ABSTRACT
Inflammatory bowel disease is the umbrella term for a group of diseases whose incidence has been increasing over the past century. Although initial literature analyses had suggested a small number of genes, perhaps between 10 and 20, responsible for disease susceptibility, subsequent national and international collaborations have significantly modified those estimations to greater than 240, with more expected as the technologies continue to advance. As well as direct effects on the genome providing possible targets for therapy or functional foods, it is clear that the immune system, the epi-genome and the gut microbiome also play an important role in disease development and progression. While certain foods accelerate disease development in individuals carrying specific genotypes, the same foods appear beneficial to other genetic groups. Gene-nutrient-microbiota interactions also become important, and tissue culture or animal models are available to initially test hypotheses. However, genomic technologies are necessary to reveal all major effects, whether beneficial or otherwise, in animal or human studies. These technologies include transcriptomics, proteomics and metabolomics, which reveal details of what has happened in a gene-diet or gene-drug interaction. At an international level, the field originally called nutrigenomics, has now been divided into two complementary fields: nutrigenetics and nutrigenomics.
Introduction: why is there increasing interest in inflammatory bowel disease?
Inflammatory bowel disease (IBD) was originally considered to comprise two distinct diseases: Crohn's disease (CD) and ulcerative colitis (UC) (Satsangi et al. Citation1994, Citation1996; Orchard et al. Citation2000). The incidence of IBD varies across different countries and different racial groups, and has been changing with time. For example, Eason et al. (Citation1982) reported that 456 patients with UC and 137 patients with CD attended public hospitals within Auckland, New Zealand, between the years 1969 and 1978. Although Polynesians comprised 15% of the population in this area between these same years, they represented only 0.4% of UC cases, and no CD cases. The annual incidence rates over these years were 5.4/100,000 Caucasians with UC and 1.75/100,000 with CD. At that time, CD was significantly less common in New Zealand than in European and North American centres. Considering those patients presenting for the first time during the course of the study, the cumulative probability of surviving 10 years was 93.9% for UC and 89.1% for CD. Eason et al. (Citation1982) further reported that clinical features and local complications of both forms of IBD correlated with the anatomic location of the disease.
By 2012, Coppell et al. (Citation2018) reported an incidence of 6.3/100,000 with UC and 21.8/100.000 with CD, in a mostly Caucasian New Zealand group. Kaplan (Citation2015) provided an international perspective of the global burden of IBD, describing a surge in worldwide IBD rates since the middle of the twentieth century. By 2015, IBD showed a prevalence of 0.5% of the population in the Western world, including New Zealand. Indeed, by that time, New Zealand and Australia were included among the most affected countries, worldwide. This has significant implications for both human health in general, and health care costs across the nation.
The genetic basis of inflammatory bowel disease
Familial aspects of IBD were recognised and reported as early as the 1970s (Singer et al. Citation1971). In their 1994 review, Satsangi et al. (Citation1994) reported the use of genome scanning techniques using microsatellite markers, to implicate specific areas of chromosomes linked to disease, while association studies of specific genes or gene complexes, such as the human leukocyte antigen (HLA) gene complex, which encodes the major histocompatibility complex (MHC) proteins, were also linked to the risk of this disease in humans. HLA or cytokine genes were considered in early IBD studies to aid in mechanistic understandings, and this association was reinforced by subsequent findings (Satsangi et al. Citation1996). The possible involvement of the X chromosome was also suggested at an early stage, since there was a somewhat higher number of reported cases in women than in men (Vermeire et al. Citation2001). The situation appeared considerably more complex than a single gene disorder, and by the year 2000, Orchard and co-workers estimated the involvement of at least somewhere between 10 and 20 genes in disease susceptibility (Orchard et al. Citation2000). One gene that appeared of considerable importance in these early studies was the nucleotide-binding oligomerization domain-containing protein 2 (NOD2) gene (Hugot et al. Citation2001). This gene, also known as caspase recruitment domain-containing protein 15 (CARD15) or inflammatory bowel disease protein 1 (IBD1), is a protein that is encoded by the NOD2 gene, located on chromosome 16 in humans.
Early studies analysed specific hypothesised genetic variants as single nucleotide polymorphisms (SNPs), linked to disease risk (Satsangi et al. Citation1994, Citation1996). However, subsequent studies used whole genome scans as genome-wide association studies (GWAS) (e.g. Karban et al. Citation2004; Low et al. Citation2004; Mathew and Lewis Citation2004). These studies examine SNPs across the genome, and require large numbers of subjects. In a number of these studies, IBD proved to be a good illustrative example of technology developments in defining the causes of genetic susceptibility. There was also good evidence that diet played an important role in the disease, but considerable confusion as to the best dietary advice to give (e.g. Harries and Rhodes Citation1985; Rhodes and Rose Citation1986; Gassull and Cabré Citation2001). For these reasons, the disease was selected as the prototype example of gene-diet interactions in the government-funded Nutrigenomics New Zealand (NuNZ) programme, which ran between 2004 and 2014.
An early step in the development of NuNZ was to establish a local cohort of individuals with IBD, and an age and sex-matched groups of control subjects. For logistic reasons, the cohort was based in the North Island, especially Auckland. The disease subjects were recruited through gastroenterology clinics or other doctor's surgeries, while the control subjects were mostly volunteers who responded to media publicity. For the patients, records and diagnoses were carefully scrutinised, and they were reclassified as necessary utilising Montreal criteria. This classification system utilises an integrated clinical, molecular and serological classification of IBD (Satsangi et al. Citation2006). Individuals of European descent make up by far the majority of this New Zealand population at risk (Eason et al. Citation1982; Gearry et al. Citation2010). Given the time available, the numbers of subjects that our statisticians advised sampling and the possibility that disease causes could vary across different ethnicities, only Caucasians were utilised in the Nutrigenomics New Zealand study (Han et al. Citation2010; Triggs et al. Citation2010).
By 2007, NuNZ had succeeded in accumulating a cohort of 389 CD patients, 405 UC patients and 416 population controls (Browning et al. Citation2007a, Citation2007b, Citation2007c). Some of the early NuNZ publications revealed that the New Zealand cohort was not completely identical with cohorts described from other countries. For example, subjects with variants in the Disks large homolog 5 (DLG5) gene showed a reduced rather than the previously reported increased risk of the disease (Browning et al. Citation2007b). Additionally, the population size numbers, coupled with meta-analysis technologies enabled the identification of some genes not previously extensively studied, such as Toll-like receptor 4 (TLR4), as strong risk factors in this population (Browning et al. Citation2007c). However, most of the early publications confirmed that Caucasians in New Zealand showed similar disease-associated genetic variants to other Caucasian populations (e.g. Petermann et al. Citation2009a, Citation2009b; Marlow et al. Citation2013b, Marlow et al. Citation2015a, Citation2015b). The interest generated by the early publications led to NuNZ being invited to be part of the International IBD Genetics Consortium (IIBDGC). This collaboration has led to important international understandings of IBD genetics, partly because of the high number of individuals now being studied, and partly also because of developments in genetic and genomic technologies
One of the first key findings of the IIBDGC was published by Jostins et al. (Citation2012). By this time, the consortium consisted of more than 75,000 cases and similar numbers of controls. This group of diseases by then was affecting over 2.5 million people of European ancestry, and the prevalence was rising in other populations. GWAS studies and subsequent meta-analyses had implicated previously unsuspected mechanisms, such as autophagy (a mechanism that removes unnecessary or dysfunctional cellular components) in disease pathogenesis. These studies had also indicated that some IBD loci are shared with other inflammatory diseases. The data analysis by Jostins et al. identified 71 new associations, leading to a total of 163 IBD loci that met significance thresholds. It was also of considerable interest that several pathways were shared between host responses to mycobacteria, and predisposition to IBD. These data also raised the probability that weak or inappropriate immune response may be involved in disease susceptibility.
In 2016, another key paper from this consortium was published by Cleynen et al. (Citation2016). By this time, it was recognised that more than 200 distinct genetic loci were involved in disease susceptibility. This update expanded the range of subjects studied, including patients from 49 centres across 16 countries in Europe, North America and Australasia. Cleynen and co-workers studied 34, 619 patients (19,713 with CD, 14, 683 with UC), genotyped on the Immunochip array. This array is a genotyping platform that considers 195 806 genetic variants, derived from previous association studies of immune-related disorders, including but not restricted to, CD and UC. The authors then used these data to test (and prove) the hypothesis that colonic CD, ileal CD and UC were genetically distinct. Three loci – NOD2, MHC and Macrophage stimulating protein 3p21 (MST 3p21) – were associated with these three sub-phenotypes of IBD. Disease location was identified as an intrinsic aspect of a patient's disease, and as one of the major drivers of disease behaviour and changes over time.
In 2018, a further publication including the IIBDGC amongst several other large consortia (Momozawa et al. Citation2018) recognised over 240 loci associated with risk for IBD, and utilised newer technologies as well as an even larger set of individuals. These newer technologies included transcriptional profiling of specific cell subsets (Furey et al. Citation2019; Lee and Cleynen Citation2019). Transcriptional profiling considers the range of genes that are over- or under-expressed during disease development or progression, but has proved less informative in studying IBD than hoped, because of confounding factors such as medication use and disease severity (Lee and Cleynen Citation2019). More useful were the genome scale molecular phenotyping approaches used by Furey et al. (Citation2019), to compare diseased and non-diseased subjects in relation to their host gene and microRNA expression, the state of the chromatin landscape and the composition of the gut microbiota. Zhang et al. (Citation2015) defined molecular phenotyping as the technique of quantifying pathway reporter genes, i.e. pre-selected genes that are modulated specifically by metabolic and signalling pathways, in order to infer the activity of these pathways. The analysis by Furey et al. (Citation2019) enabled a more precise redefining of these pathways in IBD. This study generated a large transcriptome dataset (looking at the expression of genes from nine disease-relevant cell types) and identified 23,650 expression quantitative trait loci (eQTL). eQTL have been described as genomic loci that explain all or a fraction of variation in the levels of expression of messenger RNAs (Rockman and Kruglyak Citation2006). Furey et al. (Citation2019) confirmed the involvement of at least 240 genetic variants in this disease, but identified that approximately ten-fold higher numbers of human subjects would be needed to fully quantify the disease risk associated with individual genes or combinations thereof.
Human observational studies of gene-diet interactions in IBD
Although genetics has been identified as crucial in susceptibility to these diseases (Satsangi et al. Citation1994, Citation1996; Orchard et al. Citation2000), disease development is also susceptible to the influence of environmental factors, especially diet (Han et al. Citation2010; Triggs et al. Citation2010). These authors analysed data from the New Zealand cohort described above. An extensive food frequency questionnaire was developed, with 257 food items in 15 groups, to find self-reported dietary tolerances and intolerances. Across each of the food groups, there were statistically significant differences among the probability that certain foods or food groups would prove either detrimental or beneficial. While there were a small number of foods that appeared to be generally considered beneficial, such as white fish, gluten-free products, bananas and yoghurt, there were also a considerable number that generally appeared detrimental, including sweet corn and its’ products, chilli or chilli sauce, peanuts, cream and salami. Interestingly, however, for a number of food items, the same item that was beneficial to one group appeared detrimental to others. A sizable range of these was found, including soya milk, goats’ milk, kiwi fruit, ginger and ginger products, beef, liver and oily fish (Han et al. Citation2010; Triggs et al. Citation2010). It was not possible to identify a specific diet that could be recommended to a new patient developing the disease. These data supported the need for personalisation of diets for individuals with IBD. However, the authors also recognised that the numbers of participants in the New Zealand cohort was not sufficient to rule out the possibilities of false positive effects or reverse causality.
The human cohort described above enabled the testing of specific hypotheses, across a range of people who were currently showing symptoms of the diseases. In 2010, Nolan and co-workers specifically considered the effects of adult lactase persistence in CD (Nolan et al. Citation2010). This characteristic was associated with an increased risk of the disease and a specific genetic signature, which they in turn linked to high levels of Mycobacterium avium subspecies paratuberculosis, found in ruminants and milk products. Given that dairy products are important to the New Zealand economy, it seemed important to further question their potential role in initiating this disease and/or accelerating disease development. Indeed, Nolan-Clark et al. (Citation2011) were able to demonstrate that effects of dairy products on disease were only clinically apparent for those products with a high fat content, and in patients showing colonic inflammation.
Brassica vegetables are generally recommended to be part of a healthy diet plan (Folkard et al. Citation2015). This group includes broccoli, cabbage, cauliflower, Chinese greens, rocket, watercress, horseradish and wasabi. These vegetables contain many significant nutrients, including dietary fibres, antioxidants such as vitamin A and C, folate and vitamin K, minerals such as potassium, calcium, selenium and zinc, as well as various phytochemicals that are generally considered beneficial to health (Ferguson Citation2001). Sulforaphane is a compound produced by the hydrolytic conversion of glucoraphanin after the ingestion of cruciferous vegetables, particularly broccoli and broccoli sprouts. This compound has been extensively studied due to its’ apparent health-promoting properties in disease, limited toxicity in normal tissue, and some apparent anticancer effects (Folkard et al. Citation2015). It does, however, have a somewhat bitter taste, which some people find offensive (Laing et al. Citation2013). Brassica were another group of vegetables associated with tolerance or intolerance in various CD patients, with beneficial effects relating to carrying 37 different SNPs, or detrimental effects associated with 64 SNPs. Two of these effects, one in the deiodinase iodothyronine, type 1 (D101) gene and another in the HLA (major histocompatibility complex, class II, DO alpha) gene remained significant after multiple testing corrections (Laing et al. Citation2013). Information on the genotype of these two genes could be especially important in providing the correct dietary advice for CD patients. Probiotics and prebiotics, which modulate the gut microbiota, also appeared beneficial in several patient groups (Baker et al. Citation2009; Huebner et al. Citation2011; Gentschew and Ferguson Citation2012; Laing et al. Citation2018, Citation2019).
When beneficial dietary components were compared with genetic data, it could generally be seen that good dietary advice for individuals correlated with their genetic data, also segregating into the three major genetics groups (NOD2, MHC and MST 3p21) that had been identified as relating to disease location (Ferguson Citation2015; Cleynen et al. Citation2016). If the primary genetic changes are those affecting inflammation, then recognised anti-inflammatory medicines, or foods such as oily fish, containing long omega-3 polyunsaturated fatty acids, might be particularly beneficial. In contrast, if genes affecting microbial diversity are predominant (Baker et al. Citation2009; Gentschew and Ferguson Citation2012), then either a probiotic capsule (containing live beneficial bacteria), or prebiotic foods that stimulate the growth of beneficial bacteria (such as Brassica vegetables, garlic or various sources of dietary fibre) may be particularly useful (Lim et al. Citation2005; Ferguson and Schlothauer Citation2012; Marlow et al. Citation2015a, Citation2015b; Wong et al. Citation2016). Both vitamin D and various phytochemicals may play roles in both these processes, and are likely to benefit IBD patients if taken at higher than normal recommended levels (Ferguson Citation2015). These data support the types of therapeutic interventions in IBD suggested by Plichta et al. (Citation2019), Furey et al. (Citation2019) and Lee and Cleynen (Citation2019). A diagrammatic summary of some of the key factors implicated in IBD risk and/or progression is provided in .
Figure 1. This illustrates the overlaps between two key groups of genes, in both humans and their gut bacteria, and key influences on these. While human genes determine key sensitivities, they are greatly impacted by the nature of the gut microbiome. The expression of human genes is impacted strongly by both diet and medication, and these effects might be beneficial or detrimental, depending upon their nature and the specific human genes involved in disease susceptibility. Inflammation, however caused, is likely to have an adverse effect. The gut microbiome is strongly influenced by early life nutrition, and breast feeding plays an important role. Antibiotics may be beneficial, but if used to excess, may be detrimental. Diet is also of key importance, and both probiotics and prebiotics are designed to benefit the gut microbiome.
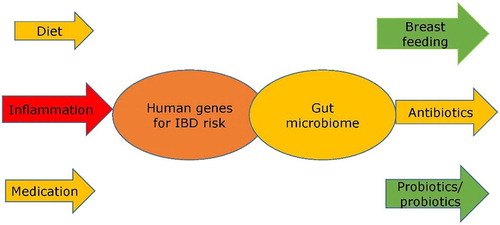
In vitro model systems to test the capacity of dietary components to modulate detrimental genetic influences
While a number of gastrointestinal cell lines are commercially available, it has not always been clear that these are relevant to IBD. The importance of cell culture models has been emphasised by Philpott et al. (Citation2007). With the increasing publication of GWAS studies on genes involved in the inheritance of these diseases, it became possible to scan a range of existing cell lines for disease relevance. Huebner and co-workers (Citation2010) genotyped 50 IBD markers across 32 susceptibility genes in 9 commonly used gastrointestinal cell lines. They used Sequenom, TaqMan and DNA sequencing for genotyping, and determined the expression profile of selected genes using real time RT–PCR. Genetic variants such as Multidrug resistance 1 (MDR1) altered the response to drugs, while TLR2, TLR4 and NOD2 had important effects on bacterial recognition. Huebner et al. (Citation2011), were able to show potentially beneficial effects of a probiotic on genes related to IBD in a tissue culture model.
Nasef et al. (Citation2014b) recognised that pattern recognition receptors such as TLR2 and TLR4 are very important in detecting and responding to stress and bacterial stimuli. These authors considered whether fractions of fruits regularly eaten in New Zealand might show effects on the expression of such genes. Relevant cell lines were exposed to inflammatory stress, then treated with different extracts from one of 12 fractionated fruits. The strongest anti-inflammatory effects were seen for extracts of feijoa and blackberry. The effects of blackberry were not unexpected, since these contain high levels of polyphenols, generally considered to be beneficial to health (Ferguson Citation2001). However, feijoa, the fruit of Acca sellowiana, is not so widely grown, and the taste may be slightly tart for individuals either with or without IBD. A literature survey by Nasef et al. (Citation2014a), suggested that one mechanism of their action could be through autophagy, an important process known to regulate inflammation. Nasef et al. (Citation2015) more specifically showed beneficial effects of extracts of feijoa on TLR2 signalling and activation of autophagy in a specially developed cell model.
Broccoli has a somewhat bitter taste, partly associated with the presence of sulforophane (Ferguson and Schlothauer Citation2012). Folkard et al. (Citation2015) considered whether there could be beneficial effects of sulforophane on the processes known to be involved in inflammation in IBD. Interestingly, this compound proved to have particularly beneficial effects in controlling inflammation, at least in this cell model. Such work may suggest that a feijoa or broccoli extract could be incorporated into a functional food product, which might slightly mask the flavour found unpleasant by some (Ferguson et al. Citation2010). It is important, however, to stress the importance of moving on from cell culture models, since these do not correct for the variety of other factors, including gut microbiota and metabolism, that occur in animal models or in humans.
Animal models to test the capacity of dietary components to modulate detrimental genetic influences
Now that the genetic characteristics of IBD are emerging more clearly, it becomes easier to select (or develop) appropriate animal models to test effects of foods or dietary supplements, before suggesting them for use in the very vulnerable people, either susceptible to or currently showing symptoms of the disease. As well as analytical developments in genotyping, transcriptomics. proteomics and metabolomics are now available to nutritional research, and used increasingly in these studies (van Ommen and Stierum Citation2002; Barnett et al. Citation2015; Ferguson and Barnett Citation2016; ). Barnett et al. (Citation2015) emphasised the value of all of these nutrigenomics technologies in various animal models. Proteomics (the study of proteins), combining two-dimensional gel electrophoresis with liquid chromatography-mass spectrometry (LCMS), provides an analysis of peptides. Metabolomics is formally defined as the study of chemical processes involving metabolites, the small molecule intermediates and products of metabolism. Metabolomics using both gas chromatograph-MS (GCMS) and LCMS have been combined with transcript-omics (gene expression analysis), proteomics and analyses of microbiota to give a comprehensive picture of which foods may be beneficial in individuals carrying susceptibility genes. These tools can non-invasively be applied to animal or human studies.
Figure 2. Potential sequence of in vitro to in vivo studies, including the application of omics technologies. This flow diagram shows a potential approach, working from in vitro studies in tissue culture models, through in vivo studies in animals, to human clinical trials, with the application of omics technologies at several stages. (1) There is increasing use of high-throughput techniques, such as single nucleotide polymorphism (SNP) chips, which enable genome-wide assessment to identify genetic factors which may be linked to a particular disease or health outcome. Relevant SNPs can then be incorporated into suitable in vitro assays (2) in which food compounds can be assessed for their abilities to interact with the SNP of interest and modulate its function. Effective food compounds can then be tested in appropriate animal models (3) which exhibit the relevant phenotype and/or have the SNP of interest (or one in a related gene). This can initially be used to establish if the food has an effect on phenotype and, subsequently a range of omics techniques can be applied, and data derived from them integrated, to better understand the mechanism by which a food may exert its’ effect. Food compounds which show efficacy both in vitro and in vivo may then be suitable as candidates for human studies, both pre-clinically (for example, to assess any possible biomarkers identified in the animal studies (4)) and, finally, clinically, to ascertain a clinically-relevant end-point, such as an improvement of IBD-associated symptoms (5). The Manhattan plot shown in (1) was originally published by Ikram et al. (Citation2010) was obtained from Wikipedia (https://commons.wikimedia.org/wiki/File:Manhattan_Plot.png). This image file is licensed under the Creative Commons Attribution 2.5 Generic license. Reproduced with permission from Ferguson and Barnett (Citation2016).
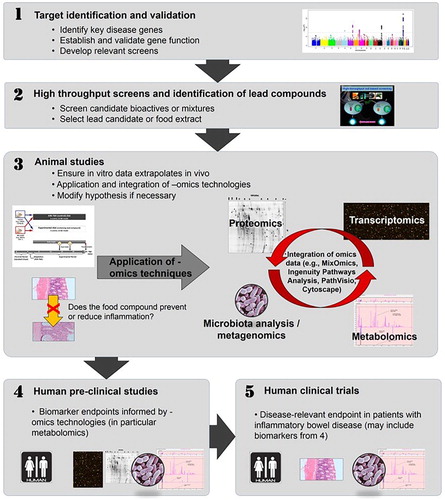
Blood, urine and faecal samples can be taken before and after a specific dietary intervention. Changes in gene expression can be monitored by transcriptomic profiles from peripheral blood mononuclear cells. Urine and/or faecal samples can be used for metabolomics and proteomic techniques, to enable the measurement of dietary compliance and also provide biomarkers of disease progression (Lin et al. Citation2009, Citation2010; Citation2011a, Citation2011b; Ferguson Citation2010, Citation2012, Citation2013, Citation2015). The faecal microbes also play a key role in disease development, as emphasised by Jostins et al. (Citation2012). Therefore measuring effects of dietary interventions on the microbiome is also highly desirable (Baker et al. Citation2009; Gentschew and Ferguson Citation2012; Ferguson Citation2015).
One of the genes shown to be involved in the development of CD is Interleukin-10, an immunosuppressive cytokine involved in the regulation of gastrointestinal mucosal immunity toward intestinal microbiota. The interleukin-10-deficient (IL10-/-) mouse is a variant of C57BL/6 mice, that develops intestinal inflammation unless raised in germ-free conditions, and has been used as a mouse model of CD. Lin et al. (Citation2009) used non-targeted urinary profiling to study systemic metabolic changes associated with the development of intestinal inflammation in this mouse. Spot urine samples were collected from IL10-/- and the control mice at 5.5, 7, 8.5, and 10.5 weeks of age. Urine samples were analysed by gas chromatography-mass spectrometry (GCMS), while statistical analysis utilised XCMS software, multiple t tests, and ANOVA. Elevated urinary levels of xanthurenic acid and fucose in IL10-/- mice, relative to those in wild type mice, indicated upregulation of tryptophan catabolism and perturbed fucosylation caused by this mutation. Other data also suggested the downregulation of fatty acid oxidation in the variant mice. These metabolites were thus identified as potential markers of intestinal inflammation. Further investigation of these mouse models (Lin et al. Citation2010) showed fifteen further metabolite differences, including fucose, xanthurenic acid, and 5-aminovaleric acid, that were associated with intestinal inflammation. Elevated urinary levels of xanthurenic acid in the variant mouse were attributed to an increased production of kynurenine metabolites that may induce T-cell tolerance toward intestinal microbiota.
Importantly, this same genetic difference in the IL10 gene also affects responses to foods that are potentially important to the New Zealand food industry. Thus, Lin et al. (Citation2011a, Citation2011b) considered the metabolic effects of consuming extracts from the fruits of yellow (Actinidia chinensis) or green-fleshed (A. deliciosa) kiwifruit. These extracts had previously shown in vitro anti-inflammatory activity, and their effects were investigated in the IL-10 mouse model, as compared to effects in wild type mice. The primary endpoint was metabolomic analysis of urine samples. Kiwifruit-derived metabolites appeared at significantly higher levels in the urine of the variant mice relative to those of wild-type mice, suggesting they may be excreted more rapidly, and therefore be less beneficial to mice (or humans) carrying this genetic variant. It should be stressed that this has still not been proved in such individuals. Nevertheless, this study demonstrates the use of metabolomic analysis to study the influence of genotype on food metabolism, and is likely to have implications for the development of functional foods and also medication in the treatment of human IBD.
Also relevant to the New Zealand food industry are the studies by Espley et al. (Citation2013, Citation2014). These authors used novel plant breeding techniques to develop a variant of the Royal Gala apple with red skin and flesh, high in flavonoids that have antioxidant potential. Their initial studies considered the potential of such an apple for the human market, by seeking consumer opinions on the taste and appearance of the novel apple (Espley et al. Citation2013). These initial studies gave positive feedback, and encouraged them to continue their studies. Espley et al. (Citation2014) used a wild type animal model to test the effects of a diet containing this red-fleshed apple, in comparison with the parent strain of apple, on various endpoints relevant to IBD. Endpoints were considered after 7 or 21 days of this supplemented diet. The authors showed that the red-fleshed apple but not the control apple significantly reduced the expression of inflammation-related genes, and also led to potentially beneficial changes in the composition of the gut microbiota.
The value of nutrigenomics technologies in testing new hypotheses in human subjects
While our various study approaches enable the development of hypotheses on desirable diets in specific IBD groups, it is clearly unethical to place human subjects onto such diets, and wait to see if and when disease symptoms appear. The evidence would support reducing the intake of certain foods, but compensating for any possible nutritional deficiencies. Nutrigenomics applies genomic technologies which can detect potential beneficial (or unpredicted detrimental) effects on disease development before medical symptoms can be detected (Ferguson and Barnett Citation2016; ). It must be stressed that these -omics technologies would be in addition to more conventional markers used in traditional human intervention studies.
Transcriptomics, applied to human blood samples in an intervention study, measures effects on the expression of many genes, including those associated with early stages of an inflammatory response. Marlow et al. (Citation2013a) used transcriptomics as an endpoint to study the effect of a 6 week intervention with a Mediterranean-inspired diet on inflammation in CD patients. The study examined changes in inflammation and also in the composition of the gut microbiome, comparing the results of established biomarkers of inflammation, including C-reactive protein and the micronucleus assay (Fenech Citation2000), with results from a transcriptomic approach. Data showed that being on this diet for 6 weeks was able to slightly reduce the established biomarkers of inflammation. However, using transcriptomics, highly significant changes in gene expression were shown. Although no single gene stood out, the cumulative effect of small changes in many genes combined to have a beneficial effect. Data also showed that this type of diet resulted in a trend towards normalising the gut microbiome.
The growing understanding of the genes involved with disease susceptibility, coupled with the use of multi-omics technologies, enables more rapid and less invasive testing of new products of potential value to the New Zealand food and supplement industries. An example of this is the study on the effects of supplementation of human diets with a novel olive leaf extract (Boss et al. Citation2016). Although non-diseased subjects were volunteers in the study, the pattern of gene regulation strongly supported the view that this novel extract could be of benefit to IBD-susceptible individuals. There was also good reason to believe that diets enhanced with long chain omega-3 polyunsaturated fatty acids could be beneficial, and novel foods utilising nutrition, food science and engineering could be usefully produced (Ferguson et al. Citation2010). A kiwifruit extract enhanced gluten-free bread also showed evidence for beneficial use as a functional food in such populations (Sun-Waterhouse et al. Citation2009).
How are recent or on-going genetic studies adding value to our health advice in other populations and/or ethnic groups?
The formation of Nutrigenomics New Zealand occurred at a fortuitous time, enabling those working with IBD patients a means of overcoming food intolerances and nutrient deficiencies in these subjects (Laing et al. Citation2019), and also adding to a growing international database showing the importance of regulating gene-diet interactions in order to reduce disease susceptibility and optimise health. It became part of an international initiative to publicise and recognise the importance of nutrigenetics, often referred to as personalised nutrition (Kaput et al. Citation2005; Fenech et al. Citation2011; Bush et al. Citation2020). Even now, the field has not been internationally regulated and there are some companies exploiting public misunderstandings. Kohlmeier et al. (Citation2016) and Grimaldi et al. (Citation2017) proposed internationally endorsed guidelines to evaluate the scientific basis for strength of evidence of personalised nutrition (genotype-based dietary advice), not only in IBD, but also in a wider range of diseases. The summary of these recommendations was developed as a framework, reproduced here as . These guidelines reinforce the value of recognising, and responding to, such well characterised gene-diet interactions as the need for folate supplementation in those individuals carrying a variant in the methylene-tetrahydrofolate reductase gene, who have a reduced enzyme activity related to efficacy of folate utilisation. The recommendations also stress the danger of dietary supplementations that are based on flawed or incomplete scientific evidence that has not been replicated.
Table 1. Framework for critical assessment of the evidence relating to gene × diet interactions (Grimaldi et al. Citation2017).
It is important to stress that this was only ever considered as a preliminary study, and very pleasing to see that an Asia-Pacific Association of Gastroenterology (APAGE) Working Group of Inflammatory Bowel Disease has now been established, to focus on diagnosis, epidemiology and management of the IBD in different racial groups in this area (Ooi et al. Citation2016a, Citation2016b). The technologies described in this review are also being applied to other diseases of both national and international importance, including cancer (Nasir et al. Citation2019), obesity and cardiovascular disease (Peña-Romero et al. Citation2018). Indeed, this latter paper describes nutrigenomics and nutrigenetics as the future of nutrition research. I would completely agree with this conclusion.
Conclusions
The timing of establishment of Nutrigenomics New Zealand, and the decision to use IBD as a prototype disease for study, were highly fortuitous. Technologic developments during the twenty first century have resulted in what appeared to be a simple disease with a small number of genes involved in susceptibility, to a much more complex group of diseases. Nutrigenetics techniques have revealed how an understanding of the genetic disease basis can be applied to both novel dietary interventions and new therapeutic opportunities. The technological developments (nutrigenomics) used in this project can now be applied to other diseases of importance worldwide that have a genetic susceptibility, but are also modulated by diet and/or gut microbiota.
Acknowledgements
I am greatly appreciative of the Royal Society of New Zealand for making me a Fellow. The research described in this manuscript is from a 10 year collaboration among The University of Auckland, AgResearch and Plant and Food Ltd. I am very grateful to all members of the highly committed and hard-working team that made up Nutrigenomics New Zealand. FRST must also be praised highly for their recognition of the emerging importance of nutrigenomics as a field likely to be of future benefit to the New Zealand food industry.
Disclosure statement
No potential conflict of interest was reported by the author(s).
Additional information
Funding
References
- Baker PI, Love DR, Ferguson LR. 2009. Role of gut microbiota in Crohn’s disease. Expert Review of Gastroenterology & Hepatology. 3(5):535–546.
- Barnett M, Young W, Cooney J, Roy N. 2015. Metabolomics and proteomics, and what to do with all these ‘omes’: insights from nutrigenomic investigations in New Zealand. Journal of Nutrigenetics and Nutrigenomics. 7(4-6):274–282.
- Boss A, Kao CH, Murray PM, Marlow G, Barnett MP, Ferguson LR. 2016. Human intervention study to assess the effects of supplementation with olive leaf extract on peripheral blood mononuclear cell gene expression. International Journal of Molecular Sciences. 17(12):2019.
- Browning BL, Annese V, Barclay ML, Bingham SA, Brand S, Buning C, Castro M, Cucchiara S, Dallapiccola B, Drummond H, et al. 2007a. Gender-stratified analysis of DLG5 R30Q in 4707 patients with Crohn disease and 4973 controls from 12 Caucasian cohorts. Journal of Medical Genetics. 45(1):36–42.
- Browning BL, Huebner C, Petermann I, Demmers P, McCulloch A, Gearry RB, Barclay ML, Shelling AN, Ferguson LR. 2007b. Association of DLG5 variants with inflammatory bowel disease in the New Zealand Caucasian population and meta-analysis of the DLG5 R30Q variant. Inflammatory Bowel Diseases. 13(9):1069–1076.
- Browning BL, Huebner C, Petermann I, Gearry RB, Barclay ML, Shelling AN, Ferguson LR. 2007c. Has toll-like receptor 4 been prematurely dismissed as an inflammatory bowel disease gene? Association study combined with meta-analysis shows strong evidence for association. The American Journal of Gastroenterology. 102(11):2504–2512.
- Bush CL, Blumberg JB, El-Sohemy A, Minich DM, Jóse M, Ordovás JM, Reed DG, Behm VAY. 2020. Towards the definition of personalized nutrition: a proposal by the American Nutrition Association. Journal of the American College of Nutrition. 39(1):5–15.
- Cleynen I, Boucher G, Jostins L, Schumm LP, Zeissig S, Ahmad T, Andersen V, Andrews JM, Annese V, Brand S, et al. 2016. Inherited determinants of Crohn’s disease and ulcerative colitis phenotypes: a genetic association study. Lancet. 387(10014):156–167.
- Coppell KJ, Galts CP, Huizing FY, Norton JK, Gray AR, Schultz K, Hobbs CE, Aluzaite K, Schultz M. 2018. Annual incidence and phenotypic presentation of IBD in Southern New Zealand: An 18-year epidemiological analysis. Inflammatory Intestinal Diseases. 3(1):32–39.
- Eason RJ, Lee SP, Tasman-Jones C. 1982. Inflammatory bowel disease in Auckland, New Zealand. Australian and New Zealand Journal of Medicine. 12(2):125–131.
- Espley RV, Bovy A, Bava C, Jaeger SR, Tomes S, Norling C, Crawford J, Rowan D, McGhie TK, Brendolise C, et al. 2013. Analysis of genetically modified red-fleshed apples reveals effects on growth and consumer attributes. Plant Biotechnology Journal. 11(4):408–419.
- Espley RV, Butts CA, Laing WA, Martell S, Smith H, McGhie TK, Zhang J, Paturi G, Hedderley D, Bovy A, et al. 2014. Dietary flavonoids from modified apple reduce inflammation markers and modulate gut microbiota in mice. The Journal of Nutrition. 144(2):146–154.
- Fenech M. 2000. The in vitro micronucleus technique. Mutation Research/Fundamental and Molecular Mechanisms of Mutagenesis. 455:81–95.
- Fenech M, El-Sohemy A, Cahill L, Ferguson LR, French TA, Tai ES, Milner J, Koh W-P, Xie L, Zucker M, et al. 2011. Nutrigenetics and nutrigenomics: viewpoints on the current status and applications in nutrition research and practice. Journal of Nutrigenetics and Nutrigenomics. 4(2):69–89.
- Ferguson LR. 2001. Role of plant polyphenols in genomic stability. Mutation Research/Fundamental and Molecular Mechanisms of Mutagenesis. 475(1-2):89–111.
- Ferguson LR. 2010. Nutrigenomics and inflammatory bowel diseases. Expert Review of Clinical Immunology 6(4):573–583.
- Ferguson LR. 2012. Potential value of nutrigenomics in Crohn’s disease. Nature Reviews Gastroenterology & Hepatology 9(5):260–270.
- Ferguson LR. 2013. Nutrigenetics, nutrigenomics and inflammatory bowel diseases. Expert Review of Clinical Immunology. 9(8):717–726.
- Ferguson LR. 2015. Nutritional modulation of gene expression: might this be of benefit to individuals with Crohn’s disease? Frontiers in Immunology. 6:467.
- Ferguson LR, Barnett MP. 2016. Why are omics technologies important to understanding the role of nutrition in inflammatory bowel diseases? International Journal of Molecular Sciences. 17(10):E1763.
- Ferguson LR, Schlothauer RC. 2012. The potential role of nutritional genomics tools in validating high health foods for cancer control: broccoli as example. Molecular Nutrition & Food Research. 56(1):126–146.
- Ferguson LR, Smith BG, James BJ. 2010. Combining nutrition, food science and engineering in developing solutions to inflammatory bowel diseases–omega-3 polyunsaturated fatty acids as an example. Food & Function. 1(1):60–72.
- Folkard DL, Marlow G, Mithen RF, Ferguson LR. 2015. Effect of sulforaphane on NOD2 via NF-κB: implications for Crohn’s disease. Journal of Inflammation. 12:6.
- Furey TS, Sethupathy P, Sheikh SZ. 2019. Redefining the IBDs using genome-scale molecular phenotyping. Nature Reviews Gastroenterology & Hepatology. 16(5):296–231.
- Gassull MA, Cabré E. 2001. Nutrition in inflammatory bowel disease. Current Opinion in Clinical Nutrition and Metabolic Care. 4:561–569.
- Gearry RB, Richardson AK, Frampton CM, Dodgshun AJ, Barclay ML. 2010. Population-based cases control study of inflammatory bowel disease risk factors. Journal of Gastroenterology and Hepatology. 25(2):325–333.
- Gentschew L, Ferguson LR. 2012. Role of nutrition and microbiota in susceptibility to inflammatory bowel diseases. Molecular Nutrition & Food Research. 56(4):524–535.
- Grimaldi KA, van Ommen B, Ordovas JM, Parnell LD, Mathers JC, Bendik I, Brennan L, Celis-Morales C, Cirillo E, Daniel H, et al. 2017. Proposed guidelines to evaluate scientific validity and evidence for genotype-based dietary advice. Genes & Nutrition. 12:35.
- Han DY, Fraser AG, Dryland P, Ferguson LR. 2010. Environmental factors in the development of chronic inflammation: a case-control study on risk factors for Crohn’s disease within New Zealand. Mutation Research/Fundamental and Molecular Mechanisms of Mutagenesis. 690(1-2):116–122.
- Harries A, Rhodes J. 1985. Undernutrition in Crohn’s disease: an anthropometric assessment. Clinical Nutrition. 4:87–89.
- Huebner C, Ding Y, Petermann I, Knapp C, Ferguson LR. 2011. The probiotic Escherichia coli Nissle 1917 reduces pathogen invasion and modulates cytokine expression in Caco-2 cells infected with Crohn’s disease-associated E. coli LF82. Applied and Environmental Microbiology. 77(7):2541–2544.
- Huebner C, Petermann I, Lam WJ, Shelling AN, Ferguson LR. 2010. Characterization of single-nucleotide polymorphisms relevant to inflammatory bowel disease in commonly used gastrointestinal cell lines. Inflammatory Bowel Diseases. 16(2):282–295.
- Hugot JP, Chamaillard M, Zouali H, Lesage S, Cézard J-P, Belaiche J, Almer S, Tysk C, O’Morain CA, Gassull M, et al. 2001. Association of NOD2 leucine-rich repeat variants with susceptibility to Crohn’s disease. Nature. 411(6837):599–603.
- Ikram MK, Xueling S, Jensen RA, Cotch MF, Hewitt AW, Ikram MA, Wang JJ, Klein R, Klein BEK, Breteler MMB, et al. 2010. Four novel Loci (19q13, 6q24, 12q24, and 5q14) influence the microcirculation in vivo. PLoS Genetics. 6:e1001184.
- Jostins L, Ripke S, Weersma RK, Duerr RH, McGovern DP, Hui KY, Lee JC, Philip Schumm L, Sharma Y, Anderson CA, et al. 2012. Host-microbe interactions have shaped the genetic architecture of infammatory bowel disease. Nature. 491:119–124.
- Kaplan GG. 2015. The global burden of IBD: from 2015 to 2025. Nature Reviews Gastroenterology & Hepatology. 12(12):720–727.
- Kaput J, Ordovas JM, Ferguson L, van Ommen B, Rodriguez RL, Allen L, Ames BN, Dawson K, German B, Krauss R, et al. 2005. The case for strategic international alliances to harness nutritional genomics for public and personal health. British Journal of Nutrition. 94(5):623–632.
- Karban AS, Okazaki T, Panhuysen CI, Gallegos T, Potter JJ, Bailey-Wilson JE, Silverberg MS, Duerr RH, Cho JH, Gregersen PK, et al. 2004. Functional annotation of a novel NFKB1 promoter polymorphism that increases risk for ulcerative colitis. Human Molecular Genetics. 13(1):35–45.
- Kohlmeier M, De Caterina R, Ferguson LR, Görman U, Allayee H, Prasad C, Kang JX, Nicoletti CF, Martinez JA. 2016. Guide and position of the international society of nutrigenetics/nutrigenomics on personalized nutrition: part 2 – ethics, challenges and endeavours of precision nutrition. Journal of Nutrigenetics and Nutrigenomics. 9(1):28–46.
- Laing B, Barnett MPG, Marlow G, Nasef NA, Ferguson LR. 2018. An update on the role of gut microbiota in chronic inflammatory diseases, and potential therapeutic targets. Expert Review of Gastroenterology & Hepatology. 12(10):969–983.
- Laing B, Han DY, Ferguson LR. 2013. Candidate genes involved in beneficial or adverse responses to commonly eaten brassica vegetables in a New Zealand Crohn’s disease cohort. Nutrients. 5(12):5046–5064.
- Laing BB, Lim AG, Ferguson LR. 2019. A personalised dietary approach—a way forward to manage nutrient deficiency, effects of the western diet, and food intolerances in inflammatory bowel disease. Nutrients. 11(7):E1532.
- Lee HS, Cleynen I. 2019. Molecular profiling of inflammatory bowel disease: is it ready for use in clinical decision-making? Cells. 8(6):E535–E554.
- Lim CC, Ferguson LR, Tannock GW. 2005. Dietary fibres as “prebiotics”: implications for colorectal cancer. Molecular Nutrition & Food Research. 49(6):609–619.
- Lin HM, Barnett MP, Roy NC, Joyce NI, Zhu S, Armstrong K, Helsby NA, Ferguson LR, Rowan DD. 2010. Metabolomic analysis identifies inflammatory and non-inflammatory metabolic effects of genetic modification in a mouse model of Crohn’s disease. Journal of Proteome Research. 9(4):1965–1975.
- Lin HM, Edmunds SI, Helsby NA, Ferguson LR, Rowan DD. 2009. Non-targeted urinary metabolite profiling of a mouse model of Crohn’s disease. Journal of Proteome Research. 8(4):2045–2057.
- Lin HM, Edmunds SJ, Zhu S, Helsby NA, Ferguson LR, Rowan DD. 2011a. Metabolomic analysis reveals differences in urinary excretion of kiwifruit-derived metabolites in a mouse model of inflammatory bowel disease. Molecular Nutrition & Food Research. 55(12):1900–1904.
- Lin HM, Helsby NA, Rowan DD, Ferguson LR. 2011b. Using metabolomic analysis to understand inflammatory bowel diseases. Inflammatory Bowel Diseases. 17(4):1021–1029.
- Low JH, Williams FA, Yang X, Cullen S, Colley J, Ling KL, Armuzzi A, Ahmad T, Neville MJ, Dechairo BM, et al. 2004. Inflammatory bowel disease is linked to 19p13 and associated with ICAM-1. Inflammatory Bowel Diseases. 10(3):173–181.
- Marlow GJ, Ellett S, Ferguson IR, Zhu S, Karunasinghe N, Jesuthasan AC, Han D, Fraser AG, Ferguson LR. 2013a. Transcriptomics to study the effect of a Mediterranean-inspired diet on inflammation in Crohn’s disease patients. Human Genomics. 7:24.
- Marlow GJ, Han DY, Triggs CM, Ferguson LR. 2015a. Food intolerance: associations with the rs12212067 polymorphism of FOXO3 in Crohn’s disease patients in New Zealand. Journal of Nutrigenetics and Nutrigenomics. 8(2):70–80.
- Marlow GJ, Han DY, Wickens K, Stanley T, Crane J, Mitchell EA, Dekker J, Barthow C, Fitzharris P, Ferguson LR, et al. 2015b. Differential effects of two probiotics on the risks of eczema and atopy associated with single nucleotide polymorphisms to Toll-like receptors. Pediatric Allergy and Immunology. 26(3):262–271.
- Marlow GJ, van Gent D, Ferguson LR. 2013b. Why interleukin-10 supplementation does not work in Crohn's disease patients. World Journal of Gastroenterology. 19(25):3931–3941. doi:10.3748/wjg.v19.i25.3931.
- Mathew CG, Lewis CM. 2004. Genetics of inflammatory bowel disease: progress and prospects. Human Molecular Genetics. 13(90001):161R–1168.
- Momozawa Y, Dmitrieva J, Théâtre E, Deffontaine V, Rahmouni S, Charloteaux B, Crins F, Docampo E, Elansary M, Gori A-S, et al. 2018. IBD risk loci are enriched in multigenic regulatory modules encompassing putative causative genes. Nature Communications. 9(1):2427.
- Nasef NA, Mehta S, Ferguson LR. 2014a. Dietary interactions with the bacterial sensing machinery in the intestine: the plant polyphenol case. Frontiers in Genetics. 5:64.
- Nasef NA, Mehta S, Murray P, Marlow GJ, Ferguson LR. 2014b. Anti-inflammatory activity of fruit fractions in vitro, mediated through toll-like receptor 4 and 2 in the context of inflammatory bowel disease. Nutrients. 6(11):5265–5279.
- Nasef NA, Mehta S, Powell P, Marlow G, Wileman T, Ferguson LR, Ojcius DM. 2015. Extracts of feijoa inhibit toll-like receptor 2 signaling and activate autophagy implicating a role in dietary control of IBD. PLoS One. 10(6):e0130910.
- Nasir A, Bullo MMH, Ahmed Z, Imtiaz A, Yaqoob E, Jadoon M, Ahmed H, Afreen A, Yaqoob S. 2019. Nutrigenomics: epigenetics and cancer prevention: a comprehensive review. Critical Reviews in Food Science and Nutrition. 59:1–13.
- Nolan-Clark D, Tapsell LC, Hu R, Han DY, Ferguson LR. 2011. Effects of dairy products on Crohn’s disease symptoms are influenced by fat content and disease location but not lactose content or disease activity status in a New Zealand population. Journal of the American Dietetic Association. 111(8):1165–1172.
- Nolan DJ, Han DY, Lam WJ, Morgan AR, Fraser AG, Tapsell LC, Ferguson LR. 2010. Genetic adult lactase persistence is associated with risk of Crohn’s disease in a New Zealand population. BMC Research Notes. 3:339.
- Ooi CJ, Makharia GK, Hilmi I, Gibson PR, Fock KM, Ahuja V, Ling KL, Lim WC, Thia KT, Wei S-C, et al. 2016a. Asia Pacific consensus statements on Crohn’s disease. part 1: definition, diagnosis, and epidemiology. Journal of Gastroenterology and Hepatology. 31(1):45–55.
- Ooi CJ, Makharia GK, Hilmi I, Gibson PR, Fock KM, Ahuja V, Ling KL, Lim WC, Thia KT, Wei S-C, et al. 2016b. Asia-Pacific consensus statements on Crohn’s disease. Part 2: management. Journal of Gastroenterology and Hepatology. 31(1):56–68.
- Orchard TR, Satsangi J, Van Heel D, Jewell DP. 2000. Genetics of inflammatory bowel disease: a reappraisal. Scandinavian Journal of Immunology. 51(1):10–17.
- Peña-Romero AC, Navas-Carrillo D, Marín F, Orenes-Piñero E. 2018. The future of nutrition: nutrigenomics and nutrigenetics in obesity and cardiovascular diseases. Critical Reviews in Food Science and Nutrition. 58(17):3030–3041.
- Petermann I, Huebner C, Browning BL, Gearry RB, Barclay ML, Kennedy M, Roberts R, Shelling AN, Philpott M, Han DY, et al. 2009a. Interactions among genes influencing bacterial recognition increase IBD risk in a population-based New Zealand cohort. Human Immunology. 70(6):440–446.
- Petermann I, Triggs CM, Huebner C, Han DY, Gearry RB, Barclay ML, Demmers PS, McCulloch A, Ferguson LR. 2009b. Mushroom intolerance: a novel diet-gene interaction in Crohn’s disease. British Journal of Nutrition. 102(4):506–508.
- Philpott M, Mackay L, Ferguson LR, Forbes D, Skinner M. 2007. Cell culture models in developing nutrigenomics foods for inflammatory bowel disease. Mutation Research/Fundamental and Molecular Mechanisms of Mutagenesis. 622(1-2):94–102.
- Plichta DR, Graham DB, Subramanian S, Xavier RJ. 2019. Therapeutic opportunities in inflammatory bowel disease: mechanistic dissection of host-microbiome relationships. Cell. 178(5):1041–1056.
- Rhodes J, Rose J. 1986. Does food affect acute inflammatory bowel disease? The role of parenteral nutrition, elemental and exclusion diets. Gut. 27(5):471–474.
- Rockman MV, Kruglyak L. 2006. Genetics of global gene expression. Nature Reviews Genetics. 7(11):862–872.
- Satsangi J, Jewell DP, Rosenberg WM, Bell JI. 1994. Genetics of inflammatory bowel disease. Gut. 35(5):696–700.
- Satsangi J, Silverberg MS, Vermeire S, Clombel JF. 2006. The Montreal classification of inflammatory bowel disease: controversies, consensus, and implications. Gut. 55:749–753.
- Satsangi J, Welsh KI, Bunce M, Julier C, Farrant JM, Bell JI, Jewell DP. 1996. Contribution of genes of the major histocompatibility complex to susceptibility and disease phenotype in inflammatory bowel disease. Lancet. 347(9010):1212–1217.
- Singer HC, Anderson JG, Frischer H, Kirsner JB. 1971. Familial aspects of inflammatory bowel disease. Gastroenterology. 61(4):423–430.
- Sun-Waterhouse D, Chen J, Chuah C, Wibisono R, Melton LD, Laing W, Ferguson LR, Skinner MA. 2009. Kiwifruit-based polyphenols and related antioxidants for functional foods: kiwifruit extract-enhanced gluten-free bread. International Journal of Food Sciences and Nutrition. 60(Suppl 7):251–264.
- Triggs CM, Munday K, Hu R, Fraser AG, Gearry RB, Barclay ML, Ferguson LR. 2010. Dietary factors in chronic inflammation: food tolerances and intolerances of a New Zealand Caucasian Crohn’s disease population. Mutation Research/Fundamental and Molecular Mechanisms of Mutagenesis. 690(1-2):123–138.
- van Ommen B, Stierum R. 2002. Nutrigenomics: exploiting systems biology in the nutrition and health arena. Current Opinion in Biotechnology. 13(5):517–521.
- Vermeire S, Satsangi J, Peeters M, Parkes M, Jewell DP, Vlietinck R, Rutgeerts P. 2001. Evidence for inflammatory bowel disease of a susceptibility locus on the X chromosome. Gastroenterology. 120(4):834–840.
- Wong C, Harris PJ, Ferguson LR. 2016. Potential benefits of dietary fibre intervention in inflammatory bowel disease. International Journal of Molecular Sciences. 17(6): 919.
- Zhang JD, Küng E, Boess F, Certa U, Ebeling M. 2015. Pathway reporter genes define molecular phenotypes of human cells. BMC Genomics. 16:342.