; Faville et al. Citation2015). Despite these low alkaloid concentrations, protection from other insects may occur in mature plants (Cooper et al. Citation2007). Epichloë may also protect the seed prior to germination through moderate concentrations of endophyte alkaloids such as peramine and lolines in seed (Sugawara et al. Citation2006) (D. E. Hume unpublished data).
Given E. occultans-infected ryegrasses are free of mammalian toxic ergovaline and lolitrem B (Sugawara et al. Citation2006; Schardl et al. Citation2013b), or toxins are at very low concentrations (Prestidge Citation1991), there is the opportunity to utilise E. occultans to improve agronomic performance of L. multiflorum/L. boucheanum pastures in New Zealand.
Tall fescue
Tall fescue can be found naturalised throughout the country, particularly along roadsides, unmanaged waste areas, poorly drained areas, and neighbouring drains and other waterways. Cultivars are utilised by pastoral farmers and as a turf grass, with Epichloë endophytes found in both naturalised populations and managed cultivars (Neill Citation1941; Guy and Davis Citation2002; Young et al. Citation2013). New Zealand and Australia have similar and somewhat checkered histories in the use and development of tall fescue and its associated Epichloë endophyte (Easton et al. Citation1994; Hume and Sewell Citation2014).
Tall fescue became known for its toxicity to livestock in New Zealand from the early 1900s (Anonymous Citation1918; Cunningham Citation1948) and in Australia from at least the mid-1900s (Pulsford Citation1950). This toxicity was most prominent in cattle and is now known to be due to toxic strains of Epichloë, mirroring the widespread occurrence of fescue toxicity in the south-east of the USA and other regions of the world (Hume et al. Citation2016). The toxicity appeared to be particularly severe in New Zealand as a combination of Epichloë strains and the grass host resulted in extensive endophyte colonisation of leaves leading to high concentrations of the mammalian toxic ergovaline alkaloid (Christensen et al. Citation1998). While an equivalent study has not been undertaken for Australian-sourced material, the situation may be similar as at least in one region of South Australia toxicity arose from the use of tall fescue imported from New Zealand (Pulsford Citation1950).
Due to the toxicity of naturalised populations and its invasion of grazed pastures, tall fescue became known as a poisonous weed particularly in northern regions of New Zealand (Glassey and Reade Citation1986) and some areas of Australia (Pulsford Citation1950). In New Zealand, this greatly hindered the use of what is an agronomically valuable species used in temperate pastures throughout the world (Fribourg et al. Citation2009). Endophyte infection of these naturalised tall fescues would undoubtedly have played a significant role in its notoriety as a weed. Toxic endophytes are known to deter overgrazing (Waller Citation2009), contributing to a competitive advantage for Epichloë-infected plants. In addition, structured experiments evaluating cultivars infected with mammalian toxic and non-toxic endophytes compared with endophyte-free tall fescue, have clearly demonstrated substantially greater agronomic performance which is closely associated with reduced damage from a range of exotic and native insects, particularly in northern New Zealand (Pennell and Ball Citation1999; Popay et al. Citation2005; Hume et al. Citation2009). In the late twentieth century in New Zealand and Australia, after the toxicity problem of endophyte was recognised, tall fescue cultivars developed were bred free of endophyte. Today, tall fescue cultivars are widely available that contain selected Epichloë endophytes lacking the toxin ergovaline (Young et al. Citation2013).
Meadow fescue
Meadow fescue is uncommon in New Zealand with Edgar and Connor (Citation2000) commenting that many herbaria specimens of meadow fescue were incorrectly identified as tall fescue. In general, it is a species that is not well suited to New Zealand, requiring high fertility and high soil moisture, being well adapted to extremely cold climates in the Northern Hemisphere. However, the history of endophyte infection may have had a major impact on its persistence and performance, and therefore prevalence in the New Zealand landscape. This first became apparent when plants of meadow fescue from a Northland dairy farm were surveyed, as this population was increasing and providing significant feed in drought years, which was completely contrary to the expectations of the zone of adaptation for this species. All the plants sampled were infected with endophyte (Cooper Citation1996) and through subsequent testing it was found that endophyte in this population reduced insect damage and greatly improved agronomic performance, relative to endophyte-free meadow fescue, in Northland (Hume et al. Citation2009) and Canterbury (Fletcher et al. Citation2001) and proved to be essential for survival in other locations (D.E. Hume unpublished data). Through breeding and selection, endophyte-infected meadow fescue has become an option for New Zealand farmers (Stewart et al. Citation2014).
The origin of the Northland meadow fescue population was most likely through importation of commercial seed sown widely in swamps in this area in the early 1900s (L. Corkill pers. comm.). This was not the first report of meadow fescue infected with Epichloë in New Zealand, with Neill (Citation1941) observing Epichloë in meadow fescues from five locations and four lines of New Zealand seed. Surveying meadow fescue for endophyte infection may well reveal it is more broadly distributed in New Zealand than currently thought.
Sexual Epichloë of other fescues
Other species of the genus Festuca have been reported as infected with Epichloë in New Zealand () (Hedley and Braithwaite Citation1978; Latch et al. Citation1984; McKenzie and Latch Citation1984; Leuchtmann Citation1994; Rolston et al. Citation2002). These are mainly fine fescues which are colonised by a sexual form of Epichloë (E. festucae) in contrast to the asexual form of Epichloë colonising tall and meadow fescues. This aligns with observations elsewhere in the world for Festuca and Epichloë (Schardl Citation2010; Tadych et al. Citation2014).
Plants infected with sexual Epichloë can be asymptomatic and only develop the external choke disease of seed infloresences under certain environmental conditions. For F. rubra and F. glauca, choke has been recorded (Hedley and Braithwaite Citation1978; McKenzie and Latch Citation1984; Leuchtmann Citation1994) and observed (A.V. Stewart and M.J. Christensen unpublished data) in various regions of New Zealand. In commercial Festuca seed production fields of imported and locally sourced material, choke has seldom been seen but can be extensive on rare occasions (A.V. Stewart unpublished data).
No toxicity to livestock has been reported in New Zealand or globally for Festuca infected with E. festucae, despite the production of mammalian toxic alkaloids (Schardl et al. Citation2013b; Hume et al. Citation2016). In part this is due to grasses infected with sexual Epichloë generally having fewer and lower concentrations of the known alkaloids than grasses infected with asexual Epichloë (Leuchtmann et al. Citation2000). This is likely to have arisen through less evolutionary pressure for sexual Epichloë to produce high levels of defensive alkaloids (Bush et al. Citation1997), as unlike the asexual forms, they are not totally dependent on the fitness of the host grass for survival and propagation.
Future opportunities
Further discovery
The prospect that yet undiscovered Epichloë-grass associations are present in New Zealand is worthy of further investigation for both native and naturalised grasses. There are good possibilities of finding new associations as over half the taxa in New Zealand are Pooideae grasses, with these taxa almost exclusively within tribes recognised as hosting Epichloë ().
Identifying Epichloë-infected grasses can be difficult as not all populations may be infected or populations may be infected at low rates that are not detected given the sample size of the populations examined. This was evident for P. matthewsii as the first population to be surveyed from Lake Ohau was found to be free of Epichloë (Rolston et al. Citation2002), however, subsequent surveys found several infected populations but with a wide range in the proportion of infected plants (Leuchtmann et al. Citation2019). In addition, many of the native grasses occur in remote localities, or at low frequency, so are difficult to survey.
Standard methods for screening germplasm for the presence of Epichloë involve staining plant tissues to visualise the fungus. Fungus can also be isolated directly from infected tissues. In recent decades genetic tests have become available, such as the use of amplified repeat sequences (Simple Sequence Repeats) from the Epichloë genome, the use of such tests provides both an indication of infection and a method of typing strains of Epichloë that are present. We have taken such genetic tests to a new level to survey Epichloë in New Zealand using high throughput DNA extraction and high resolution melting genetic analyses. As Epichloë endophytes are seed transmitted, this technology was used to interrogate the genetic resources held as seed accessions in the Margot Forde Germplasm Centre, New Zealand's national genebank of grassland plants. Out of nearly 6000 Pooideae accessions examined, over 7% were found to be infected with Epichloë, including species from agronomically important grasses (W.R. Simpson unpublished data). These preliminary data suggest Epichloë are present in New Zealand in genera other than the Lolium and Festuca identified to date.
Introducing new Epichloë to New Zealand
There are good prospects in New Zealand for Epichloë to be used to provide agronomic benefits to valuable cultivated naturalised grasses other than Lolium and Festuca, without harmful effects on livestock. This not only includes grasses used in pastoral farming or turf, but also grasses grown for cereal grains.
Pasture grasses
Invertebrate pests of pastures are estimated to cause losses of NZ$1.7-2.3 billion per annum to the New Zealand pastoral industry (Ferguson et al. Citation2019). Epichloë endophytes are used as effective biocontrol agents to help manage these losses for ryegrasses, the major sown grass species, while other valuable but minor grasses such as prairie grass (Bromus catharticus) lack the protective properties imparted by Epichloë. New Zealand cultivars of prairie grass were first developed in the late 1900s as this species became renowned for its high cool-season growth, an ability to tolerate dry summers and a high potential to regenerate through natural reseeding (Stewart et al. Citation2014). However, these benefits proved difficult to realise on-farm due at least in part to damage from a range of invertebrate pests (Thom et al. Citation1992).
In other regions of the world, several species of Bromus are known to be infected with a range of Epichloë (Tadych et al. Citation2014) and have the potential to protect plants from insect damage as has been demonstrated for Argentine stem weevil (Bell and Prestidge Citation1991; Iannone et al. Citation2012). While mammalian toxins have been detected in some Epichloë-Bromus associations, others lack these toxins and have no known toxicity to livestock (Bell and Prestidge Citation1991; Iannone et al. Citation2012). Using an established laboratory technique, prairie grass could be inoculated with Epichloë sourced from other species of Bromus, however, even transfers between closely related grass species can be highly problematic due to incompatibility in new Epichloë-grass associations (Johnson et al. Citation2019). Epichloë-infected populations of prairie grasses have been documented in South America (Franco et al. Citation2015), and if proven safe for livestock, strains of Epichloë could be utilised in New Zealand bred cultivars to address the issue of susceptibility of prairie grass to insect damage.
Cereals
The cereal grasses within Tribe Hordeeae have for millennia been vitally important food crops for humans and a source of feed for animals (Feuillet and Muehlbauer Citation2009). While Epichloë have not been found in modern cereals such as wheat (Triticum spp.), barley (Hordeum vulgare) and rye (Secale cereale), they are present in other genera that are wild relatives (Elymus and Hordeum) from this tribe (Card et al. Citation2014). The use of endophytes to improve adaptation and yield of cereal production systems through development of Epichloë-cereal associations has been explored with some promising results (Simpson et al. Citation2014).
A key element in achieving this goal has been to establish compatible and stable mutualistic associations involving modern cultivated cereals and Epichloë isolated from wild grasses. Despite the formidable challenge given the genetic distance between these grasses, enduring symbioses have been formed, although unsurprisingly not all new associations are fully compatible (Simpson et al. Citation2014). As occurs in pasture grasses, host plant genetics have been critical in addressing issues of compromised shoot morphology, flowering and transmission of endophyte to seed of rye and wheat (Simpson et al. Citation2018). These achievements represent a world first, putting New Zealand at the cutting edge of Epichloë research and commercial application.
Role of new genetic technologies
Fundamental research has provided considerable knowledge around Epichloë biochemistry including the identification of the genes encoding the biochemical pathways for the four classes of endophyte alkaloids. Comprehensive understanding of these pathways has created the opportunity to manipulate them (Schardl et al. Citation2013a). The Royal Society Te Apārangi (Citation2018) has recently published a technical paper that highlights the potential use of gene edited Epichloë endophytes to respond to insect pests and environmental stress. Techniques used for gene editing, such as CRISPR-Cas9 allow for the precise insertion, removal or replacement of genes at predetermined sites (Cong et al. Citation2013) and is being widely deployed to engineer crop plants internationally (Komor et al. Citation2017).
Gene editing techniques provide the means to precisely delete Epichloë genes to generate strains unable to synthesise mammalian toxins but still capable of accumulating compounds with useful bioactivity against pests (Johnson et al. Citation2013a). In addition, it is possible to engineer completely new secondary metabolite pathways, through the introduction of genes from other organisms, including other Epichloë species, to confer entirely new properties. As such, these new technologies have the potential to rapidly advance Epichloë endophyte research and provide new genetic variation to deliver endophytes for grasses that further increase yield and reliability, as well as improve animal health, welfare and productivity, whilst also reducing use of synthetic chemistry for a better environment.
Conclusion
Epichloë fungal endophytes play a role in the biology of both wild and cultivated cool-season grasses worldwide. Early in New Zealand’s European agricultural history, grasses were unknowingly imported with resident Epichloë. These infected grasses have been shown to be producers of secondary metabolites that are often toxic to grazing animals and/or provide insect resistance to the host grass. Research efforts over several decades have resulted in the identification of Epichloë that can be used to replace these toxic strains with those that produce metabolites that protect the grasses from biotic and abiotic stresses without the production of unmanageable mammalian toxins. Research has also shown that Epichloë are present in native and endemic New Zealand grasses. These native grasses and their endophytes do not present immediate opportunities for pastoral agriculture, however further research may identify uses for these and other introduced Epichloë in agricultural systems. Regardless, their identification places New Zealand amongst the regions in the world where Epichloë naturally occur and represent a biological taonga to Aotearoa (New Zealand). Epichloë fungal endophytes play a fundamental role in New Zealand’s introduced pastures and turf species while further research is required on their presence and ecological role in our native grasses.
Disclosure statement
No potential conflict of interest was reported by the author(s).
ORCID
David E. Hume http://orcid.org/0000-0002-9223-4853
Alan V. Stewart http://orcid.org/0000-0002-4147-0119
Wayne R. Simpson http://orcid.org/0000-0003-0061-3571
Richard D. Johnson http://orcid.org/0000-0003-2100-6658
Additional information
Funding
References
- Aldous DE, Isaacs S, Mebalds MI. 1999. Endophytes in the genus Neotyphodium are not found in Australian native grasses. Australasian Plant Pathology. 28:183–186. doi: 10.1071/AP99031
- Anonymous. 1918. A case of ergotism. New Zealand Journal of Agriculture. 17:263–264.
- Bell NL, Prestidge RA. 1991. The effects of the endophytic fungus Acremonium starii on feeding and oviposition of the Argentine stem weevil. Proceedings of the New Zealand Weed and Pest Control Conference. 44:181–184. doi: 10.30843/nzpp.1991.44.10828
- Bush LP, Wilkinson HH, Schardl CL. 1997. Bioprotective alkaloids of grass-fungal endophyte symbioses. Plant Physiology. 114:1–7. doi: 10.1104/pp.114.1.1
- Campbell MA, Tapper BA, Simpson WR, Johnson RD, Mace W, Ram A, Lukito Y, Dupont PY, Johnson LJ, Scott DB, et al. 2017. Epichloë hybrida, sp. nov., an emerging model system for investigating fungal allopolyploidy. Mycologia. 109:715–729.
- Caradus J, Lovatt S, Belgrave B. 2013. Adoption of forage technologies. Proceedings of the New Zealand Grassland Association. 75:39–44.
- Card SD, Faville MJ, Simpson WR, Johnson RD, Voisey CR, De Bonth ACM, Hume DE. 2014. Mutualistic fungal endophytes in the Triticeae – survey and description. FEMS Microbial Ecology. 88:94–106. doi: 10.1111/1574-6941.12273
- Christensen MJ, Bennett RJ, Ansari HA, Koga H, Johnson RD, Bryan GT, Simpson WR, Koolaard JP, Nickless EM, Voisey CR. 2008. Epichloë endophytes grow by intercalary hyphal extension in elongating grass leaves. Fungal Genetics and Biology. 45:84–93. doi: 10.1016/j.fgb.2007.07.013
- Christensen MJ, Easton HS, Simpson WR, Tapper BA. 1998. Occurrence of the fungal endophyte Neotyphodium coenophialum in leaf blades of tall fescue and implications for stock health. New Zealand Journal of Agricultural Research. 41:595–602. doi: 10.1080/00288233.1998.9513343
- Cong L, Ran FA, Cox D, Lin S, Barretto R, Habib N, Hsu PD, Wu X, Jiang W, Marraffini LA, et al. 2013. Multiplex genome engineering using CRISPR/Cas systems. Science. 339:819–823. doi: 10.1126/science.1231143
- Cooper BM. 1996. Evaluation of a meadow fescue (Festuca pratensis) population from Northland. Proceedings of the New Zealand Grassland Association. 57:69–72.
- Cooper BM, Hume DE, Panckhurst KA, Popay AJ. 2007. Agronomic responses of Italian ryegrasses infected with different Neotyphodium strains. In: Popay AJ, Thom ER, editor. Grassland research and practice series. Christchurch: New Zealand Grassland Association; p. 297–300.
- Cunningham IJ. 1948. Tall fescue grass is poison for cattle. New Zealand Journal of Agriculture. 77:519.
- Cunningham IJ. 1958. Non-toxicity to animals of ryegrass endophyte and other endophytic fungi of New Zealand grasses. New Zealand Journal of Agricultural Research. 1:489–497. doi: 10.1080/00288233.1958.10431534
- Cunningham IJ, Hartley WJ. 1959. Ryegrass staggers. New Zealand Veterinary Journal. 7:1–7. doi: 10.1080/00480169.1959.33317
- Davis LT, Guy PL. 2001. Introduced plant viruses and the invasion of a native grass flora. Biological Invasions. 3:89–95. doi: 10.1023/A:1011464804245
- de Lange PJ, Norton DA, Courtney SP, Heenan PB, Barkla JW, Cameron EK, Hitchmough R, Townsend AJ. 2009. Threatened and uncommon plants of New Zealand (2008 revision). New Zealand Journal of Botany. 47:61–96. doi: 10.1080/00288250909509794
- di Menna ME, Finch SC, Popay AJ, Smith BL. 2012. A review of the Neotyphodium lolii / Lolium perenne symbiosis and its associated effects on animal and plant health, with particular emphasis on ryegrass staggers. New Zealand Veterinary Journal. 60:315–328. doi: 10.1080/00480169.2012.697429
- DOC. 2000. The New Zealand biodiversity strategy. Department of Conservation; [accessed 2019 Oct 2]. https://www.doc.govt.nz/nature/biodiversity/nz-biodiversity-strategy-and-action-plan/new-zealand-biodiversity-strategy-2000-2020/.
- Easton HS, Lee CK, Fitzgerald RD. 1994. Tall fescue in Australia and New Zealand. New Zealand Journal of Agricultural Research. 37:405–417. doi: 10.1080/00288233.1994.9513078
- Eaton CJ, Cox MP, Scott B. 2011. What triggers grass endophytes to switch from mutualism to pathogenism? Plant Science. 180:190–195. doi: 10.1016/j.plantsci.2010.10.002
- Edgar E, Connor HE. 2000. Flora of New Zealand. Volume V. Gramineae. Lincoln: Manaaki Whenua Press.
- Faville MJ, Briggs L, Cao M, Koulman A, Jahufer MZZ, Koolaard J, Hume DE. 2015. A QTL analysis of host plant effects on fungal endophyte biomass and alkaloid expression in perennial ryegrass. Molecular Breeding. 35:161. doi: 10.1007/s11032-015-0350-1
- Ferguson CM, Barratt BIP, Bell N, Goldson SL, Hardwick S, Jackson M, Jackson TA, Phillips CB, Popay AJ, Rennie G, et al. 2019. Quantifying the economic cost of invertebrate pests to New Zealand’s pastoral industry. New Zealand Journal of Agricultural Research. 62:255–315. doi: 10.1080/00288233.2018.1478860
- Feuillet C, Muehlbauer GJ. editors. 2009. Genetics and genomics of the Triticeae. Dordrecht, Netherlands: Springer.
- Fletcher LR, Harvey IC. 1981. An association of a Lolium endophyte with ryegrass staggers. New Zealand Veterinary Journal. 29:185–186. doi: 10.1080/00480169.1981.34839
- Fletcher LR, Popay AJ, Stewart AV, Tapper BA. 2001. Herbage and sheep production from meadow fescue with and without the endophyte Neotyphodium uncinatum. The Grassland Conference 2000 Fourth International Neotyphodium/ Grass Interactions Symposium; Soest, Germany.
- Forde MB, Burgess RE, Halligan EA, Gardiner SE, Latch GCM. 1988. Varieties of Italian ryegrass in New Zealand. Proceedings of the New Zealand Grassland Association. 49:101–106.
- Franco MF, Colabelli MN, Petigrosso LR, De Battista JP, Echeverría MM. 2015. Evaluation of infection with endophytes in seeds of forage species with different levels of ploidy. New Zealand Journal of Agricultural Research. 58:181–189. doi: 10.1080/00288233.2015.1011283
- Fribourg HA, Hannaway DB, West CP. editors. 2009. Tall fescue for the twenty-first century. Agronomy Monograph. Vol. 53. Madison, Wisconsin, USA: American Society of Agronomy, Crop Science Society of America, Soil Science Society of America.
- Glassey CB, Reade JB. 1986. The elimination and replacement of wild tall fescue in Northland dairy pastures. Proceedings of the New Zealand Grassland Association. 47:187–190.
- Guy PL, Davis LT. 2002. Disease notes or new records: variation in the incidence of barley yellow dwarf virus and in the ability of Neotyphodium endophytes to deter feeding by aphids (Rhopalosiphum padi) on Australasian tall fescue. Australasian Plant Pathology. 31:307–308. doi: 10.1071/AP02032
- Hassing B, Winter D, Becker Y, Mesarich CH, Eaton CJ, Scott B. 2019. Analysis of Epichloë festucae small secreted proteins in the interaction with Lolium perenne. PLoS ONE. 14(2):e0209463. doi: 10.1371/journal.pone.0209463
- Hedley J, Braithwaite M. 1978. Choke, a disease of grasses caused by Epichloe typhina (Pers). Tul. Bulletin of the Royal New Zealand Institute of Horticulture. 9:6–9.
- Hume DE, Cooper BM, Panckhurst KA. 2009. The role of endophyte in determining the persistence and productivity of ryegrass, tall fescue and meadow fescue in Northland. Proceedings of the New Zealand Grassland Association. 71:145–150.
- Hume DE, Ryan GD, Gibert A, Helander M, Mirlohi A, Sabzalian MR. 2016. Chapter 6, Epichloë fungal endophytes for grassland ecosystems. In: Lichtfouse E, editor. Sustainable agriculture reviews. Switzerland: Springer International Publishing; p. 233–305.
- Hume DE, Sewell JC. 2014. Agronomic advantages conferred by endophyte infection of perennial ryegrass (Lolium perenne L.) and tall fescue (Festuca arundinacea Schreb.) in Australia. Crop and Pasture Science. 65:747–757. doi: 10.1071/CP13383
- Iannone LJ, Novas MV, Young CA, De Battista JP, Schardl CL. 2012. Endophytes of native grasses from South America: biodiversity and ecology. Fungal Ecology. 5:357–363. doi: 10.1016/j.funeco.2011.05.007
- Inda LA, Segarra-Moragues JG, Müller J, Peterson PM, Catalán P. 2008. Dated historical biogeography of the temperate Loliinae (Poaceae, Pooideae) grasses in the northern and southern hemispheres. Molecular Phylogenetics and Evolution. 46(3):932–957. doi: 10.1016/j.ympev.2007.11.022
- Johnson LJ, Caradus JR, 2019. The science required to deliver Epichloë endophytes to commerce. In: Hodkinson TR, Doohan FM, Saunders MJ, et al. editor. Endophytes for a growing world. Cambridge: Cambridge University Press; p. 343–370.
- Johnson LJ, De Bonth ACM, Briggs LR, Caradus JR, Finch SC, Fleetwood DJ, Fletcher LR, Hume DE, Johnson RD, Popay AJ, et al. 2013a. The exploitation of epichloae endophytes for agricultural benefit. Fungal Diversity. 60:171–188. doi: 10.1007/s13225-013-0239-4
- Johnson LJ, Koulman A, Christensen M, Lane GA, Fraser K, Forester N, Johnson RD, Bryan GT, Rasmussen S. 2013b. An extracellular siderophore is required to maintain the mutualistic interaction of Epichloë festucae with Lolium perenne. PLoS Pathogens. 9(5): e1003332. doi: 10.1371/journal.ppat.1003332
- Johnson LJ, Voisey CR, Faville MJ, Moon CD, Simpson WR, Johnson RD, Stewart AV, Caradus JR, Hume DE. 2019. Advances and perspectives in breeding for improved grass-endophyte associations. Grassland Science in Europe. 24:351–363.
- Jung GA, Van Wijk AJP, Hunt WF, Watson CE. 1996. Chapter 19, ryegrasses. In: Moser LE, Buxton DR, Casler MD, editor. Cool-season forage grasses. Madison, Wisconsin: ASA, CSSA and SSSA; p. 605–641.
- Kaur J, Ekanayake PN, Tian P, Van Zijll De Jong E, Dobrowolski MP, Rochfort SJ, Mann RC, Smith KF, Forster JW, Guthridge KM, et al. 2015. Discovery and characterisation of novel asexual Epichloë endophytes from perennial ryegrass (Lolium perenne L.). Crop and Pasture Science. 66:1058–1070. doi: 10.1071/CP14332
- Komor AC, Badran AH, Liu DR. 2017. CRISPR-based technologies for the manipulation of eukaryotic genomes. Cell. 168:20–36. doi: 10.1016/j.cell.2016.10.044
- Langer RHM. editor. 1990. Pastures their ecology and management. Auckland: Oxford University Press.
- Latch GCM, Christensen MJ, Hickson RE. 1988. Endophytes of annual and hybrid ryegrasses. New Zealand Journal of Agricultural Research. 31:57–63. doi: 10.1080/00288233.1988.10421364
- Latch GCM, Christensen MJ, Samuels GJ. 1984. Five endophytes of Lolium and Festuca in New Zealand. Mycotaxon. 20:535–550.
- Latch GCM, Tapper BA. 1988. Lolium endophytes - problems and progress. Proceedings of the Japanese Association of Mycotoxicology. 1:220–223. doi: 10.2520/myco1975.1988.1Supplement_220
- Leuchtmann A. 1994. Isozyme relationships of Acremonium endophytes from twelve Festuca species. Mycological Research. 98:25–33. doi: 10.1016/S0953-7562(09)80331-6
- Leuchtmann A, Bacon CW, Schardl CL, White Jr JF, Tadych M. 2014. Nomenclatural realignment of Neotyphodium species with genus Epichloë. Mycologia. 106:202–215. doi: 10.3852/13-251
- Leuchtmann A, Schmidt D, Bush LP. 2000. Different levels of protective alkaloids in grasses with stroma-forming and seed-transmitted Epichloë/Neotyphodium endophytes. Journal of Chemical Ecology. 26:1025–1036. doi: 10.1023/A:1005489032025
- Leuchtmann A, Young CA, Stewart AV, Simpson WR, Hume DE, Scott B. 2019. Epichloë novae-zelandiae, a new endophyte from the endemic New Zealand grass Poa matthewsii. New Zealand Journal of Botany. 57:271–288. doi: 10.1080/0028825X.2019.1651344
- Malinowski DP, Belesky DP. 2000. Adaptations of endophyte-infected cool-season grasses to environmental stresses: mechanisms of drought and mineral stress tolerance. Crop Science. 40:923–940. doi: 10.2135/cropsci2000.404923x
- McKenzie EHC, Latch GCM. 1984. New plant disease records in New Zealand: Graminicolous fungi. New Zealand Journal of Agricultural Research. 27:113–123. doi: 10.1080/00288233.1984.10425739
- Miles CO, Menna M, Jacobs SWL, Garthwaite I, Lane GA, Prestidge RA, Marshall SL, Wilkinson HH, Schardl CL, Ball OJP, et al. 1998. Endophytic fungi in indigenous Australasian grasses associated with toxicity to livestock. Applied and Environmental Microbiology. 64:601–606. doi: 10.1128/AEM.64.2.601-606.1998
- Ministry for Primary Industries. 2016. How valuable is that plant species? application of a method for enumerating the contribution of selected plant species to New Zealand’s GDP. MPI technical paper No: 2016/62. Wellington, New Zealand: Ministry for Primary Industries.
- Moon CD, Miles CO, Järlfors U, Schardl CL. 2002. The evolutionary origins of three new Neotyphodium endophyte species from grasses indigenous to the Southern Hemisphere. Mycologia. 94:694–711. doi: 10.1080/15572536.2003.11833197
- Moon CD, Scott DB, Schardl CL, Christensen MJ. 2000. The evolutionary origins of Epichloë endophytes from annual ryegrasses. Mycologia. 92:1103–1118.
- Mortimer PH, Fletcher LR, Menna M, Harvey IC, Smith GS, Barker GM, Gallagher RT, White EP. 1982. Recent advances in ryegrass staggers. Proceedings of the Ruakura farmers’ conference. 34:71–74.
- Neill JC. 1940. The endophyte of ryegrass (Lolium perenne). New Zealand Journal of Science and Technology. 21A:280–291.
- Neill JC. 1941. The endophytes of Lolium and Festuca. New Zealand Journal of Science and Technology. 23A:185–193.
- Neill JC. 1952. Note on agronomic effect of the endophyte Lolium perenne. New Zealand Journal of Science and Technology. 34A:230.
- Popay AJ, Hume DE. 2011. Endophytes improve ryegrass persistence by controlling insects. In: Mercer CF, editor. Pasture persistence symposium grassland research and practice series No 15. Dunedin: New Zealand Grassland Association; p. 149–156.
- Popay AJ, Jensen JG, Cooper BM. 2005. The effect of non-toxic endophytes in tall fescue on two major insect pests. Proceedings of the New Zealand Grassland Association. 67:169–173.
- Pennell C, Ball OJ-P. 1999. The effects of Neotyphodium endophytes in tall fescue on pasture mealy bug (Balanococcus poae). Proceedings of the New Zealand Plant Protection Conference. 52:259–263.
- Prestidge RA. 1991. Susceptibility of Italian ryegrasses (Lolium multiflorum Lam.) to Argentine stem weevil (Listronotus bonariensis (Kuschel)) feeding and oviposition. New Zealand Journal of Agricultural Research. 34:119–125. doi: 10.1080/00288233.1991.10417801
- Pulsford MF. 1950. A note on lameness in cattle grazing on tall meadow fescue (Festuca arundinacea) in South Australia. The Australian Veterinary Journal. 26:87–88. doi: 10.1111/j.1751-0813.1950.tb04880.x
- Rolston MP, Stewart AV, Latch GCM, Hume DE. 2002. Endophytes in New Zealand grass seeds: occurrence and implications for conservation of grass species. New Zealand Journal of Botany. 40:365–372. doi: 10.1080/0028825X.2002.9512797
- Royal Society Te Apārangi. 2018. Gene editing in the primary industries. technical paper. Wellington, New Zealand: Royal Society Te Apārangi.
- Schardl CL. 2010. The epichloae, symbionts of the grass subfamily Poideae. Annals of the Missouri Botanical Garden. 97:646–665. doi: 10.3417/2009144
- Schardl CL, Craven KD, Speakman S, Stromberg A, Lindstrom A, Yoshida R. 2008. A novel test for host-symbiont codivergence indicates ancient origin of fungal endophytes in grasses. Systematic Biology. 57(3):483–498. doi: 10.1080/10635150802172184
- Schardl CL, Leuchtmann A, Tsai HF, Collett MA, Watt DM, Scott DB. 1994. Origin of a fungal symbiont of perennial ryegrass by interspecific hybridization of a mutualist with the ryegrass choke pathogen, Epichloë typhina. Genetics. 136:1307–1317.
- Schardl CL, Young CA, Hesse U, Amyotte SG, Andreeva K, Calie PJ, Fleetwood DJ, Haws DC, Moore N, Oeser B, et al. 2013a. Plant-Symbiotic fungi as chemical engineers: multi-genome analysis of the Clavicipitaceae reveals dynamics of alkaloid Loci. PLoS Genetics. 9(2):e1003323. doi: 10.1371/journal.pgen.1003323
- Schardl CL, Young CA, Pan J, Florea S, Takach JE, Panaccione DG, Farman ML, Webb JS, Jaromczyk J, Charlton ND, et al. 2013b. Currencies of mutualisms: Sources of alkaloid genes in vertically transmitted epichloae. Toxins. 5:1064–1088. doi: 10.3390/toxins5061064
- Schirrmann MK, Zoller S, Croll D, Stukenbrock EH, Leuchtmann A, Fior S. 2018. Genomewide signatures of selection in Epichloë reveal candidate genes for host specialization. Molecular Ecology. 27:3070–3086. doi: 10.1111/mec.14585
- Scott B, Takemoto D, Tanaka A. 2007. Fungal endophyte production of reactive oxygen species is critical for maintaining the mutualistic symbiotic interaction between Epichloë festucae and perennial ryegrass. Plant Signaling & Behavior. 2:171–173. doi: 10.4161/psb.2.3.3725
- Simpson WR, Faville MJ, Moraga RA, Williams WM, McManus MT, Johnson RD. 2014. Epichloë fungal endophytes and the formation of synthetic symbioses in Hordeeae (=Triticeae) grasses. Journal of Systematics and Evolution. 52:794–806. doi: 10.1111/jse.12107
- Simpson WR, Popay AJ, Mace WJ, Hume DE, Johnson RD. 2018. Creating synthetic symbioses between Epichloë and rye (Secale cereale) to improve crop performance. In: Zabalgogeazcoa I, Vázquez de Aldana BR, editor. International symposium on fungal endophyte of grasses. Salamanca, Spain; p. 96.
- Simpson WR, Schmid J, Singh J, Faville MJ, Johnson RD. 2012. A morphological change in the fungal symbiont Neotyphodium lolii induces dwarfing in its host plant Lolium perenne. Fungal Biology. 116:234–240. doi: 10.1016/j.funbio.2011.11.006
- Soreng RJ, Peterson PM, Romaschenko K, Davidse G, Zuloaga FO, Judziewicz EJ, Filgueiras TS, Davis JI, Morrone O. 2015. A worldwide phylogenetic classification of the Poaceae (Gramineae). Journal of Systematics and Evolution. 53:117–137. doi: 10.1111/jse.12150
- Spatafora JW, Sung GH, Sung JM, Hywel-Jones NL, White JF. 2007. Phylogenetic evidence for an animal pathogen origin of ergot and the grass endophytes. Molecular Ecology. 16:1701–1711. doi: 10.1111/j.1365-294X.2007.03225.x
- Stewart A. 2005a. The potential for domestication and seed propagation of native New Zealand grasses for turf. In: Dawson M, editor. Greening the city: Bringing diversity back into the urban environment. Lincoln, New Zealand: Royal New Zealand Institute of Horticulture; p. 277–284.
- Stewart AV. 2005b. The commercial use of endophyte in turf ryegrass in New Zealand. International Turfgrass Society Research Journal. 10:641–644.
- Stewart A, Hume D, Latch G, Tapper B, Simpson W. 2004. Poa matthewsii, a New Zealand grass endemic with an endophyte. In: Kallenback R, Rosenkrans C, Lock TR, editor. International symposium on Neotyphodium/grass interactions. Fayetteville, Arkansas, USA: University of Arkansas; p. Poster 217.
- Stewart A, Kerr G, Lissaman W, Rowarth J. 2014. Chapter 5, grasses. In: Davis K, Casey M, editor. Pasture and forage plants for New Zealand grassland research and Practice Series No 8. Fourth ed. Palmerston North, New Zealand: New Zealand Grassland Association; p. 25–44.
- Stewart AV. 1989. Ceres Progrow Westerwolds ryegrass (Lolium multiflorum Lam.). New Zealand Journal of Agricultural Research. 32:125–126. doi: 10.1080/00288233.1989.10423487
- Sugawara K, Inoue T, Yamashita M, Ohkubo H. 2006. Distribution of the endophytic fungus, Neotyphodium occultans in naturalized Italian ryegrass in western Japan and its production of bioactive alkaloids known to repel insect pests. Japanese Society of Grassland Science. 52:147–154. doi: 10.1111/j.1744-697X.2006.00060.x
- Tadych M, Bergen MS, White Jr JF. 2014. Epichloë spp. associated with grasses: New insights on life cycles, dissemination and evolution. Mycologia. 106:181–201. doi: 10.3852/106.2.181
- Tan YY, Spiering MJ, Scott V, Lane GA, Christensen MJ, Schmid J. 2001. In planta regulation of extension of an endophytic fungus and maintenance of high metabolic rates in its mycelium in the absence of apical extension. Applied and Environmental Microbiology. 67:5377–5383. doi: 10.1128/AEM.67.12.5377-5383.2001
- Thom ER, Popay AJ, Hume DE, Fletcher LR. 2012. Evaluating the performance of endophytes in farm systems to improve farmer outcomes - a review. Crop and Pasture Science. 63:927–943. doi: 10.1071/CP12152
- Thom ER, Prestidge RA, Wildermoth DD, Taylor MJ, Marshall SL. 1992. Effect of Hessian fly (Mayetiola destructor) on production and persistence of prairie grass (Bromus willdenowii) when rotationally grazed by dairy cows. New Zealand Journal of Agricultural Research. 35:75–82. doi: 10.1080/00288233.1992.10417704
- Waller JC. 2009. Chapter 9, endophyte effects on cattle. In: Fribourg HA, Hannaway DB, West CP, editor. Tall fescue for the twenty-first century. Madison, Wisconsin, USA: American Society of Agronomy, Crop Science Society of America, Soil Science Society of America; p. 289–310.
- Young CA, Hume DE, McCulley RL. 2013. Fungal endophytes of tall fescue and perennial ryegrass: pasture friend or foe? Journal of Animal Science. 91:2379–2394. doi: 10.2527/jas.2012-5951