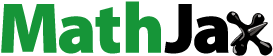
ABSTRACT
Malignant tumour cells, including malignant keratinocytes of oral squamous cell carcinoma (OSCC), exist in conjunction with other functional cells and it is well recognised that interaction between these groups of cells is important in tumour progression. In addition to cells (tumour cells, immune/inflammatory cells, fibroblasts, vascular and lymphatic endothelial cells, adipocytes) the tumour microenvironment (TME) comprises extracellular matrix and soluble factors, signalling molecules and metabolites such as enzymes, growth factors, cytokines, microRNAs and microvesicles. Our Oral Molecular and Immunopathology Research Group has explored and examined the interactions of the immune network involved in the TME such as tumour infiltrating lymphocytes (TILs), regulatory T cells (Tregs), antigen presenting cells (APCs), associated cytokines such as IL17, IL22, IL23 and other current potential anti-cancer targets such as ER stress and exosomes. This paper will provide an overview of the history of OSCC research at the University of Otago, as well as elaborate current understanding of the TME in OSCC, based on ongoing research undertaken in the Oral Molecular and Immunopathology Research Group of the Sir John Walsh Research Institute at the Faculty of Dentistry, University of Otago.
Introduction
Oral squamous cell carcinoma (OSCC) is the most common malignancy in the oral cavity and accounts for more than 90% of oral cancer (Warnakulasuriya Citation2009). The common sites for OSCC are tongue, buccal mucosa, gingiva, floor of the mouth and lip. Gavidi et al. (Citation2014) described 1916 cases of OSCC in New Zealand that had been diagnosed at the Oral Pathology Centre, a specialised oral pathology diagnostic centre at the Faculty of Dentistry, University of Otago, between 2000 and 2010. It was shown that OSCC was more common in men with the mean average age at the time of diagnosis being 63 and tongue being the most prevalent site. Chronic high alcohol intake and tobacco smoking are the most common aetiological agents of OSCC in New Zealand (Yakin et al. Citation2017), while chewing areca wrapped in betel leaf (betel quid) is a frequent cause of OSCC in many Southern Asia countries where OSCC is particularly prevalent. There has been a marked increase in the incidence of oropharyngeal cancer (OPC) over recent years and many of these lesions have been associated with high-risk human papillomavirus (HPV) infection. The oropharynx is the part of the pharynx that comprises the soft palate, pillars of the fauces, palatine tonsils, uvula and posterior one-third or base of the tongue. It is important that carcinoma of this area (OPC) is differentiated from OSCC since they have a different aetiology and prognosis. In contrast to the oropharynx, current evidence for the role of HPV in the development of OSCC is much less certain (Lopes et al. Citation2011; Tauati-Williams Citation2019; Yakin et al. Citation2019a). In this paper, OSCC is defined as squamous cell carcinoma arising in the oral cavity and lip vermilion, excluding the skin of the lips and oropharynx, unless stated otherwise.
Research in OSCC tumour microenvironment (TME) began to intensify at the University of Otago with the establishment of the Oral Molecular and Immunopathology Research Group (OMIRG) in 2007 under the umbrella of Sir John Walsh Research Institute. The OMIRG is based in the Oral Pathology Centre, which also operates New Zealand’s only official Oral Pathology Tissue Bank. Initially, our focus was on the interactions of immune cells and cytokines with OSCC tumour cells. However, this research genre has expanded into various focus areas including angiogenesis, endoplasmic reticulum stress, DNA methylation, cancer-associated fibroblasts and exosomes. The aim of this paper is to showcase recent projects and developments relating to the OSCC TME undertaken in the OMIPRG and to highlight the historical journey that the Faculty has taken in an attempt to become the premier OSCC research centre in New Zealand. This paper also seeks to highlight our contribution to the conceptualisation of the development novel cancer therapies that have the flexibility to cope within the complex dynamics of tumour cells in the TME.
The ‘oral cancer’ scenario in New Zealand
Accurate statistical records for morbidity and mortality of ‘oral cancer’ prior to 1960s are difficult to obtain. In that period, the data were classified under ICD (International Classification of Diseases) coding which lumped cancer of the oral cavity proper with pharyngeal sites and salivary gland tumours. In 1973, the Department of Health reported that there were 167 cases of ‘oral cancer’ (ICD 10, C00-C14) which translated to an age-standardised incidence rate (ASR) of 5.3 per 100,000 population. Following a spike in ‘oral cancer’ cases worldwide prior to the 70s, there was a general consensus of an overall decrease in the incidence of ‘oral cancer’ throughout the Western world (Binnie Citation1976). However, doubt was expressed that this decrease was seen in New Zealand (Ferguson Citation1977). The Ministry of Health published a historical summary showing the ASR of oral cavity, pharyngeal and salivary gland cancer since 1940 where the ASR peaked between 1975 and 1995, with the highest ASR recorded in 1989 (8.3 per 100,000 population) (), and then dropping back to an average ASR of 6.3–6.8 until 2013 (Ministry of Health Citation2016). The latest ASR figures for oral cavity, pharyngeal and salivary gland cancer have risen to 7.7 (2015) and 8.0 (2017) per 100,000 population respectively (Ministry of Health Citation2019). This data demonstrated an apparent significant increase in ‘oral cancer’ incidence back to the peak levels observed in the 80s, but it may actually reflect the increase in OPC noted internationally also occurring in New Zealand rather than an increase in OSCC per se. However, when only lip and oral cavity proper cancers were examined, the incidence was still relatively high. The GLOBOCAN database and the International Agency for Cancer Research (IACR) ranked lip/oral cavity cancer as the 19th most prevalence cancer in New Zealand (Ferlay et al. Citation2018) putting it ahead of ovarian cancer and Hodgkin lymphoma. In 2018, the ASR for lip and oral cavity cancer in New Zealand was 3.6, which was higher than most East Asian countries and close to other developed countries in Europe (ASR 4.1) and the UK (ASR 5.0). This rising trend warrants a concerted effort in raising oral cancer awareness and coordinating early detection strategies similar to those implemented in other developed countries. Reviews have shown that events such as ‘oral cancer awareness week’ had a level of effectiveness in raising cancer awareness among the public (Ghani et al. Citation2013; MacPherson Citation2018). In Malaysia and the UK, establishment of a research foundation or a research coordinating centre has been associated with increased research activities (Zain et al. Citation2013; MacPherson Citation2018). Both of these strategies should be encouraged and explored in New Zealand.
Figure 1. Age-standardised ratio (ASR) for lip, oral cavity, pharyngeal and salivary gland cancer for New Zealand 1960–2015. The figure showed ASR peak between 1975 and 1995, with the highest ASR recorded in 1989 (Ministry of Health Citation2016).
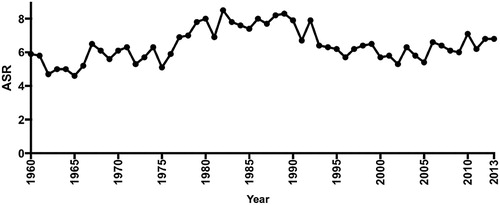
Early OSCC research at University of Otago
The Faculty of Dentistry, University of Otago was founded in 1907 and this consolidated the practice of dentistry in New Zealand to the appropriately trained and qualified professionals rather than barbers, pharmacists, blacksmiths or doctors. During this period, dentistry was very much focused on the practical aspect of caries removal and mechanics of tooth replacement. Even so, oral cancer was already being recognised as one of the major ailments in dentistry. Henry Pickerill, the first Dean of the Faculty, defined OSCC as ‘Epithelioma’ in Chapter XIII of his book ‘Stomatology in Dental Practice’. He went on further to elaborate that ‘a malignancy of squamous-celled variety commonly arises from the oral mucous membrane originating from Leukoplakia, and smoking is said to be the cause’ (Pickerill Citation1912). The terminology of carcinoma was later used in Val MacDonald’s paper in the New Zealand Dental Journal (NZDJ) which described two cases of Leukoplakia transforming into carcinoma (MacDonald Citation1924). In 1946, Frank Schroff established the nation’s first oral pathology diagnostic service at the Faculty of Dentistry, University of Otago. As diagnostic cases accumulated, he started to describe multiple cases of OSCC encountered in New Zealand in several articles complete with histological photomicrographs (Schroff Citation1954).
Most of the academic work on OSCC in New Zealand prior to the 1960s were descriptive studies and observational case reports. During this time, describing clinical presentations of oral cancer cases in the NZDJ was important as the journal was an important source of information for dentists in New Zealand, many of whom worked in single-person practices and some in remote isolated regions throughout the country (MacDonald Citation1924; Schroff Citation1954). It must be remembered that during this period, New Zealand and the rest of the world were recovering from the post-war economic downturn and investment in scientific research was not a priority. Research spaces and laboratories during that time were generally constrained and limited as pointed out by Wilfred Fish in his article entitled ‘The Future of Dentistry’ (Fish Citation1950), where he mentioned ‘we must start to build up dental research. We must look at the dental school for research, and speed up the emergence of results by way of training’. This philosophy was encouraged by the then Dean of the Faculty, Sir John Walsh. After the construction of the then new Walsh Building in 1961, laboratory-based scientific research projects in OSCC began to flourish. Research projects specifically on OSCC started in the early 70s and the first Master of Dental Science thesis on this subject at University of Otago was published in 1977 by John Ferguson entitled ‘Studies on the progression of experimental pre-malignant lesions’ (Ferguson Citation1977) followed by publications related to carcinogenesis, primarily using the hamster cheek pouch model, under the leadership of Professors AC Smillie and MD McMillan. In 1998 Malcolm McMillan delivered his Inaugural Professorial Lecture (IPL) entitled ‘There's more to dentistry than teeth and gums: for instance, oral cancer’ making it the first IPL delivered on oral cancer at the University of Otago (McMillan Citation1988). The choice of his lecture topic could not have been more appropriate and significant at this time, as the oral cavity, pharyngeal and salivary gland cancer incidence was high, prompting the realisation that more research in New Zealand was direly needed. With the advancement of molecular and genetic technologies, research themes relating to the histology and ultra-structure of ‘pre-malignant’ lesions was further expanded in the 80s and 90s resulting several publications and theses from the University (Ferguson et al. Citation1991; Shearer et al. Citation1991, Citation1994, Citation1997; McMillan et al. Citation1994; Symes et al. Citation1995; McMillan and Smillie Citation1996). These publications and theses were essential in describing the histological and ultrastructural changes in the progression of ‘pre-malignant lesions’ to cancer in animal models, and contributed towards our current understanding of how OSCC develops.
By the early millennium, the expansion of genetic research applications has vastly changed the landscape of oral cancer research at Otago and molecular biomarker discovery projects using mRNA detection techniques commenced, example of such research is the detection of Candida albicans mRNA in oral pathology samples by Beggs in early 2000 (Beggs et al. Citation2004). While John Ferguson published a PhD thesis on experimental OSCC in the hamster cheek pouch (Ferguson Citation1985), it was not until 2013 that the first PhD thesis specifically on molecular biomarkers in OSCC TME was published at the University of Otago (Hussaini Citation2013).
International collaborations on oral cancer research at the Faculty started in the late 1960s. Exchange and gifting of tissue and gross specimens, largely from the Institute of Medical Research, Malaysia, can be observed in the archival collection of the Oral Pathology Centre. Over the years there have been numerous visits to the OPC by esteemed international diagnostic and experimental oral pathologists and Faculty oral pathology staff have been able to undertake reciprocal sabbatical visits to their laboratories, greatly assisting research development and exchange of ideas. Today the OMIRG has international collaborative arrangements with the Oral Cancer Research and Coordinating Centre (OCRCC), University of Malaya and MAHSA University, Malaysia through which the group undertakes research projects through access to a major oral cancer tissue bank which has archived tissue from primary OSCC, associated lymph nodes, blood and saliva samples along with clinical follow-up data (Zain et al. Citation2013).
With consolidation of OSCC research into the OMIRG in 2007, we have intensified research in OSCC by looking specifically at OSCC and the TME and further expanded into subspecialised research themes over the subsequent years. The current research themes are broadly categorised into (1) immune cells and cytokines in OSCC, (2) Angiogenic mechanisms in OSCC, (3) Endoplasmic reticulum stress in OSCC, and (4) Exosomes and microvesicles in OSCC. Below are summarised discussions of the research work and advances made within our research group.
The OSCC tumour microenvironment
For many years research into OSCC and potentially malignant oral disorders (PMOD) concentrated on the epithelium alone. However, malignant tumours, including OSCC, exist in conjunction with host cells and it is well recognised that interaction between these components is important in tumour development and progression. In addition to cells (tumour cells, immune/inflammatory cells, fibroblasts, vascular and lymphatic endothelial cells, adipocytes), the TME comprises extracellular matrix (ECM) and soluble factors, signalling molecules and metabolites such as enzymes, growth factors, cytokines, microRNAs and microvesicles. In the 2000s we were interested in the network of immune cells and cytokines and how they interacted which each other in the TME. Little was known back then on the mechanisms of how OSCC progresses from a dysplastic lesion to ultimately metastasise to regional lymph nodes.
Tumour infiltrating lymphocytes (TILs) and regulatory T cells (Tregs) in OSCC TME
Malignant tumours, including OSCC, are frequently infiltrated by variable numbers of mononuclear immune cells, mostly lymphocytes, known as TILs (Wanebo Citation1979; Rollins Citation2006; Fang et al. Citation2017). This phenomenon was initially thought to be evidence of a host response to eliminate cancer cells (Yu and Fu Citation2006). However, a growing body of evidence suggests that TILs may, in fact, facilitate the escape of tumours cells from the immune system. Results from studies on the prognostic implication of TILs, particularly in OSCC, have shown conflicting evidence with several authors finding that increased numbers of TILs were associated with survival benefits (Wolf et al. Citation1986; Brandwein-Gensler et al. Citation2005), whereas others showed no significant correlation (Guo et al. Citation1987; Rajjoub et al. Citation2007). Given these equivocal results, Uppaluri et al. (Citation2008) concluded that there was little value in using the number of TILs to determine the prognosis of OSCC. However, more recent work has suggested that TILs do play a significant role in OSCC and can also predict the survival outcome (Fang et al. Citation2017). In malignant melanoma, where TILs have been shown to play a significant role in predicting the outcome, it was apparent that the prognostic value of TILs depends on the TIL subsets present rather than the overall number of TILs (Oble et al. Citation2009). It is interesting to note that an increased population of CD4+ cells among TILs in OSCC (Snyderman et al. Citation1991) was observed, before the ‘re-discovery’ of Tregs in 1995 (Sakaguchi et al. Citation1995). The increased number of CD4+ cells related particularly to CD4+ Tregs and was found to be due to a suppressive microenvironment in the stroma of OSCC, induced by depression of natural killer (NK) cells and CD8+ cell activity (Whiteside Citation2006; Strauss et al. Citation2009).
Histological grading systems used as an adjunct to staging systems to define prognosis and treatment strategies for oral cancer have moved from concentrating just on the morphology and arrangement of the malignant keratinocytes themselves to include features in the adjacent tumour stroma, particularly at the invasive front, such as intensity of the lymphocyte host response (LHR) (Bryne Citation1998; Brandwein-Gensler et al. Citation2010). LHR is assessed at the infiltrating front of the tumour and, depending on the presence of collections of lymphocytes (lymphoid nodules) is classified as strong, intermediate or limited (Maleki et al. Citation2011). More interface CD8+ T cells were present in lesions with strong LHR, with strong LHR being inversely correlated with disease progression (Zancope et al. Citation2010; Maleki et al. Citation2011). Potentially working against CD8+ T cells are Tregs, a subpopulation of CD4+ T cells that are immunosuppressive and can down-regulate the induction and proliferation of other effector cells and thus inhibit the effectiveness of the immune system (O’Higgins et al. Citation2018). Using formalin-fixed paraffin-embedded (FFPE) tissue from 25 cases of OSCC the distribution and type of immune cells, namely CD3+ , CD4+ (T helper cells [Th]), CD8+ (cytotoxic T cells [Tc]), CD56+ (NK cells) and CD68+ cells within the primary OSCC microenvironment was determined in our laboratory using immunohistochemistry (IHC). Qualitative and semi-quantitative analyses showed that there were reduced numbers of NK cells and Tc cells in OSCC compared with inflammatory controls (Hussaini Citation2013) (). Our group has also shown that there are more CD25+ FoxP3+ Tregs in the stroma of OSCC by comparison with inflammatory controls () (Hussaini Citation2013). This may contribute to the anti-tumour response, allowing tumour progression, although the role of Tregs in carcinogenesis is complex.
Figure 2. Showing immunhistochemistry (IHC) staining with 3,3′-Diaminobenzidine (DAB) chromogen of selected antibodies (brown staining). (A) OSCC group: CD8+ cells were sparsely distributed in the deeper connective tissue (x200, bar: 50 m). (B) OSCC group: Moderate numbers of CD8+ cells around strands and islands of malignant keratinocytes (x200, bar: 50 m). (C) Control group: The frequency and intensity of staining was more than in OSCC sections with focal collections of CD8+ cells in the control tissues in comparison to the sparse distribution in OSCC sections (x200, bar: 50 m).
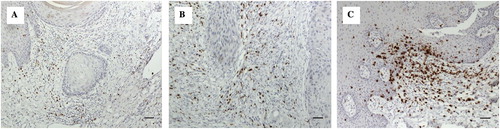
Figure 3. Box and whisker plot showing significantly more T regs in the TME of OSCC than inflamed control tissue. IHC staining was used to stained T regs positive cells using FoxP3 antibody and DAB chromogen.
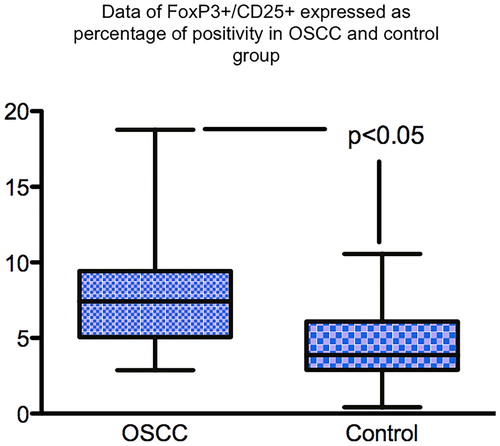
The interest in gaining a greater understand of the composition and functional activity of the stromal immune cells in OSCC is related to the potential to expand the field of cancer immunotherapy to this region, using the experience from other solid tumours as a platform e.g. the use of antibodies against T cell antigens such as ipilimumab for some melanomas (Wado-Ohno et al. Citation2019). The management of primary oral cancer currently remains centred around surgery with or without radiotherapy. Both modalities are associated with significant morbidity even when the treatment is curative and so it is important to search for effective durable targeted medical management for oral cancer, as has been used successfully in cancers of other systems. General systemic chemotherapy with the likes of 5-fluorouracil (FU) and bleomycin were used for advanced and recurrent head and neck squamous cell carcinoma (HNSCC) with some success and later combination therapies e.g. 5FU/cisplatin were employed, but these regimens also had many side effects (Blasco et al. Citation2017) and were often followed by recurrence of the cancer and resistance (Derbal Citation2018). The epithelial growth factor receptor inhibitor cetuximab was the first targeted therapy approved by the Food and Drug Administration (FDA) for use in HNSCC and it is now the standard of care for metastatic HNSCC in some centres (Kaidar-Person et al. Citation2018). Subsequently, with increased understanding of the role immune cells play in the TME and greater understanding of immune escape, where tumour cells evade the immune system, other drugs known as immune checkpoint inhibitors have been approved, after obtaining good results in clinical trials (Blasco et al. Citation2017). Currently, immune checkpoint inhibitors that are available in New Zealand are nivolumab (PD1 inhibitor) and ipilimumab (CTLA-4 inhibitor). Only nivolumab is publicly funded through Pharmac (Pharmaceutical Management Agency, Ministry of Health, New Zealand) and available for use in OSCC patients.
Macrophages, dendritic cells and antigen presenting cells (APCs)
Discovered by Mechnikoff in the early 1900s, the macrophage was named a phagocyte due to its ability to ‘devour’. Apart from being well-known phagocytes, macrophages and DC are a crucial group of APCs. Their ability to phagocytose and process microbial agents, foreign antigens, cell debris and dying cells, process and present protein antigens to naïve lymphocytes in secondary lymphoid organs is crucial to immune homeostasis and allowed them to be characterised as APCs (Male et al. Citation2006). This role is important in order to activate differentiation and clonal expansion of T cells (Bluestone et al. Citation2009). Therefore, APCs have a significant transitional or bridging role between innate and adaptive immunity. APCs respond to a wide variety of non-specific inflammatory mediators or to stress signals produced by cancer cells through membrane bound or vesicular Pattern Recognition Receptors (PRRs) such as Heat Shock Proteins (HSPs), and TLRs (Toll-Like Receptors) (Duffield Citation2003). Upon encountering foreign antigen or tumour cells, they will phagocytose protein or tumour antigens and then proceed to secondary lymphoid organs for antigen presentation. While in the lymph nodes, antigen presentation to lymphocytes occurs and produces clonal expansion of CD4+ and CD8+ T cells, as well as the recently discovered subsets of Th17 and Tregs (McCluskey Citation2011). Through interleukin (IL)-12, CD80 and other co-signalling molecules, priming of naïve T cells occurs enabling them to become specific cytotoxic T cells (CD8+ CTLs). These cells then enter the circulation where they will recognise and destroy target cells. In tumours, macrophages are known as tumour-associated macrophages (TAMs), first discovered by Mantovani et al. (Citation1986). The role of TAMs has been unclear, however most reports acknowledge that TAMs enhance the progression of tumours and can inhibit adaptive immune responses to cancer (Allavena et al. Citation2008). The presence of TAMs was associated with poor prognosis in multivariate analyses of several cancers (Ho et al. Citation2008; Zhu et al. Citation2008). They are recruited to the region of the tumour in response to the secretion of interferon (IFN)γ where, depending on the cytokines present, can be induced to differentiate into: (1) M1 type of macrophages which express IL-12 and tumour necrosis factor (TNF) supporting a Th1 response or (2) M2 type of macrophages which secrete IL-10 and IL-4 supporting a Th2 response (Martinez et al. Citation2008; Pinto et al. Citation2019). Most TAMs observed in the cancer environment resemble M2-polarised macrophages (Mantovani et al. Citation2004; Mosser and Edwards Citation2008) and instead of being induced by IFNγ, they are associated with Th2 cytokines, such as IL-4 and IL-10 (Cassetta and Pollard Citation2018). It is not clear as to the regulatory mechanism which governs the polarisation of these macrophages, particularly in the OSCC TME. As mentioned earlier, our research group has previously assessed macrophages in OSCC tissues and shown that there were more CD68+ macrophages in OSCC compared to inflammatory control tissue (Hussaini Citation2013).
With the advances in cancer treatment using immunotherapies, research focus has shifted from TAMs and become slanted towards suppressing the functions of Treg, T cell inhibitory receptors (immune checkpoints) and chimeric antigen T cell receptors (CAR-T). Reduction in cancer burden and success with these immune checkpoint therapies relies largely on their ability to induce the activation and recruitment of tumour reactive-cytotoxic T cells (Wei et al. Citation2018). However, not every patient will benefit from immune checkpoint therapy, particularly those who have shown resistance to immunotherapies. Current research has shown that resistance to immune checkpoint can be overcome with increased tumour-associated myeloid cells such a macrophages, granulocytes and monocytes (De Henau et al. Citation2016; Pathria et al. Citation2019). Therapies that induce the recruitment of tumour-associated myeloid cells such as TLR agonists and signal transducer and activator of transcription (STAT)-3 inhibitors are currently being investigated for potential clinical use (Pathria et al. Citation2019).
Major immune cells and cytokines in OSCC
How Tregs modulate the immune system is not fully understood but one way might be through TLR. TLR were first recognised on the surface of inflammatory cells, especially macrophages where they alert the body to external pathogens, particularly bacteria, and set off an immune response, but it is now well known that they recognise endogenous damage as well. Danger signals arising from injured or altered cells are known as damage–associated molecular patterns (DAMPS) and these are recognised by certain TLRs. Perhaps cancer cells and/or potentially malignant cells release DAMPS? Our group assessed 50 cases each of inflamed irritative hyperplasia (IH), epithelial dysplasia (ED) and OSCC and found that more inflammatory cells expressed TLR2 in the stroma of OSCC than in hyperplastic tissue (A,B). No hyperplastic samples showed TLR2+ keratinocytes but keratinocytes in 64% of cases of OSCC were TLR2+. Positive TLR2 expression in the TME suggests that immune surveillance is activated against the altered epithelial cells while TLR2 expression by malignant keratinocytes may correlate with apoptosis resistance and hence the survival of tumour cells (Ng et al. Citation2011). Double immunofluoresence studies showed that TLR2+FoxP3+ Tregs were present in the OSCC microenvironment () with apparent cell-to-cell contact between TLR2+ and FoxP3+ cells. The presence of FoxP3+TLR2+ cells may represent a dendritic cell-dependent pathway capable of inhibiting Treg suppressive activity, potentially beneficial to the anti-tumour response (Hussaini et al. Citation2017).
Figure 4. Photomicrographs showing the expression of TLR2 in the stroma of inflammatory hyperplasia. A, and OSCC B. Expression of TLR2 was significantly more in OSCC tissues in comparison to control group (inflamed mucosa). IHC staining was used to stained TLR2 positive cells using TLR2 antibody and DAB chromogen.
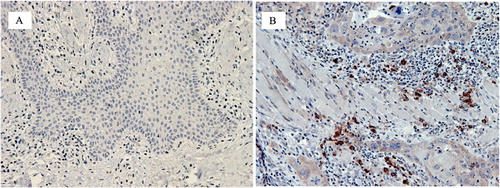
Figure 5. Photomicrograph showing co-expression of FoxP3 (red nuclear fluoresence) and TLR2 (green ring fluoresence) in the stromal cell in OSCC (cell on the lower right). Double immunofluoresence technique was used for staining of FoxP3 and TLR2 positive cells using Alexa red and green chromogen.
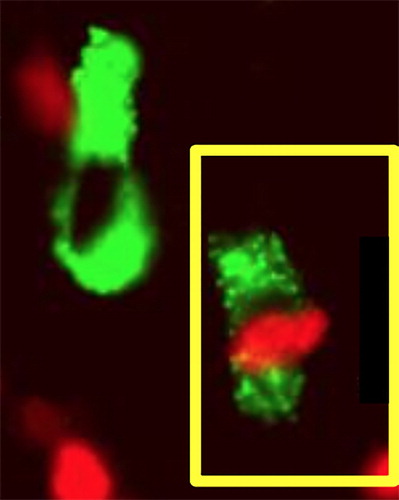
Interleukin 17 is a pro-inflammatory cytokine with both pro- and anti-tumour effects which has been shown to have increased expression in some cancers. Its pro-tumour effects are mediated by mechanisms including inducing the expression of matrix metalloproteinases (MMPs) in tumour cells and/or stimulating increased tumour angiogenesis. The anti-tumour effects of IL17 are exerted through increased Tc cells and IFNγ activity. Our studies showed significantly more IL17+ cells were present in the TME of OSCC than inflammatory controls (Avadhani Citation2015). Double-labelling immunofluorescence studies revealed that Th cells, Tc cells, macrophages and mast cells co-expressed IL17 (Avadhani et al. Citation2017). Using an extracellular matrix (ECM) cell invasion assay kit with invasion assay chambers we found that IL17 significantly promoted the in vitro invasion of OSCC cell lines (Avadhani Citation2015). These results are consistent with the observations in other cancers where IL17 was found to facilitate tumour progression e.g. breast cancer cell lines, cervical cancer cell lines (Cochaud et al. Citation2013; Punt et al. Citation2015).
Using cell culture with three immortal OSCC cell lines (SCC4, SCC15 and SCC25) obtained from the Cell Culture Core collection (Harvard Skin Disease Research Centre Culture and Microscopy Core, USA) Genes associated with tumour metastasis were differentially expressed in OSCC cells treated with IL17; mRNA expression of MMP2, MMP10 and MMP13 were up-regulated in OSCC cells lines hence we suggest that IL17 facilitates tumour progression by differential expression of genes associated with tumour metastasis, particularly those associated with ECM proteins () (Avadhani et al. Citation2015).
Table 1. MMP genes which coded for enzymes that degrade ECM proteins were significantly upregulated in OSCC cell lines treated with IL-17.
We then moved on to examine T cell anergy and immune tolerance gene expression pattern in tissue from primary OSCC tissues and metastatic lymph nodes with the hypothesis that tumour escape mechanisms seen at the local tumour site may also occur in regional cervical lymph nodes (CLN). For this investigation, we acquired CLNs from Malaysia as a research collaborative effort with the OCRCC. Pre-metastatic niche (PMN) is a new concept in the process of metastasis and is defined as the development of a TME at a future metastatic site which is established by the tumour in anticipation of the arrival of disseminated tumour cells since the cells will need a permissive environment to thrive (Peinado et al. Citation2017; Doglioni et al. Citation2019). Certain cells, especially pro-tumour immune cells such as neutrophils and macrophages, and cytokines have been reported to be key factors in building these niches (Doglioni et al. Citation2019). We investigated the IHC expression of IL17, IL22, IL23 and STAT3 by stromal cells within CLN without metastasis (n = 18) and CLN with histologically detected metastases (n = 18) in patients with primary OSCC obtained from the OCRCC. The expression of IL22, IL23 and STAT3 was significantly higher in the negative lymph nodes when compared with the positive group. This may be related to the fact that disseminating tumour cells are vulnerable and their only chance of surviving and setting up a metastasis is by suppressing the local immunity of the PMN to its maximum (Alkharusi Citation2017). We suggest that immune suppression is inversely related to the ability of signals from the tumour to manipulate the PMN environment (Alkharusi Citation2017). Metastasis is a complex disease regulated by many mediators, but potentially IL22, IL23 and STAT3 could be included in a panel to assess CLN to diagnose early signs of OSCC node metastasis. This could be part of the immune assessment of sentinel LNs, bearing in mind that IHC is widely available and cost effective. PMN modelling suggests that patients will benefit from early intervention targeting PMN formation, especially in malignancies like OSCC that have high tendency to metastasise. For example, inhibition of VEGRF suppressed PMN formation in preclinical trials in patients with liver cancer (Yamamoto et al. Citation2008).
Angiogenic mechanisms in OSCC
A typical feature of neoplasia is the secretion of angiogenic factors via a complex balance of pro- and anti-angiogenic mechanisms (Folkman and Shing Citation1992). It is known that endothelial cells in the TME participate in cross talk with tumour cells themselves and promote invasion. In a study in our laboratory angiogenic factors (vascular endothelial growth factor [VEGF], VEGF receptor 2 [KDR] and vasohibin-1 [VASH-1]) were expressed differently in the endothelial cells and the epithelial cells of inflamed hyperplastic oral tissues compared with OSCC and normal oral mucosa (NOM) (Allsobrook Citation2014). The malignant keratinocytes of OSCC were strongly VEGF+. Gene expression in three immortal OSCC cell lines (SCC4, SCC15 and SCC25) and three normal epithelial cell lines (OKF4, OKF6 and OKP7) showed differential regulation of VEGF in OSCC compared with normal epithelial cells (Allsobrook Citation2014). Likewise, lymphangiogenesis is a key component of carcinogenesis with loss of control of lymphangiogenic regulation leading to the formation of new lymph vessels within and around the tumour. This may increase the likelihood of lymphatic metastasis. Using IHC with reliable lymphatic endothelial cell (LEC) antibody markers, we were able to establish that the OSCC TME possessed significantly more lymphatic vessels expressing D2-40 () podoplanin (D2-40) and prospero-homeobox protein (Prox)-1 than the control groups (Chutipongpisit Citation2016). Overexpression of the lymphatic markers in the malignant tissues was significantly greater than in the inflamed connective tissue as well as the normal controls, i.e. increased lymphangiogenesis associated with non-specific inflammation was controlled for. This increase in lymphatic vessels density (LVD) may play a role in facilitating lymphatic invasion and later metastases. These molecular entities may serve as potential anti-oral cancer therapeutic targets. D2-40 expression on the tumour cells themselves has been associated with a role in tumour progression in experimental and human carcinogenesis (Schacht et al. Citation2005; Ugorski et al. Citation2016) including in oral cancer (Martin-Villar et al. Citation2005; Yuan et al. Citation2006; Cueni et al. Citation2010; Tsuneki et al. Citation2013) and OPMD (Kawaguchi et al. Citation2008; Mei et al. Citation2014). In OPMD, the basal cell layer of the dysplastic epithelium was D2-40+ (Kawaguchi et al. Citation2008), a phenomenon also observed in our study in dysplastic epithelium adjacent to OSCC (Chutipongpisit Citation2016). Multivariate analysis found that epithelial D2-40 positivity was an independent predictor of progression of OPMD to OSCC and that it can be used to further stratify oral cancer development risk (Kawaguchi et al. Citation2008).
Figure 6. Box and whisker plot showing a significantly greater lymphatic vessel density (using D2-40/podoplanin) in the TME of OSCC than ininflamed and uninflamed control tissue. IHC staining was used to stained D2-40 positive cells using D2-40/podoplanin antibody and DABchromogen (*indicates p<0.05, o indicates an outlier value).
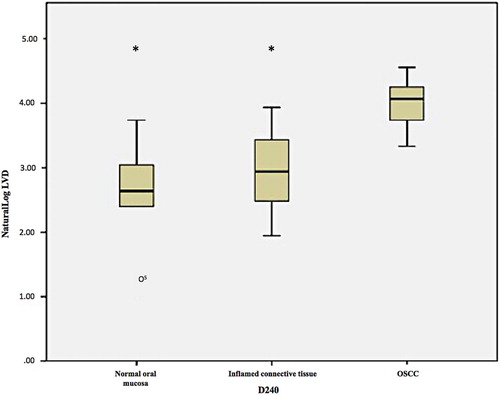
For another project, two groups of FFPE blocks were accessioned from the OCRCC, Malaysia; Group A comprised CLN with histologically confirmed metastatic deposits from primary OSCC (n = 17) and Group B were CLN from patients with primary OSCC without metastatic deposits, (n = 17). IHC showed significantly greater VEGF-C (another marker associated with lymphangiogenesis) expression in Group A compared with Group B (p = .0002) (Ibrahim Citation2017). In this study, the actual OSCC deposits in the nodes showed no VEGFC staining. It is suggested that the active VEGFC-secreting cells in these nodes were in the surrounding stroma rather than the keratinocytes themselves. However, there must be cross-talk between the metastatic OSCC cells and the surrounding microenvironment prior increased VEGFC production. Significant positive correlation was found between VEGFC expression in CLN and TNM stage (p = .004) (Ibrahim Citation2017).
Endoplasmic reticulum stress in OSCC
A further line of enquiry has been examining the effects of intrinsic and extrinsic stressful stimuli on the TME, specifically endoplasmic reticulum (ER) stress. ER stress leads to the activation of the unfolded protein response (UPR). This is an evolutionarily conserved set of mechanisms designed to ameliorate ER homeostatic imbalance or to induce cell death through apoptosis when ER stress cannot be mitigated. The UPR is extensively intertwined with other vital processes, including those that influence development and progression of cancer. As such, UPR has been implicated in either driving lesional dormancy or promotion and progression in multiple cancer types in a context-dependent manner, and it has been experimentally exploited as potential anti-cancer targets (Shen et al. Citation2018; Hsu et al. Citation2019). However, at this stage, the understanding of the role of ER stress-induced UPR in the pathogenesis and progression of OSCC is still in its infancy, requiring further elucidation. We undertook a series of investigations examining ER stress and the maintenance of OSCC cell viability and apoptosis and the differential regulation of key UPR and ER stress-associated genes in an established in-vitro model using the potent inducer of ER stress, tunicamycin. Seven extensively substantiated cell lines derived from normal, dysplastic and malignant oral keratinocytes were used. They were subjected to tunicamycin-induced ER stress of varying intensity and chronicity. In the cell viability experiments it was demonstrated that OSCC cells maintained cell viability in the presence of ER stress at a significantly greater level, compared to normal oral keratinocytes (Seo Citation2019). Furthermore, caspase-3/7 activity and DNA fragmentation, hallmarks of cell death, were suppressed in OSCC (Seo Citation2019) (A,B). It was also discovered, for the first time, that UPR-induced apoptosis-related factors, most notably DNA damage inducible transcript (DDIT)-3, were significantly up-regulated in OSCC. Also, the master regulator of lipid metabolism, sterol regulatory binding factor (SREBP)-1, and CAMP responsive element binding protein 3-Like (CREB3L)-3, an ER-resident transcription factor closely related to activating transcription factor (ATF)-6, which plays an important role in linking ER stress with immune–inflammatory responses, were significantly up-regulated in OSCC (Seo Citation2019). These studies highlighted the importance of, and the influence of ER stress and UPR, on the pathogenesis and pathobiology of OSCC, especially centred around factors that influence apoptosis, TME and lipid metabolism.
Figure 7. A&B: A, Graph showing SCC4 cell line subjected to tunicamycin-induced ER stress (test and control). B, Photomicrograph SCC cell line subjected to tunicamycin-induced ER stress with TUNEL staining. Blue = DAPI, Green = TUNEL. SCC4 cell line maintained cell viability in the presence of ER stress at a significantly greater level.
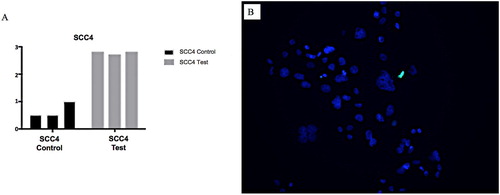
We previously showed the involvement STAT3 pathways in many malignancies including OSCC (Alkharusi Citation2017) before continuing to investigate the expression of STAT3 pathway-related genes and proteins under ER stress in OSCC. Our investigation showed that IL5 (p = .005) and IL22 (p = .024) were upregulated in OSCC cell lines (Yakin et al. Citation2019b). Greater STAT3 (p = .019) and leukaemia inhibitory factor receptor (p = .042) protein expression were observed in tunicamycin-treated than untreated NOK cell lines (Yakin et al. Citation2019b). The gene and protein regulation patterns showed that ERS played a role in modifying the TME in OSCC by up-regulating tumour-promoting cytokines.
Exosomes and OSCC
Our most recent studies relate to exosomes, extracellular microvesicles that are released by a range of cells into the extracellular environment. They are present in numerous bodily fluids including blood and saliva. Exosomes were thought to carry waste or unwanted lipids and proteins from the cells but it was found that they also contain DNA and RNA which could be translated into proteins in target cells, potentially reprogramming them (Valadi et al. Citation2007). Tumour cells have been shown to produce and secrete exosomes in greater numbers than normal cells and it is now clear that exosomes have important roles in cancer, including promoting or suppressing immune activities around malignant cells and exchange of information between tumour cells and their microenvironment (Wendler et al. Citation2013; Ruivo et al. Citation2017). They have been shown to be important in many of the major steps in carcinogenesis particularly in assisting tumour cells escape from immune surveillance, tumour-promoting effects in the TME and promoting the establishment of metastases, including contributing towards establishing PMN (Meehan and Vella Citation2016; Ruivo et al. Citation2017; Guo et al. Citation2019). We have been able to extract exosomes from OSCC cells grown in culture using ultracentrifugation and an exosome isolation kit (Exoquick TC plus) and characterise them using a Zetasizer Nano® (Aziz Citation2019, unpublished data) (). Using archival blood and saliva samples from the OCRCC we are now undertaking quantitative mRNA and protein analyses of oral cancer-related genes and proteins within exosomes isolated from plasma and saliva samples of healthy individuals and patients with OSCC. This part of the study will provide further information regarding the potential use of exosomes in the liquid diagnosis of OSCC.
Conclusion
In conclusion, the Otago effort in the examination of a range of factors that influence the TME locally and in relation to potential metastases has revealed several mechanisms that may contribute to the development and progression of OSCC. Some of these mechanisms may prove to be suitable targets for innovative drug therapies. Examples include the preclinical work we have published relating to Tregs, TLRs, IL17 and STAT3 which may contribute to the development of clinically relevant immunotherapies. It is important that this line of research into OSCC continues to flourish in New Zealand through the support of the University and the community with the ultimate goal of improving the health and lives of New Zealanders. We acknowledge the research funding we have received over the years, particularly the support received from the New Zealand Dental Research Foundation.
Disclosure statement
No potential conflict of interest was reported by the author(s).
Additional information
Funding
References
- Alkharusi A. 2017. Lymph nodes as a pre-metastatic niche for oral squamous cell carcinoma [PhD dissertation]. University of Otago.
- Allavena P, Sica A, Solinas G, Porta C, Mantovani A. 2008. The inflammatory micro-environment in tumor progression: the role of tumor-associated macrophages. Crit Rev Oncol Hematol. 66(1):1–9.
- Allsobrook OFL. 2014. Angiogenic factors in oral squamous cell carcinoma [DClinDent thesis]. University of Otago.
- Avadhani A. 2015. Role of interleukin 17 in progression of oral squamous cell carcinoma [PhD dissertation]. University of Otago.
- Avadhani AV, Milne T, Seymour GJ, Rich AM. 2015. Interleukin-17 induces matrix metalloproteinase activation and invasion in oral cancer. J Dent Res. 94:2331200.
- Avadhani A, Parachuru VPB, Milne T, Seymour GJ, Rich AM. 2017. Multiple cells express interleukin 17 in oral squamous cell carcinoma. J Oral Pathol Med. 46(1):39–45.
- Aziz M. 2019. Exosomes in oral cancer [unpublished PhD dissertation]. University of Otago.
- Beggs KT, Holmes AR, Cannon RD, Rich AM. 2004. Detection of Candida albicans mRNA in archival histopathology samples by reverse transcription-PCR. J Clin Microbiol. 42(5):2275–2278.
- Binnie WH. 1976. Epidemiology and etiology of oral cancer in Britain. Proc R Soc Med. 69(10):737–740.
- Blasco MA, Svider PF, Raza SN, Jacobs JR, Folbe AJ, Saraf P, Eloy JA, Barades S, Fribley AM. 2017. Systemic therapy for head and neck squamous cell carcinoma: historical perspectives and recent breakthroughs. Laryngoscope. 127(11):2565–2569.
- Bluestone JA, Mackay CR, O’Shea JJ, Stockinger B. 2009. The functional plasticity of T cell subsets. Nat Rev Immunol. 9(11):811–816.
- Brandwein-Gensler M, Smith RV, Wang B, Penner C, Theilken A, Broughel D, Schiff B, Owen RP, Smith J, Sarta C, et al. 2010. Validation of the histologic risk in a new cohort of patients with head and neck squamous cell carcinoma. Am J Surg Pathol. 34(5):676–688.
- Brandwein-Gensler M, Teixeira MS, Lewis CM, Lee B, Rolnitzky L, Hille JJ, Genden E, Urken ML, Wang BY. 2005. Oral squamous cell carcinoma: histologic risk assessment, but not margin status, is strongly predictive of local disease-free and overall survival. Am J Surg Pathol. 29(2):167–178.
- Bryne M. 1998. Is the invasive front of an oral carcinoma the most important area for prognostication? Oral Dis. 4(2):70–77.
- Cassetta L, Pollard JW. 2018. Targeting macrophages: therapeutic approaches in cancer. Nat Rev Drug Discov. 17(12):887–904.
- Chutipongpisit K. 2016. Lymphangiogenesis in oral squamous cell carcinoma [DClinDent thesis]. University of Otago.
- Cochaud S, Giustiniani J, Thomas C, Laprevotte E, Garbar C, Savoye M, Cure H, Mascaux C, Alberici G, Bonnefoy N, et al. 2013. IL-17A is produced by breast cancer TILs and promotes chemoresistance and proliferation through ERK1/2. Sci Rep. 3:3456.
- Cueni LN, Hegyi I, Shin JW, Albinger-Hegyi A, Gruber S, Kunstfeld R, Moch H, Detmar M. 2010. Tumor lymphangiogenesis and metastasis to lymph nodes induced by cancer cell expression of podoplanin. Am J Pathol. 177(2):1004–1016.
- De Henau O, Rausch M, Winkler D, Campesato LF, Liu C, Cymerman DH, Budhu S, Ghosh A, Pink M, Tchaicha J, et al. 2016. Overcoming resistance to checkpoint blockade therapy by targeting PI3Kgamma in myeloid cells. Nature. 539(7629):443–447.
- Derbal Y. 2018. The adaptive complexity of cancer. Biomed Res Int. DOI:10.1155/2018/5837235.
- Doglioni G, Parik S, Fendt S-M. 2019. Interactions in the (pre) metastatic niche support metastasis formation. Front Oncol. DOI:10.3389/fonc.2019.00219.
- Duffield J. 2003. The inflammatory macrophage: a story of Jekyll and Hyde. Clin. Sci. 104(1):27–38.
- Fang J, Li X, Ma D, Liu X, Chen Y, Wang Y, Lui VWY, Xia J, Cheng B, Wang Z. 2017. Prognostic significance of tumor infiltrating immune cells in oral squamous cell carcinoma. BMC Cancer. 17(1):375.
- Ferguson JW. 1977. Studies on the progression of experimental pre-malignant lesions [Masters of Dental Surgery thesis]. University of Otago.
- Ferguson JW. 1985. A light and transmission electron microscope study of normal mucosa and chemically induced premalignant and malignant lesions in hamster cheek pouch [PhD dissertation]. University of Otago.
- Ferguson JW, McMillan MD, Smillie AC. 1991. Ultrastructural examination of experimentally induced premalignant lesions. Int J Oral Maxillofac Surg. 20(2):112–118.
- Ferlay J, Ervik M, Lam F, Colombet M, Mery L, Piñeros M, Znaor A, Soerjomataram I, Bray F. 2018. Global cancer observatory: cancer today. Lyon: International Agency for Research on Cancer. https://gco.iarc.fr/today.
- Fish WD. 1950. The future of dentistry. New Zeal Dent J. 40:144.
- Folkman J, Shing Y. 1992. Angiogenesis. J Biol Chem. 267(16):10931–10934.
- Gavidi RO, Rich AM, Cox B, King T. 2014. Comparing the occurrence of oral squamous cell carcinoma in New Zealand and the Fiji islands from 2000–2010. Int J Cancer Res. 48(1):1212–1225.
- Ghani WMN, Doss JG, Jamaluddin M, Kamaruzaman D, Zain RB. 2013. Oral cancer awareness and its determinants among a selected Malaysian population. Asian Pacific J Cancer Prevent. 14(3):1957–1963.
- Guo Y, Ji X, Liu J, Fan D, Zhou Q, Chen C, Wang W, Wang G, Wang H, Yuan W, et al. 2019. Effects of exosomes on pre-metastatic niche formation in tumors. Mol Cancer. 18(1):39.
- Guo M, Rabin BS, Johnson JT, Paradis IL. 1987. Lymphocyte phenotypes at tumor margins in patients with head and neck cancer. Head Neck Surg. 9(5):265–271.
- Ho C-C, Liao W-Y, Wang C-Y, Lu Y-H, Huang H-Y, Chen H-Y, Chan W-K, Chen H-W, Yang P-C. 2008. TREM-1 expression in tumor-associated macrophages and clinical outcome in lung cancer. Am J Respir Crit Care Med. 177(7):763–770.
- Hsu S, Chiu C, Dahms H, Chou C, Cheng C, Chang W, Cheng K, Wang H, Lin I. 2019. Unfolded protein response (UPR) in survival, dormancy, immunosuppression, metastasis, and treatments of cancer cells. Int J Mol Sci. 20(10):2518.
- Hussaini HM. 2013. Immune responses in primary oral squamous cell carcinoma and lymph node metastasis [PhD dissertation]. University of Otago.
- Hussaini HM, Parachuru VPB, Seymour GJ, Rich AM. 2017. Forkhead box-P3+ regulatory T cells and toll-like receptor 2 co-expression in oral squamous cell carcinoma. Acta Histochem. 119(3):205–210.
- Ibrahim NR. 2017. Lymphangiogenesis in metastatic lymph nodes of oral squamous cell carcinoma [DClinDent Dissertation]. University of Otago.
- Kaidar-Person O, Gil Z, Billan S. 2018. Precision medicine in head and neck cancer. Drug Resist Updates. DOI:10.1016/j.drup.2018.09.001.
- Kawaguchi H, El-Naggar AK, Papadimitrakopoulou V, Ren H, Fan Y-H, Feng L, Lee JJ, Kim E, Hong WK, Lippman SM, Mao L. 2008. Podoplanin: A novel marker for oral cancer risk in patients with oral premalignancy. J Clin Oncol. 26(3):354–360.
- Lopes V, Murray P, Williams H, Woodman C, Watkinson J, Robinson M. 2011. Squamous cell carcinoma of the oral cavity rarely harbours oncogenic human papillomavirus. Oral Oncol. 47(8):698–701.
- MacDonald VP. 1924. The mouth in health and disease. New Zeal Dent J. 20:155.
- MacPherson LMD. 2018. Raising awareness of oral cancer from a public and health professional perspective. Br Dent J. 225(9):809–814.
- Male D, Brostoff J, Roth D, Roitt I. 2006. Immunology. 7th ed. Orlando (FL): Mosby. p. 145–161.
- Maleki S, Schlecht NF, Keller G, Diaz J, Moss J, Prystowsky MB, Macian F, Brandwein-Gensler M. 2011. Lymphocytic host response to oral squamous cell carcinoma: an adaptive T-cell response at the tumor interface. Head Neck Patho.l. 5(2):117–122.
- Mantovani A, Ming WJ, Balotta C, Abdeljalil B, Bottazzi B. 1986. Origin and regulation of tumor-associated macrophages: the role of tumor-derived chemotactic factor. Biochim Biophys Acta. 865(1):59–67.
- Mantovani A, Sica A, Sozzani S, Allavena P, Vecchi A, Locati M. 2004. The chemokine system in diverse forms of macrophage activation and polarization. Trends Immunol. 25(12):677–686.
- Martin-Villar E, Scholl FG, Gamallo C, Yurita MM, Munoz-Guerra M, Cruces J, Quintanilla M. 2005. Characterization of human PA2.26 antigen (T1alpha-2, podoplanin), a small membrance mucin induced in oral squamous cell carcinomas. Int J Cancer. 113(6):899–910.
- Martinez FO, Sica A, Mantovani A, Locati M. 2008. Macrophage activation and polarization. Front Biosci. 13:453–461.
- McCluskey J. 2011. Update on T-cell immunity: Implications for tumor immunology. Asia Pac J Clin Oncol. 7:3–8.
- McMillan MD. 1988. There's more to dentistry than teeth and gums: for instance, oral cancer. Inaugural Professorial Lecture (IPL). Hocken Library, University of Otago.
- McMillan MD, Smillie AC. 1996. Scanning electron microscopy of dimethylbenz-anthracene-treated hamster cheek pouch. J Oral Pathol Med. 25(1):14–19.
- McMillan MD, Smith CJ, Ramirez V. 1994. The effect of intraperitoneal N-methyl-N-nitrosurea on hamster palatal mucosa. Arch Oral Biol. 39(6):519–528.
- Meehan K, Vella LJ. 2016. The contribution of tumour-derived exosoms to the hallmarks of cancer. Crit Rev Clin Lab Sci. 53(2):121–131.
- Mei Y, Zhang P, Zuo H, Clark D, Xia R, Li J, Liu Z, Mao L. 2014. Ebp1 activates podoplanin expression and contributes to oral tumorigenesis. Oncogene. 33(29):3839–3850.
- Ministry of Health New Zealand. 2016. New cancer registrations 2016. https://www.health.govt.nz/publication/new-cancer-registrations-2016.
- Ministry of Health New Zealand. 2019. Selected cancers 2015, 2016–2017. https://www.health.govt.nz/publication/selected-cancers-2015-2016-2017.
- Mosser DM, Edwards JP. 2008. Exploring the full spectrum of macrophage activation. Nat Rev Immunol. 8(12):958–969.
- Ng LK, Rich AM, Hussaini HM, Thomson WM, Fisher AL, Horne LS, Seymour GJ. 2011. Toll-like receptor 2 is present in the microenvironment of oral squamous cell carcinoma. Brit J Cancer. 104(3):460–463.
- Oble DA, Loewe R, Yu P, Mihm MC. 2009. Focus on TILs: prognostic significance of tumor infiltrating lymphocytes in human melanoma. Cancer Immun. 9:3.
- O’Higgins C, Ward FJ, Abu Eid R. 2018. Deciphering the role of regulatory CD4 T cells in oral and oropharyngeal cancer: a systematic review. Front Oncol. 8:442.
- Pathria P, Louis TL, Varner JA. 2019. Targeting tumor-associated macrophages in cancer. Trends Immunol. 40(4):310–327.
- Peinado H, Zhang H, Matei IR, Costa-Silva B, Hoshino A, Rodrigues G, Psaila B, Kaplan RN, Bromberg JF, Kang Y, et al. 2017. Pre-metastatic niches: organ-specific homes for metastases. Nat Rev Cancer. 17(5):302–317.
- Pickerill HP. 1912. Stomatology in general practice. London: Oxford Medical Publication.
- Pinto ML, Rios E, Durães C, Ribeiro R, Machado JC, Mantovani A, Barbosa MA, Carneiro F, Oliveira MJ. 2019. The two faces of tumor-associated macrophages and their clinical significance in colorectal cancer. Front Immunol. 10:1875.
- Punt S, Fleuren GJ, Kritikou E, Lubberts E, Trimbos JB, Jordanova ES, Gorter A. 2015. Angels and demons: Th17 cells represent a beneficial response, while neutrophil IL-17 is associated with poor prognosis in squamous cervical cancer. Oncoimmunol. 4:e984539.
- Rajjoub S, Basha SR, Einhorn E, Cohen MC, Marvel DM, Sewell DA. 2007. Prognostic significance of tumor-infiltrating lymphocytes in oropharyngeal cancer. Ear Nose Throat J. 86(8):506–511.
- Rollins BJ. 2006. Inflammatory chemokines in cancer growth and progression. Eur J Cancer. 42(6):760–767.
- Ruivo CF, Adem B, Silva M, Meio SA. 2017. The biology of cancer exosomes: insights and new perspectives. Cancer Res. 77(23):6480–6488.
- Sakaguchi S, Sakaguchi N, Asano M, Itoh M, Toda M. 1995. Immunologic self-tolerance maintained by activated T cells expressing IL-2 receptor alpha-chains (CD25). Breakdown of a single mechanism of self-tolerance causes various autoimmune diseases. J. Immunol. 186(7):3808–3821.
- Schacht V, Dadras SS, Johnson LA, Jackson DG, Hong YK, Detmar M. 2005. Up-regulation of the lymphatic marker podoplanin, a mucin-type transmembrane glycoprotein, in human squamous cell carcinomas and germ cells tumors. Am J Pathol. 166(3):913–921.
- Schroff WD. 1954. The value of simple aids to diagnosis. New Zeal Dent J. 50:102.
- Seo B. 2019. ER stress and UPR in oral cancer [PhD dissertation]. University of Otago.
- Shearer BH, Jenkinson HF, McMillan MD. 1994. Changes in cytokeratins following treatment of hamster cheek pouch epithelia with hyperplastic or neoplastic agents. J Oral Pathol Med. 23(4):149–155.
- Shearer BH, McMillan MD, Jenkinson HF. 1991. Comparison of the effects of four hyperplastic agents on hamster cheek pouch mucosa. J. Biol. Buccale. 19(4):315–318.
- Shearer BH, McMillan MD, Jenkinson HF. 1997. Differential expression of type 1 cytokeratins in hamster cheek pouch epithelium following treatment with dimethylbenzanthracene. J Oral Pathol Med. 26(10):470–476.
- Shen K, Johnson D, Vessey D, McGuckin M, Gobe G. 2018. Role of the unfolded protein response in determining the fate of tumor cells and the promise of multi-targeted therapies. Cell Stress Chaperones. 23(3):317–334.
- Snyderman CH, Heo DS, Johnson JT, D’Amico F, Barnes L, Whiteside TL. 1991. Functional and phenotypic analysis of lymphocytes in head and neck cancer. Arch Otolaryngol Head Neck Surg. 117(8):899–905.
- Strauss L, Bergmann C, Whiteside TL. 2009. Human circulating CD4 + CD25highFoxp3+ regulatory T cells kill autologous CD8+ but not CD4+ responder cells by Fas-mediated apoptosis. J. Immunol. 182(3):1469–1480.
- Symes GC, McMillan MD, Smillie AC, Boyd NM. 1995. Structure of hamster palatal gingiva and intermolar mucosa after intraperitoneal dosing with N-methyl-N-nitrosourea: a scanning electron microscope study. J Comparat Pathol. 112(4):403–415.
- Tauati-Williams E. 2019. Investigation of the presence of human papillomavirus in verrucal-papillary lesions of the oral cavity and comparison of viral detection methods [DClinDent (Oral Pathology) thesis]. University of Otago.
- Tsuneki M, Yamazaki M, Maruyama S, Cheng J, Saku T. 2013. Podoplanin-mediated cell adhesion through extracellular matrix in oral squamous cell carcinoma. Lab Invest. 93(8):921–932.
- Ugorski M, Dziegiel P, Suchanski J. 2016. Podoplanin-a small glycoprotein with many faces. Am J Cancer Res. 6(2):370–386.
- Uppaluri R, Dunn GP, Lewis JS. 2008. Focus on TILs: prognostic significance of tumor infiltrating lymphocytes in head and neck cancers. Cancer Immun. 8:16.
- Valadi H, Ekström K, Bossios A, Sjöstrand M, Lee JJ, Lötvall JO. 2007. Exosome-mediated transfer of mRNAs and microRNAs is a novel mechanism of genetic exchange between cells. Nat Cell Biol. 9(6):654–659.
- Wado-Ohno M, Ito T, Furue M. 2019. Adjuvant therapy for melanoma. Curr Treat Options Oncol. 20(8):63.
- Wanebo HJ. 1979. Immunobiology of head and neck cancer: basic concepts. Head Neck Surg. 2(1):42–55.
- Warnakulasuriya S. 2009. Global epidemiology of oral and oropharyngeal cancer. Oral Oncol. 45(4–5):309–316.
- Wei SC, Duffy CR, Allison JP. 2018. Fundamental mechanisms of immune checkpoint blockade therapy. Cancer Discov. 8(9):1069–1086.
- Wendler F, Bota-Rabassedas N, Franch-Marro X. 2013. Cancer becomes wasteful: emerging roles of exosomes in cell-fate determination. J Extracell Vesicles. 2. DOI:10.3402/jev.v2i0.22390.
- Whiteside TL. 2006. The role of immune cells in the tumor microenvironment. Cancer Treat Res. 130:103–124.
- Wolf GT, Hudson JL, Peterson KA, Miller HL, McClatchey KD. 1986. Lymphocyte subpopulations infiltrating squamous carcinomas of the head and neck: correlations with extent of tumor and prognosis. Otolaryngol Head Neck Surg. 95(2):142–152.
- Yakin M, Gavidi O, Cox B, Rich AM. 2017. Oral cancer risk factors in New Zealand. New Zealand Med J. 130(1451):30–38.
- Yakin M, Seo B, Hussaini HM, Rich AM, Hunter K. 2019a. Human papillomavirus and oral and oropharyngeal carcinoma: the essentials. Aust Dent J. 64(1):11–18.
- Yakin M, Seo B, Rich AM. 2019b. Tunicamycin-induced endoplasmic reticulum stress up-regulates tumour-promoting cytokines in oral squamous cell carcinoma. Cytokine. 120:1390–1143.
- Yamamoto M, Kikuchi H, Ohta M, Kawabata T, Hiramatsu Y, Kondo K, Baba M, Kamiya K, Tanaka K, Kitagawa M, et al. 2008. TSU68 prevents liver metastasis of colon cancer xenografts by modulating the premetastatic niche. Cancer Res. 68(23):9754–9762.
- Yu P, Fu Y-X. 2006. Tumor-infiltrating T lymphocytes: friends or foes? Lab. Invest. 86(3):231–245.
- Yuan P, Temam S, El-Naggar A, Zhou X, Liu DD, Lee JJ, Mao L. 2006. Over-expression of podoplanin in oral cancer and its association with poor clinical outcome. Cancer. 107(3):563–569.
- Zain RB, Asthirajan V, Ghani WMN, Razak IA, Raja Latifah RJ, Ismail SM, Sallam AA, Bustam AZ, Rahman ZA, Hussien A, et al. 2013. An oral cancer biobank initiative: a platform for multidisciplinary research in a developing country. Cell Tissue Bank. 14(1):45–52.
- Zancope E, Costa NL, Junqueira-Kipnis AP, Valadares MC, Silva TA, Leles CR, Mendonca EF, Batista AC, et al. 2010. Differential infiltration of CD8+ and NK cells in lip and oral cavity squamous cell carcinoma. J Oral Pathol Med. 39(2):162–167.
- Zhu X-D, Zhang J-B, Zhuang P-Y, Zhu H-G, Zhang W, Xiong Y-Q, Wu W-Z, Wang L, Tang Z-Y, Sun H-C. 2008. High expression of macrophage colony-stimulating factor in peritumoral liver tissue is associated with poor survival after curative resection of hepatocellular carcinoma. J Clin Oncol. 26(16):2707–2716.