ABSTRACT
The aim of this article is to examine research on inquiry-based chemistry education in primary and secondary schools to discuss how it is addressed in the research literature. A systematic review was conducted, including 102 articles published between 2000 and 2020. Through inductive analyses, the articles were categorised into four groups: (1) articles testing specific teaching approaches or models, (2) articles testing specific learning environments, (3) articles reporting on teachers and (4) additional relevant studies. Within each group, the articles were further categorised into five scientific domains (i.e. conceptual, epistemic, social, procedural and affective) and two categories: classroom practice and other. The experimental studies were also given a typology according to the quality of the methods applied. Overall, the research has been conducted with varied foci and it generally reports positive learning outcomes. However, the main emphasis is on the conceptual and affective domains, with fewer studies focusing on the epistemic domain. Finally, when it comes to methodology, the reviewed articles included many quantitative studies, often with few respondents and of varied quality. Thus, there is a need for more studies with larger numbers of participants, longer durations, more purposeful sampling and with focus on the epistemic and social domains.
Introduction
During the past few decades, there has been a global shift in science education from an emphasis on science for future scientists to the education of future citizens. The rapidly changing field of science and technology calls for an increased understanding of the criteria needed to evaluate knowledge (Duschl, Citation2008). Osborne (Citation2010) therefore argues that science for citizenship needs to emphasise how science works and that students should be given opportunities to study science-in-the-making and be allowed to interpret data and examine arguments that involve uncertainties.
There has also been greater emphasis on student-active teaching and learning practices, such as inquiry-based learning (IBL). Inquiry is not a new idea and was emphasised by Dewey, who argued that science education had suffered due to ‘so much ready-made knowledge, so much subject-matter of fact and law, rather than as the effective method of inquiry into any subject matter’ (Dewey, Citation1910, p. 124). He argued that students must have their own experiences, and he emphasised active learning and reflective thinking as the main purposes of teaching (Barrow, Citation2006). After Dewey, IBL was emphasised in major science educational reforms and is now one of the few overarching themes in science curricula worldwide (Abd‐El‐Khalick et al., Citation2004; Crawford, Citation2014; Crawford & Capps, Citation2018; Gericke et al., Citation2022).
Several meta-studies have revealed a positive effect of IBL on students’ learning (e.g. Furtak et al., Citation2012; Minner et al., Citation2010; Savelsbergh et al., Citation2016). Despite the massive endeavour to implement IBL, this approach has also been criticised for not working because of minimal guidance from the teachers (Kirschner et al., Citation2006). However, others have spoken out against this critique, arguing that it is founded on a misinterpretation of IBL as minimally guided instruction in line with discovery learning (Hmelo-Silver et al., Citation2007).
Some newer studies have used the results from international tests to investigate the effect of IBL on students’ learning. Teig et al. (Citation2018) used results from the TIMSS (Trends in International Mathematics and Science Study) 2015 test in Norway and found a curvilinear relationship between the prevalence of IBL in school science and students’ results. Students who had experienced a moderate amount of IBL scored better than their peers who had experienced IBL as either very prevalent or rarely used, where the former could be explained by IBL taking the place of other useful teaching activities (Teig et al., Citation2018). Aditomo and Klieme (Citation2020) studied the relationship between inquiry-based instruction and learning outcomes among the 10 highest- and 10 lowest-performing regions in PISA (Programme for International Student Assessment) 2015. They found that guided inquiry was positively associated with learning outcomes, while independent inquiry was negatively associated. This is in line with the meta-analysis conducted by Furtak et al. (Citation2012), who found that teacher-led IBL had a larger effect on learning than student-led IBL, and they therefore emphasised the importance of teacher guidance in the activities.
Science education is composed of different disciplines with different teaching traditions. In a study of secondary school science teachers’ conceptions, goals and enactment for inquiry-based teaching and learning, Breslyn and McGinnis (Citation2012) found that the teachers were more affected by which scientific discipline they taught than by the curriculum and other contextual factors. Teachers in biology and earth science emphasised teaching about the process of scientific investigations, physics teachers focused on modelling and generating mathematical expressions to describe physical phenomena and chemistry teachers mostly focused on content knowledge. Based on these results, they suggested offering discipline-specific examples of inquiry to teachers (Breslyn & McGinnis, Citation2012). Similar trends could be assumed to be the case for the research on IBL, and the aim of this study is therefore to specifically examine research on inquiry-based chemistry education (IBCE) in primary and secondary schools in order to discuss how it is addressed in the research literature.
While several reviews have been conducted related to inquiry-based science education (e.g. Furtak et al., Citation2012; Herranen & Aksela, Citation2019; Pedaste et al., Citation2015; Rönnebeck et al., Citation2016), little attention has been given to IBCE. Prior reviews in chemistry education have centred on general research trends (Cooper & Stowe, Citation2018; Teo et al., Citation2014), research on learning within specific chemistry topics (e.g. Bain & Towns, Citation2016; Graulich, Citation2015), context-based education (King, Citation2012) and laboratory work more generally (Agustian & Seery, Citation2017; Domin, Citation1999) – and not on IBCE. Furthermore, several of these reviews have tended to focus on tertiary education rather than on primary and secondary education (e.g. Agustian & Seery, Citation2017; Cooper & Stowe, Citation2018; Graulich, Citation2015). Therefore, a systematic literature review of peer-reviewed journal articles published in English between the years 2000 and 2020 was conducted in order to answer the following research questions (RQs):
What characterises the research literature on IBCE in primary and secondary schools regarding publication rate, geographical origin and the methods applied?
What are the foci in the research literature on IBCE in primary and secondary schools?
What are the most prominent findings reported in the research on IBCE in primary and secondary schools?
Theoretical background
Despite the long tradition of IBL in school science, it has been defined in different ways, which in turn has resulted in uneven implementation across classrooms (Crawford, Citation2014; Furtak et al., Citation2012). Research has revealed that, for many teachers, IBL has become synonymous with hands-on activities or laboratory work (Crawford & Capps, Citation2018; Osborne, Citation2014a, Citation2014b): ‘At its worst, the product is cookbook laboratory exercises where students simply follow a series of instructions to replicate the phenomenon’ (Osborne, Citation2014b, p. 178).
This review relies on definitions of IBL from Crawford (Citation2014) and Furtak et al. (Citation2012). Crawford (Citation2014) defines inquiry as follows:
Inquiry involves engaging students in using critical thinking skills, which includes asking questions, designing and carrying out investigations, interpreting data as evidence, creating arguments, building models, and communicating findings in the pursuit of deepening their understanding by using logic and evidence about the natural world. (p. 515)
This definition is related to teaching science as inquiry (Crawford, Citation2014), which includes both the pedagogy used when learners are active in the learning process, designing and carrying out practical or theoretical investigations, and the learning outcomes, such as scientific knowledge and the nature of science (NOS). It is also in line with the concept of scientific practices (National Research Council, Citation2012), which, in recent years, has largely replaced inquiry in the literature to focus on the methods used in authentic science (Gericke et al., Citation2022).
Furtak et al. (Citation2012) define IBL in two dimensions: the guidance provided by the teacher and the cognitive and social activities the learners are engaged in. With respect to teacher guidance, Furtak et al. (Citation2012) emphasise the importance of students being active during knowledge construction, and they describe it as a continuum from traditional instruction, which is teacher-led, to discovery learning, which is student-led. This is in line with Schwab (Citation1960), who identified three types of inquiry that differ in the levels of guidance in the laboratory: structured inquiry, where the teacher provides both the question and the method; guided inquiry, where the teacher gives the question but not the method; and open inquiry, where the learner may choose both the question and the method. Several researchers have defined categories along this continuum (e.g. Banchi & Bell, Citation2008; Gyllenpalm et al., Citation2010).
The cognitive and social activities described by Furtak et al. (Citation2012) are founded on the work of Duschl (Citation2003, Citation2008), who argued that the conditions for science learning are improved if students engage in active learning environments where the cognitive, epistemic and social domains are integrated. The conceptual domain includes facts, theories and scientific principles, and concerns constructing scientific understanding and reasoning. In chemistry education, scientific reasoning is related to the three levels of chemistry (Johnstone, Citation1991): macro, which are phenomena that can be observed; sub-micro, which involves the use of theoretical models at a particulate level that are developed to make sense of the observations; and symbolic, which is formulas, equations etc. A concern within chemistry teaching is that it tends to focus on the sub-micro and symbolic levels, without helping students to build connections between these three levels (Talanquer, Citation2011). The epistemic domain is related to how knowledge is created and evaluated, and includes understanding NOS and the fact that scientific knowledge is tentative in its character and could change in the face of new evidence (Lederman & Lederman, Citation2012). Students should gain experience in drawing conclusions based on evidence (Furtak et al., Citation2012), but NOS still needs to be addressed explicitly (Bell et al., Citation1998). Finally, the social domain includes an awareness of the social process of knowledge creation and the role of communication and argumentation in this process (Duschl, Citation2008). The scientific domains are intertwined, and learners need to develop knowledge within the conceptual and epistemic domains in order to engage in the social domain through scientific argumentation and to develop and evaluate claims (Duschl, Citation2008). Furthermore, Duschl (Citation2008) stresses that science education needs to shift from a dominant focus on conceptual learning to include epistemic and social learning goals as well in order to achieve a more balanced learning focus.
Other researchers have used these domains as analytical frameworks and have added more domains. In their meta-analysis of inquiry-based teaching, Furtak et al. (Citation2012) added the procedural domain as a fourth domain, which is linked to scientific methods, and this includes asking questions and designing and conducting scientific experiments. In a recently conducted literature review about inquiry-based science teacher education, Strat et al. (Citation2023) included the affective domain as the fifth domain in the analysis. The affective domain is used by others as well (e.g. Rodriguez et al., Citation2020) and includes identity, attitudes, beliefs and self-efficacy related to IBL and science in general. These five domains constitute the starting point of the analytical framework used in this article, which will be explained in the following sections.
Methods
This review is inspired by a systematic review approach (cf. Grant & Booth, Citation2009; Sutton et al., Citation2019). The search was conducted on 7 July 2020 and was restricted to journal articles published between 2000 and 2020. It was further restricted to articles written in English to investigate research that is available to the wider research community.
Search procedure
The library databases ERIC and the Web of Science were searched to provide an initial list of articles. ERIC was included as a disciplinary database publishing education-related resources, and Web of Science was included as a multidisciplinary database. In addition, a manual search for relevant articles published before 7 July 2020 was conducted in the following eight journals: International Journal of Science Education (IJSE), Science Education (SE), Research in Science Education (RSE), Journal of Research in Science Teaching (JRST), International Journal of Science and Mathematics Education (IJSME), Research in Science & Technological Education, Journal of Chemical Education (JCE) and Chemistry Education Research and Practice (CERP). These journals were chosen because they have the highest impact factor among the science and chemistry education journals with the relevant scope and, hence, they represent trend-setting journals in the field. Several of these journals are also the ones included in manual searches in other review articles (e.g. Teo et al., Citation2014).
The interest of this study lies in all articles related to IBCE and not only in articles that the authors have labelled as IBCE. The search terms enquiry and problem-based were therefore included, in addition to inquiry, to ensure that relevant studies for the concept were targeted. Enquiry is sometimes used as a synonym for inquiry, especially in British English (Barrow, Citation2006) and was therefore chosen. Furthermore, problem-based learning was included, since it can be seen as an approach to IBCE that focuses on teaching and learning based on concrete problems (Dobber et al., Citation2017).
The queries used to find the appropriate articles are shown in below. The search terms were used for the fields ‘title’, ‘abstract’ and ‘keywords’ in the databases. To broaden the search, but at the same time keep it narrow enough, a proximity operator was used in the first search (S1 and S5) to include articles that had relevant terms (i.e. teaching, learning, based, activity or oriented) in a proximity of five words from inquiry or enquiry. The search terms used in the two databases were similar, except for the term related to education (S6) that was used in the Web of Science but was not needed in ERIC, since ERIC is already targeted only towards journals in education.
Table 1. Inquiry-based chemistry education: searches in ERIC and the Web of Science.
The search resulted in 1906 articles, which, after narrowing the search down in terms of years and publication type, and by removing duplicates, resulted in 1181 articles. The further selection of studies was conducted in three steps (steps A, B and C), as illustrated in and explained below.
Selection of studies
The selection of studies was guided by the inclusion and exclusion criteria listed in below. The criteria were based on the RQs and were conscious choices that had to be made during the selection process. Some judgements had to be made during the selection process, since some of the inclusion and exclusion criteria exist on a spectrum and are not dichotomous; these judgements are commented on in relation to . During the selection process, all articles were first examined with respect to titles, abstracts and keywords (step A in ). Secondly, the full texts were examined (step B). Thirdly, the manual search in the eight journals mentioned above was conducted (step C). Applying these three steps led to a final pool of 102 articles, including seven articles from the manual search (1 from RSE, 1 from IJSE, 2 from IJSME and 3 from CERP). A complete list of the 102 articles can be found in Appendix A.
Table 2. Inclusion and exclusion criteria developed based on the research questions.
Analysis and methodological reflections
To answer the RQs, the final pool of articles was read and analysed with respect to the following: year of publication, geographic origin of the study, type of method, number of participants, target group (students or teachers) and school level. These categories gave an overview of the research in the field and this is presented in the first part of the results section. The articles were further analysed inductively in three phases. The first analysis of the articles revealed that many of them tested specific teaching approaches, applied specific learning environments or were articles where teachers reported on their implementation of IBCE. Thus, during the first phase of the analysis, the articles were categorised into four groups, as listed in . These groups constitute the headings of the second part of the results section. Within each group, the articles were further categorised into relevant sub-groups, such as a sub-group describing a specific teaching model in Group 1 or the specific learning environment in Group 2. The sub-groups constitute the sub-headings of each section in the results section.
Table 3. An overview of the groups and sub-groups of the analysis.
The four groups with corresponding sub-groups were considered mutually exclusive, meaning that an article was placed in one group only. There was one article that used a specific teaching model combined with virtual or real media (Sugiharti & Habeahan, Citation2018), and therefore could have been placed in both Group 1 and Group 2. This article was placed in Group 2, since the main focus was on the type of media, not on the teaching model itself. Furthermore, there were two articles exploring teachers’ implementation of technology-based learning environments (Donnelly et al., Citation2013; Tolentino et al., Citation2009) that were placed in Group 3 instead of Group 2, since the main emphasis was on the teachers. Otherwise, there were no issues in categorising the articles with respect to the groups.
In the second phase of analysis, the articles were categorised according to the five domains presented earlier (i.e. conceptual, epistemic, social, procedural and affective) and two other categories that were found inductively. First, a category named classroom practice was included to categorise articles focused on describing how IBCE was implemented in the classroom. The articles included in this category either had an explicit aim of describing the implementation of an activity or described a teacher’s practice (typical of several of the articles in Group 3). Secondly, a category named other was included to capture aspects that were not included in the other domains, such as creative thinking, drawing and metacognition. When referred to jointly, the five domains and two categories are hereafter referred to as the seven domains.
Since the articles reviewed in this study typically had more than one focus area, the same article could be categorised into more than one domain. The main challenge relating to this categorisation was that some studies investigated other or more things than they, in their aims or RQs, argued that they would investigate. Typically, they said they were investigating one or two issues but had results covering more – or other – things. In cases where they said they had investigated one issue but investigated another, the articles were categorised with respect to what they actually did. In cases where they investigated two things but also had superficial results from other things, they were categorised according to what they said they did, which often coincided with what they discussed. There were also some overlaps between the domains. For instance, working with practical experiments may improve the students’ procedural knowledge, but also their epistemic knowledge. Furthermore, since the three scientific domains from Duschl (Citation2003, Citation2008) are intertwined, there are some topics that could be placed in more than one domain, such as argumentation which is placed in the social domain in this article, since it concerns communicating scientific ideas. There were also some articles that focused on students’ problem-solving competence, which in some articles was related to problem-solving in the laboratory, while in other articles, the focus was on problem-solving in general. Thus, the latter articles were placed in the category ‘other’, whereas those connected to laboratory work were categorised in the procedural domain.
In the third and final phase of analysis, articles reporting from experimental studies (both quantitative and mixed methods studies) were categorised according to the quality of the methods applied. 40 of the 43 quantitative articles and 26 of the 32 mixed method articles were experimental studies, while the remaining articles either were related to tests or surveys (e.g. Cheung, Citation2011; Vhurumuku, Citation2011) – or mixed method studies mostly applying qualitative methods (e.g. Juntunen & Aksela, Citation2013b; Smith, Citation2012). Experimental studies are often used to test the effect of teaching innovations, but they need to be well described for authors to evaluate their implications (Taber, Citation2019). Thus, Taber (Citation2019) argues that it is good practice to report the units of analysis, sampling and detailed descriptions of the different treatments, as well as effect size.
The articles reporting from experimental studies were given a typology as shown in . First, the articles were categorised according to whether there was a control or comparison group or not, and if there was such a group, the type of comparison conditions were identified, following a classification from Taber (Citation2019), where level 1 implies no treatment for the control/comparison group, level 2 implies standard treatment for the control/comparison group (i.e. ‘traditional teaching’) and in level 3, the control/comparison group is treated with teaching that is acknowledged as good practice. Secondly, the articles with a control or comparison group were categorised according to whether the teaching for this group was described or not. Finally, the articles were categorised according to whether the effect size was reported or not.
Table 4. Categorisation of experimental studies according to the quality of the methods applied.
The categorisation into typologies was mutually exclusive. If there were combinations of treatments, for example both level 2 and level 3 comparison conditions, the articles were always lifted to the ‘higher’ typology (e.g. A rather than B). Thus, the article from Apedoe et al. (Citation2008) reporting from both a study without a control group and a study with a level 1 control group, was assigned C1. When it comes to the description of the treatment for the control or comparison group, the articles labelled ‘described’ had to describe the specific treatment in some detail, rather than just stating that they used traditional methods with lectures and students answering questions from the textbook.
The analysis of the mixed method studies revealed that the articles had three approaches to mixed methods: (1) some articles were mostly quantitative in character and used qualitative methods to provide results related to another research question (often related to the affective domain); (2) some articles were mostly quantitative in character and used qualitative methods to gain a deeper understanding of the results of the quantitative analyses (i.e. the explanatory design, according to Creswell (Citation2014)); and (3) some articles used both quantitative and qualitative methods to answer the question at hand (i.e. the convergent design, according to Creswell (Citation2014)). Thus, the typology in the mixed methods studies must be seen in relation to the qualitative methods, especially in the third approach.
In line with suggestions from Egger et al. (Citation2003), two steps were taken to increase the validity of this research: (1) all relevant studies were included in the review and (2) methodological quality was ensured due to the transparent documentation from the review process. First, a systematic review features a comprehensive search, including both searches in bibliographic databases and supplementary search methods (Sutton et al., Citation2019). The search in this review was conducted using two databases, one subject-specific (i.e. Web of Science) and one for articles published in education (i.e. ERIC). In addition, an extensive manual search was conducted. The manual search in seven of the eight journals resulted in only four additional articles, indicating that the database search was quite successful. The search in CERP resulted in a total of three articles. These articles were all published in 2020, indicating that they had not yet been registered in the databases at the time of the search. Nevertheless, the inclusion of an extended manual search contributed to the validity of this research.
The second step to achieving validity in systematic reviews is transparency, allowing others to replicate the process (Grant & Booth, Citation2009; Sutton et al., Citation2019). The search procedure and the selection and analysis of articles have therefore been described thoroughly in both the methods and results sections, and the categorisation of the articles according to the three types of analyses are listed in Appendix A. In addition, thoroughness was aimed for in all phases of the research. Thus, too many rather than too few articles were included, and if there was any doubt about whether an article should have been excluded or not, the article was kept back for another round. The analytical process was also thoroughly undertaken, and the articles were revisited several times, if needed, during the analysis and writing phases. Finally, the frameworks and articles were discussed with colleagues who also read both drafts and the final text. However, since the inclusion and exclusion criteria exist on a spectrum, other scholars might have included other articles. Furthermore, due to the overlapping domains, others might have categorised the articles differently as well.
Results
In this section, the findings from the review are presented according to the RQs. First, a general overview of the analysed studies is given relating to the publication rate, geographical origin and methods (RQ 1). This section also includes an overview of the included studies relating to the four groups and seven domains (RQ 2). Thereafter, the distribution of articles according to groups and domains is explored in depth in relation to the findings (RQ 3).
A general overview of the analysed studies
provides an overview of the year of publication of the 102 articles included in the study. There were no studies on IBCE between 2000 and 2003. In 2004 and 2005, there were three studies, while there has been a rather constant number of publications on IBCE since 2008; in the time period from 2008 to 2019, there has been an average of eight studies published per year.
Figure 2. Number of publications per year about IBCE in primary and secondary schools.
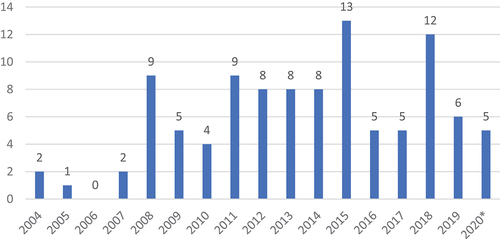
When it comes to the origin of the studies, research on IBCE seems to be of interest worldwide, as illustrated in The research included in this study was conducted in 27 different countries, including one study that collected data from Brazil and from three European countries (van Rens et al., Citation2004). Looking at the different continents, Asia (N = 48) stands out, with a high number of publications, followed by North America (N = 26) and Europe (N = 21), while the number of publications in South America (N = 2) and Africa (N = 5) is rather low. When it comes to Australia, no publications were included in this review.
Figure 3. Number of publications per country.
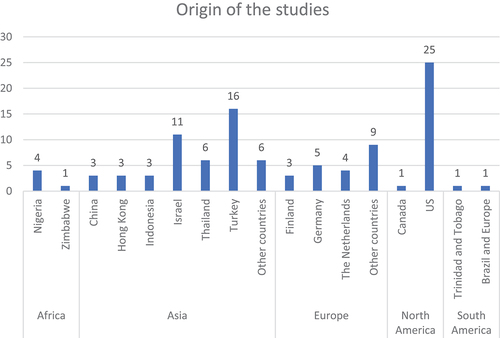
The publications on IBCE studies in Europe and Asia were from a large range of countries. In addition to the countries listed in , there were European publications from Ireland (N = 2), Hungary (N = 2), Poland, Sweden, Slovakia, Spain and the United Kingdom (all N = 1). In Asia, the category other countries includes Malaysia (N = 2), Bhutan, the Philippines, Qatar and Singapore (all N = 1).
provides an overview of the distribution of articles according to groups relating to methods, the number of participants, typology, school year, country and domains. As can be seen from the table, there are many quantitative studies or mixed-method studies, and half of the studies contained more than 50 participants. Overall, the studies included in the article were typically quasi-experimental with complete classes assigned to different conditions. There were some studies where individuals were assigned across classes, but students in the same class are not considered as independent learners as they influence each other (Dorman, Citation2012), and these studies were not considered to be ‘true experiments’ (Taber, Citation2019). When it comes to the typology of the experimental studies, the majority of studies belongs to level 2 control conditions (i.e. standard treatment of control group) (N = 27), followed by studies with level 3 control conditions (N = 20) and without control group (N = 17). The studies categorised with level 3 control or comparison group were of two different types: they either compared different experimental groups, sometimes also with an additional control group (e.g. Sola & Ojo, Citation2007), or they assigned the same treatment to different types of students, studying for instance the effect of different learning styles on learning outcomes (Sudria et al., Citation2018).
Table 5. Frequency of studies according to groups.
When it comes to the age of the students, there is an overweighting of studies with students in grades 9 to 12. Furthermore, 25% of the studies were completed in the United States, followed by Turkey (16%) and Israel (11%). Regarding domains, most articles were in the conceptual domain, followed by the affective domain. These numbers will be further commented on in the following sections, along with details from the included studies in the respective groups.
Group 1: articles testing specific teaching approaches or models
Thirty of the included articles tested a specific teaching approach or teaching model with respect to the development of different aspects of content knowledge or skills. Some articles studied the effect of the actual model on students’ learning or development, while in other articles, the model was more of a context. gives an overview of the articles according to methods and domains; the articles covering the different teaching approaches are explored in the following sections.
Table 6. Overview of the articles categorised in group 1 according to methods and categorisation.
Problem-based learning (PBL)
Ten of the articles in Group 1 focused on problem-based learning (PBL). PBL is a specific approach to IBL that was developed in higher education, originally within the context of medical education (Dobber et al., Citation2017), and it has been widely used in chemistry in higher education. PBL is a sub-set of context-based learning, where learning takes place in a meaningful context by starting with an open-ended problem/scenario (Overton et al., Citation2009). The problem is introduced to the learners before they have the in-depth knowledge for solving it, with the aim of motivating them to learn (Drake & Long, Citation2009).
Five of the included PBL studies were conducted in Turkey and investigated the effectiveness of PBL for a specific topic of chemistry in the conceptual domain – in addition to a more educational topic, typically in the affective domain. Baran (Citation2016) investigated the effects of PBL on 9th-grade students’ academic achievement when learning about gases, in addition to their attitudes towards and motivation for learning chemistry. She found significant changes in achievement level, but no significant change in motivation and attitudes. The remaining four studies were conducted by Tarhan and colleagues. Ayyildiz and Tarhan (Citation2018) investigated the effect of PBL on 11th-grade students’ learning of enthalpy changes and their opinions about PBL as an instructional technique. They found that the experimental group learned significantly more and had fewer misconceptions than the control group. The students also reported several benefits, including increased motivation and self-efficacy, and a desire to learn chemistry. Similar results were reported from another study, where Tarhan and Acar (Citation2007) studied the effectiveness of PBL on 11th-grade students’ understanding of the effect of temperature, concentration and pressure on cell potential and their social skills. Tarhan and colleagues also examined 9th-grade students’ understanding of intermolecular forces (Tarhan et al., Citation2008) and 11th-grade students’ understanding of topics related to acids and bases (Tarhan & Acar-Sesen, Citation2013). In these two studies, they found that the experimental group increased their learning achievement and had fewer alternate conceptions, and the students saw several benefits to PBL. However, both studies also revealed some challenges related to the increased responsibility involved in PBL and that a minority of the students were ‘not ready for PBL’ (Tarhan et al., Citation2008, p. 297).
In a study in the United Kingdom by Smith (Citation2012), she described the development and implementation of practical activities within a problem-based laboratory approach to develop learners’ understanding of practical techniques relevant for university studies, placing it in the procedural domain. Ten practical activities were developed, and the problems were set in a real-life context. The problems included pre-lab work, group work and a form of assessment, and they were tested with 106 students aged between 16 and 18 years old in eight different schools; each group of students tested one problem-based activity. The students argued that the problem-based activities were more interesting than typical instruction and ‘better for making them think’ (Smith, Citation2012, p. 497). The students were also positive towards the approach in relation to several skills (e.g. collaboration, communication and research skills).
The remaining studies investigated the effect of PBL on affective aspects, such as attitudes towards science and the learning environment (Ferreira & Trudel, Citation2012), and different kinds of skills, such as practical skills in chemistry (Osokoya & Nwazota, Citation2018), problem-solving skills (Abubakar & Arshad, Citation2015; Ferreira & Trudel, Citation2012; Valdez & Bungihan, Citation2019) and self-regulated learning (Abubakar & Arshad, Citation2015). Osokoya and Nwazota (Citation2018) found that those exposed to PBL were better at practical chemistry than the control group and that students from single-sex schools performed better than those from co-ed schools. Other studies also reported favourable outcomes (Abubakar & Arshad, Citation2015; Ferreira & Trudel, Citation2012; Valdez & Bungihan, Citation2019). However, Ferreira and Trudel (Citation2012) found that the students had some difficulties related to problem-solving, especially with listing preliminary facts, suggesting the best solution and contributing with new facts and solutions.
Argument-driven inquiry (ADI)
The second most applied model was argument-driven inquiry (ADI) (N = 6), which is an instructional model developed by Sampson et al. (Citation2009). The model intends to ‘serve as template or a guide that instructors can use to create school science laboratory experiments that are more authentic and educative for students’ (Sampson et al., Citation2013, pp. 644–645). The model is composed of eight stages. In the first stage, the topic is introduced and the learners receive a guiding question that is supposed to capture their attention and interest. In the second stage, groups of students design and implement a method to address the question, while in stage three, they analyse their data and develop a tentative argument that is supported by evidence. These arguments are shared with peers in small groups in step four. After step four, the students may return to stage three and collect more data. In step five, the students create individual written investigation reports that include three sections: the goal of the investigation, the method used and the argument. These reports are submitted to the teachers as three-four copies that are randomly distributed to groups of students along with a peer-review sheet for a double-blind group peer review. In the seventh stage, the students rewrite their reports based on the reviewers’ comments and the final report is handed in to the teacher. After the report has been handed in, there is an explicit and reflective class discussion in stage eight about what they learned about the content, the nature of scientific inquiry and the NOS. They also discuss what could have been done better (Sampson et al., Citation2013).
In the included study from Sampson et al. (Citation2011), they conducted an 18-week intervention in grade 10 to explore how the ADI model influenced students’ participation in argumentation and crafting written arguments. This was therefore an example of the social domain. They conducted the same performance test before and after the intervention, in which groups of students were supposed to explain a scientific phenomenon and write a scientific argument. The authors found that more students participated in the discussion after the intervention and that the groups had a higher proportion of discussion and oppositional comments, indicating increased engagement and a stronger willingness to engage in argumentation. Furthermore, their explanations were more accurate, indicating increased understanding. However, the students did not use scientific theories or laws in their explanations and the model did not seem to have an impact on this aspect of argumentation. When it comes to their written arguments, the analyses showed that the students wrote better arguments (especially with respect to the quality of the evidence and the sufficiency of the reasoning), but the explanations were often ‘conceptually inaccurate’ (Sampson et al., Citation2011, p. 244). Overall, the students developed a more nuanced understanding of the components of a scientific argument during the study, which is considered important both for students to be able to provide evidence and reasoning for their claims and to be able to assess other people’s reasoning and claims.
Two other studies applying ADI also focused on different facets of argumentation and were categorised in the social domain. In a study in grade 9, Çetin and Eymur (Citation2017) found that the students improved their writing skills and that their ability to communicate scientifically via posters also increased after participating in five lab activities based on ADI. In the affective domain, they found that the students found peer reviewing their fellow students’ reports and revising the reports after peer revision to be the most helpful aspects of the ADI model. In a similar study with 30 students who experienced the ADI model and 30 students in a control group, Çetin et al. (Citation2018) found that only the experimental group increased their scientific writing skills and that the girls increased their skills more than the boys. Furthermore, the experimental group scored significantly higher than the comparison group on content knowledge (i.e. the conceptual domain). When reflecting on the method in the affective domain, the students found the argument sessions useful, and even though some students found the writing sessions to be repetitive, they had positive perceptions. The teacher was most enthusiastic about the stages where the methods and arguments were created but also pointed to the problem that the students’ written argumentation, to a large extent, focused on what they had done rather than on discussing their findings.
In the epistemic domain, Eymur (Citation2019) investigated how explicit NOS instruction embedded in ADI activities affected grade 11 students’ conceptions of NOS. The author found that the students’ views of NOS developed significantly among the 24 students who followed explicit instruction, whereas the views of NOS among the students in the control group (N = 21), who were taught with an implicit focus on NOS and who followed the structured inquiry model, did not change. The two remaining studies were placed in the affective domain and ‘other’, respectively, and they investigated 10th-grade students’ self-efficacy and perceptions about IBCE and chemistry (Eymur, Citation2018) and creative thinking (Kumdang et al., Citation2018) after participating in lab activities following the ADI approach. Both articles reported improvements in the aspect under study.
Process-oriented guided inquiry learning (POGIL)
Process-oriented guided inquiry learning (POGIL) was another commonly used model (N = 6) that in five of the reviewed articles was applied with students in grades 10–11, while in the latter with students from grade 6. POGIL is a collaborative, learner-centred, inquiry-based instruction strategy that is built upon social constructivism and follows a three-phase learning cycle (Eberlein et al., Citation2008). The learning cycle follows a series of questions and starts with an exploration phase where the learners examine a model (i.e. a combination of pictures, graphs, equations etc.); the questions guide the learner to test hypotheses or explain relationships that can be found in the model. Secondly, in the concept intervention phase, a specific concept or term is introduced, and learners discover connections and patterns in the data. Finally, there is an application phase in which the learners apply concepts to new situations (Eberlein et al., Citation2008; Hanson, Citation2006). Throughout the learning cycle, the learners work in teams, and designated roles, such as a manager, spokesperson, recorder or strategy analyst, can be given to promote interdependence and involvement (Hanson, Citation2006).
Four of the included articles studied the effect of POGIL on learning a specific chemistry topic and were, hence, categorised in the conceptual domain. Barthlow and Watson (Citation2014) studied the effect of POGIL on reducing alternative conceptions related to the particulate nature of matter among students in the 10th and 11th grades. In their study, which included pre- and post-tests, they found fewer alternative conceptions among the students in the treatment group compared to students who experienced a traditional lecture pedagogy. They found no significant differences related to gender or race. Similarly, Sen et al. (Citation2016) investigated the effect of POGIL on 11th-grade students’ understanding of electrochemistry and found that the students learning through POGIL performed better and had fewer misconceptions than the students in the control group. De Gale and Boisselle (Citation2015), on the other hand, who investigated high-school students’ academic performance and confidence after participating in a unit in organic chemistry, found varied academic performance among the participants, arguing that the learners’ inexperience with POGIL may have affected the results. However, they also found that POGIL improved the learners’ academic confidence (i.e. the affective domain). Finally, in a study conducted in middle school, Özkanbaş and Kırık (Citation2020) found that POGIL had a positive effect on 6th-graders’ understanding of the nature of matter.
Within the category ‘other’, Sen et al. (Citation2015) found that POGIL was better than traditional pedagogy at developing 11th-grade students’ skills in self-regulated learning. They also found improvements in the learners’ motivation and learning strategies. Finally, Treagust et al. (Citation2020) adapted POGIL, which is based on Western cultural and educational contexts, to the Qatari context and studied the students’ perceived relevance and perceptions of learning before and after POGIL instruction. They found that the use of roles to facilitate communication was positive and that POGIL improved the learners’ perceptions of chemistry learning and academic performance.
5E model with variations
Four articles applied different variants of the 5E model and focused on the conceptual domain. The 5E model is an instructional model to support inquiry-based teaching that involves students in five phases: engage, explore, explain, elaborate and evaluate (Bybee et al., Citation2006). First, the learners are engaged in the task and links are made to prior experiences. Thereafter, the learners explore the ideas, often through data collection, before the ideas are explained. Finally, the students elaborate on the ideas and apply them in new contexts to increase their understanding. Throughout the process, the students’ understanding is evaluated by the teacher and by themselves (Bybee et al., Citation2006).
Supasorn (Citation2015) developed small-scale experiments following the 5E model that were implemented in conjunction with a galvanic cell model kit featuring macroscopic, sub-microscopic and symbolic levels in order to investigate grade 12 students’ conceptual understanding and mental models for understanding galvanic cells at all three levels (i.e. macro, sub-micro and symbolic). Supasorn and Promarak (Citation2015) also conducted a similar study on reaction rate, investigating grade 11 students’ conceptual understanding after participating in IBCE based on the 5E model with analogies incorporated. Both studies found positive effects on conceptual understanding. Sotakova et al. (Citation2020) developed inquiry activities based on the 5E model and investigated the effect on students’ understanding of changes in chemical reactions. They found that the experimental group performed significantly better in terms of conceptual understanding – and that the students in the experimental group with lower academic grades had the biggest change from the pre- to the post-test.
Adesoji and Idika (Citation2015) conducted a study in which they compared students’ achievement in and attitudes towards chemistry after participating in different types of teaching. Two hundred and eight students participated in the study and 114 of them were taught through something called the 7E model, which is similar to the 5E model but puts more explicit emphasis on prior knowledge (elicit) and applying the knowledge to new contexts (extend). Furthermore, 50 of them participated in case-based learning, which is similar to PBL, and the remaining 44 students were taught through conventional methods. The authors found that teaching through the 7E model and case-based learning were more effective both with respect to achievement in and attitudes towards chemistry, and that case-based learning was most effective for both aspects.
Other models
Four of the included articles used one of three types of models other than those mentioned earlier: the science writing heuristic (SWH), explanation-driven inquiry (EDI) and the designed student-centred instruction (DSCI). Kingir et al. (Citation2012) and Kingir et al. (Citation2013) investigated the effects of SWH on Turkish grade 9 students’ academic achievement in the topics of chemical change and mixtures. SWH is an argument-based inquiry approach building on a writing-to-learn framework that guides teachers and learners in negotiating meaning from experiments. It includes a teacher framework with a series of suggested activities for the teacher to guide the students in writing, reading and discussions, and a student framework that includes questions that prompt students to build knowledge and construct scientific arguments (Keys et al., Citation1999). In Kingir et al. (Citation2012), the authors found through a quantitative study that the students following SWH performed significantly better than the control group. They also found that the low- and middle-achieving students performed particularly well and the achievement gap between low- and high-achieving students based on prior results disappeared. In Kingir et al. (Citation2013), they conducted both quantitative studies and in-depth interviews to examine the students’ conceptual understanding. They found that the students in the SWH group performed significantly better than the comparison group, but that students in both groups still held misconceptions after the teaching element. The results from the interviews were consistent with those observed in the test. The authors attributed the good results of the students’ learning through SWH to social interactions, both between the students themselves and between the students and the teacher (Kingir et al., Citation2013). Lu et al. (Citation2018) studied the effect of EDI, ‘which frames students’ inquiry as an effort to explain phenomena’ (p. 1857) and found significant effects on students’ conceptual understanding of redox reactions and that misconceptions were less frequent, especially related to the movement of electrons and spectator ions. Finally, Rahayu et al. (Citation2011) studied the effect of DSCI, a teaching programme that combines a constructivist approach and a hands-on inquiry approach with context-based learning through a focus on students’ everyday experiences and environmental issues. The authors found that the experimental group performed significantly better than the comparison group on acid-base concepts and displayed increased interest in learning science.
Summary of the articles in group 1
To sum up the categorisation of the articles testing different teaching models, they were mostly conducted with students in grades 9 to 11 and half of them took place in Turkey (N = 14). When it comes to the categorisation, the articles were mostly categorised in the conceptual domain (N = 18), investigating students’ achievements and/or misconceptions after participating in a teaching activity following the specific model. This is often in connection with the affective domain (N = 14), investigating students’ perspectives on the specific teaching model – or how their affection towards or motivation for chemistry changed as a result of their participation in the activity. Only one article was categorised in the epistemic domain, investigating the effect of explicit NOS teaching on students’ conceptions of NOS, whereas three articles were categorised in the procedural domain. Four articles were categorised in the social domain and three of these were, not surprisingly, connected to the ADI model, investigating students’ argumentation skills. Thus, the articles exploring the ADI model differed from the remaining articles with their focus on domains other than the conceptual domain (only one article was categorised in the conceptual domain). The seven Group 1 articles categorised as ‘other’ focused on different types of skills (study skills, creative thinking and problem-solving), self-regulated learning and the learning environment.
When it comes to the methods applied, they were mostly quantitative (N = 14) or mixed methods (N = 13), often with a strong quantitative component. In some of the mixed-method studies, the qualitative part was in the affective domain, investigating the students’ perceptions of the model. Only three studies were qualitative, and two of them were related to students’ argumentation. Even though many of the studies in this group were quantitative, they were of varied quality, as can be seen based on the typology. Six of the studies did not have a control or comparison group, and five of these did not report effect size (i.e. D2). Furthermore, there were eight studies which failed to describe the comparison conditions (i.e. A3, B3 and B4). There were also studies having a superficial character, focusing on several aspects in a small study – or having relatively few respondents (between 30 and 50). However, there were also several examples of thorough and well-conducted studies (i.e. B1).
Group 2: articles testing specific learning environments
Twenty-four articles were categorised in Group 2, focusing on specific learning environments. These learning environments included technology-based learning environments, authentic science experiences, and games and comics. The results of the analysis are given in .
Table 7. Overview of the articles categorised in group 2 according to methods and categorisation.
Technology-based learning environments
Thirteen articles in this review used technology-based learning environments as the starting points for their studies. Most of these studies used specific learning environments or programmes to explore students’ learning. Thus, similar to the articles in Group 1, most of these articles were categorised in the conceptual domain.
Many articles using technology-based learning environments explored different types of virtual experiments. In the conceptual domain, Davenport et al. (Citation2018) investigated how ChemVLab+ (i.e. ‘online activities that enable students to learn core concepts while carrying out investigations in real-world contexts’ (p. 1250)) affected high-school students’ learning of stoichiometry, equilibrium and thermodynamics. They found significant improvements from the pre- to post-test stages and found that students who completed more activities scored better. The activities contained paired tasks and the authors further found that the students applied understanding gained from the first task when solving the second. They also found that the students benefited most from working independently on the tasks (Davenport et al., Citation2018). Pyatt and Sims (Citation2012) investigated the value of physical and virtual experiments for students in first-year chemistry in high school and found that they were similar in terms of student performance in the conceptual and procedural domains, but that, in some cases, the students performed better after conducting virtual experiments because the quality of the data was better, yielding a more accurate model of the phenomena under study. The students valued both physical and virtual experiments but appreciated that virtual experiments gave them more time to focus on the content and interpretations rather than on conducting the experiments; hence, they had positive attitudes towards the use of computers in the lab (Pyatt & Sims, Citation2012). Still in the conceptual domain, Sugiharti and Habeahan (Citation2018) explored students’ learning outcomes on thermochemical materials according to their numerical ability after participating in PBL combined with either real labs or virtual labs. They found that the class taught using a virtual lab had higher average results. They also found that students with high numerical ability had higher learning outcomes than those with low numerical ability.
With the main emphasis on the procedural domain, Dori and Sasson (Citation2008) explored how a case-based computerised laboratory (i.e. ‘a chemistry learning environment that integrates computerised experiments with emphasis on scientific inquiry and comprehension of case studies’ (p. 219)) affected 12th-grade students’ chemical understanding and graphing skills. They found that the students using the computerised laboratory improved their graphical skills but not their chemical understanding.
Finally, there were some articles exploring virtual labs in the ‘other’ category. Kaberman and Dori (Citation2009a) examined how metacognitive strategies included through a case-based computerised laboratory learning unit affected students’ skills at posing questions and analysing these questions according to a given taxonomy. Questionnaires showed that the students improved their question-posing skills significantly and the number and complexity of the questions were significantly higher. There was a significant difference in the experimental group compared to the comparison group. Furthermore, interviews with some of the students revealed that the students were able to analyse the questions and they were able to improve the questions during the interview. In another study, Kaberman and Dori (Citation2009b) investigated the effect of a case-based computerised laboratory combined with computerised molecular modelling on question posing, inquiry and modelling. They found a significant effect among the experimental group from the pre- to the post-test. The students asked more questions and more complex questions. When it comes to modelling skills, the learners improved significantly in transferring from 3D models to structural formulas, but half of the students struggled with the opposite transition. Still in the context of a virtual chemistry lab, Donnelly et al. (Citation2014) investigated how power relations either stifled or facilitated IBCE. They found that the traditional roles of the teacher and student were maintained: The teacher monitored completing rather than understanding the task, hence emphasising the product over the process, and there was a lack of student engagement in scientific ideas. Thus, the authors concluded that there was an ‘apparent presence of inquiry, but under the surface, traditional expectations, beliefs and values steer the lesson towards clearly defined directions. This study highlights the difficulty of enacting true inquiry within the complex power dynamics of the classroom’ (Donnelly et al., Citation2014, 2048).
Another topic explored by four articles was the use of visualisation tools. Stieff (Citation2011) discussed the design of a computer-based visualisation tool called the Connected Chemistry Curriculum and its impact on students’ learning and use of chemical representations within the topic of matter. He found that the students following the Connected Chemistry Curriculum showed small to moderate improvements in their achievements and significant improvements in their use of representations, especially in relation to the use of sub-micro representations. Stieff (Citation2019) also investigated the efficacy of the long-term impact of visualisation-supported inquiry activities from the Connected Chemistry Curriculum. The activities were implemented in three topics (i.e. the particular nature of matter, chemical reactivity and chemical equilibrium) and the students showed large improvements from the pre- to the post-tests. They also scored better on these topics in the end-of-year achievement assessment compared to topics in which they followed the district curriculum. In another study on visualisation tools, Zhang and Linn (Citation2011) explored 8th-grade students’ understanding of chemical reactions after working with visualisations using the Web-based Inquiry Science Environment, WISE. Eighty-one students generated drawings, visualising a chemical reaction before, right after the reaction started, some time after the initiation of the reaction and after completion, while the other 52 did not generate these drawings, and instead spent more time on the visualisations. The authors found that all students improved their understanding of chemical reactions, but that the students who generated the drawings had a more complex understanding, probably owing to them having to articulate and represent their ideas. Especially those with partially correct ideas improved their understanding (Zhang & Linn, Citation2011). Finally, Ryoo et al. (Citation2018) explored how visualisations could foster 8th-grade English-language learners’ (ELLs) understanding of properties of matter and chemical reactions at the molecular level – and compared it to non-ELLs. The students showed significantly improved understanding of both topics, and molecular visualisation seemed to be especially effective in learning chemical reactions and in the conservation of mass. There was no significant difference between the ELLs and the others, which suggests that visualisation can be useful for both groups.
The two remaining articles on technology-based learning environments explored data-logging activities and collective knowledge building, respectively. Deng et al. (Citation2011) investigated whether constructivist-oriented data-logging activities affected students’ conceptual understanding and metacognition. They found significant effects in both areas and that students with better metacognition showed better conceptual understanding. Finally, Chan et al. (Citation2012) investigated the role of collective knowledge building in promoting scientific understanding among high-school chemistry students in Hong Kong using a knowledge-building community. They found that the students who had used the knowledge forum performed better than the comparison group, both on the post-test and on the public examination one year later. They also saw that the students used several discursive moves, such as posing wonderment questions and working in an inquiry-based manner to write and revise explanations – moves that the authors argue are not common in traditional Hong Kong classrooms (Chan et al., Citation2012).
Authentic experiences
Eight articles in Group 2 involved students in authentic inquiry experiences. Five of these were placed in the affective domain, often in addition to another domain. Apedoe et al. (Citation2008) described a teaching unit (i.e. classroom practice) that combined engineering with scientific inquiry, where high-school students designed a heating or cooling system that relied on chemical energy and met a personal need. The students significantly increased their understanding of the relevant topics in chemistry in the conceptual domain – and also increased their interest in engineering careers in the affective domain.
Still in the affective domain, Itzek-Greulich and Vollmer (Citation2017) compared a control group with three experimental groups taught through guided-inquiry lab work: one taught at school, one taught in a Science Centre Outreach Lab visit and one taught in a combination of the two. They found that the students in the experimental groups displayed more favourable emotions during the activity than those in the control group. Among the experimental groups, different conditions favoured different parts of the lab work. The school group favoured higher enjoyment of the theoretical part, while the combined setting favoured higher situational competence in the practical part (Itzek-Greulich & Vollmer, Citation2017). In the affective and conceptual domains, Duffin et al. (Citation2016) explored how a three-week intervention in the form of an authentic, inquiry-based chemistry project where students connected chemistry to real-world consumer products affected the students’ performance, utility value, interest and intentions to study chemistry in the future. They found that the students improved their content knowledge, found it relevant and indicated greater intentions to study chemistry in the future. However, there was no significant increase in interest in chemistry.
In the procedural domain, Blonder et al. (Citation2008) explored the levels of the inquiry questions students asked when working with an open inquiry related to gas chromatography in a science laboratory centre. They divided the students’ questions into three levels: questions about subject matter (level 1), questions related to scientific equipment or methods (level 2) and questions related to real life (level 3), and found that 80% of the inquiry questions were at level 1 and 6% were at level 3. Furthermore, they compared the questions to the students’ level of achievement and found a correlation between their level of achievement and their level of inquiry questions. The average and below-average students only asked level 1 questions, whereas the excellent students asked higher-level questions (Blonder et al., Citation2008).
The remaining four studies were conducted by van Rens and colleagues, who, in several studies, developed design principles for authentic chemistry experiences related to the category of classroom practice. In van Rens et al. (Citation2013), they proposed six design principles to bridge the gap between inquiry practices in secondary school and scientific research practice:
(1) serious partnership in the process of knowledge development, (2) knowledge development occurs by doing scientific research, (3) key questions in the respective science domain are central, (4) acquaintance with collaboration and communication in a research community, (5) critical judgement by reflection, and (6) access to scientific resources and apparatus. (p. 1015)
They found that all of the design principles were necessary to bridge the gap, but some of them were difficult to achieve. They further investigated what changes occurred with content knowledge, the understanding of NOS and interest in scientific research after participating in the authentic experience, and found increases in all three domains (i.e. conceptual, epistemic and affective).
In van Rens et al. (Citation2014), they investigated the level of understanding of quality in inquiry for secondary students when they conducted peer reviews, and the study was therefore placed in the epistemic domain. They found that the understanding was good relating to the presentation of the results, discussion and conclusion, but that it was poorer on inquiry questions and the reliability of the results. van Rens et al. (Citation2004) developed an authentic research task for students on ‘Diffusion of ions in distilled water’, with a focus on the concepts of evidence. The students conducted a guided experiment, analysed a research article, conducted their own experiment, wrote a report and had a peer discussion focusing on the concepts of evidence before rewriting their reports. The authors conclude the article by arguing, ‘The authentic research problem, followed by the need-to-know principle, as integrated in the design (…) has stimulated students to carry out deeper and more complete learning activities than we have seen in previous studies’ (van Rens et al., Citation2004, p. 506). Finally, van Rens et al. (Citation2010) constructed a framework for teaching scientific inquiry in upper secondary education. The framework is based on two design principles: 1) ‘guiding students to conduct a cyclic and iterative inquiry process’ and 2) ‘creating an inquiry community’ (p. 789). After regular discussions with five teachers over three years and testing with 80 students, they concluded the framework. Based on the first design principle, they concluded that the framework should support willingness (an authentic problem and freedom, but scaffolding when planning), knowing (relevant subject knowledge and knowledge about the equipment, accuracy, reliability and validity) and ability (practicing the different parts of scientific inquiry, such as formulating questions, handling equipment and data, etc.). Based on the second principle, they concluded that an inquiry community contains four components: an inquiry problem (an authentic problem that is common for the class and unknown to the learners and teacher); letting the learners produce inquiry results; organising a critical discourse; and stimulating them so that the learners develop new knowledge and ask further questions.
Games and comics
The final three articles in this group used games and comics as an approach to IBCE. Two of these articles described the development of computer games for learning chemistry (Chee & Tan, Citation2012; Srisawasdi & Panjaburee, Citation2019), while the latter investigated the use of comic-based lab instructions in a non-formal inquiry-based laboratory learning environment (Affeldt et al., Citation2018). Srisawasdi and Panjaburee (Citation2019) developed a computer game called the Factory Game and compared 11th-grade students’ learning about the properties of liquids through the game to students who were taught by regular IBCE. They found that both groups had a significantly increased conceptual understanding (although there was less than a 50% score in both groups), but the game group scored significantly higher than the regular group. The game group also showed higher motivation in terms of intrinsic motivation, self-determination and self-efficacy. Similarly, Chee and Tan (Citation2012) designed and developed the educational game Legends of Alkhimia to foster chemistry learning through inquiry. The game allows the students to engage in problem solving and a virtual lab and hence use chemistry in realistic contexts. They found that the game fostered an inquiry culture in the classroom of 13-year-olds and noted significant shifts in the students’ epistemological beliefs, separation effectiveness and conceptual understanding. Finally, in the affective domain, Affeldt et al. (Citation2018) found that comics had the potential to increase both learning motivation and personal engagement among students in the 6th grade.
Summary of the articles in group 2
To sum up, the articles testing specific learning environments were quite varied in terms of domains. There were still many articles in the conceptual domain (N = 15), but the remaining domains were also strongly represented (affective domain = 9, procedural domain = 6, classroom practice = 5, other = 4, epistemic domain = 4 and social domain = 3). The four Group 2 articles in the category ‘other’ were studies from the technology-based learning environment focusing on metacognition, drawing and power relations. The articles in the epistemic domain were related to authentic experiences (N = 3) and games (N = 1).
When it comes to methodology, there was an excess of quantitative studies (N = 15) and they were mainly conducted in the United States (N = 8), Western Europe (N = 6) and Asia (N = 5). Another feature to bring forward from this group is that the studies typically had many respondents: 15 of the articles had 80 respondents or more, and 6 of the articles had more than 500 respondents. Both the quantitative and qualitative studies had quite a good number of respondents. Furthermore, the quality of the experimental studies was better than those in Group 1; half of the studies with a level 3 control group (i.e. A1-A4) was placed in Group 2 and the majority of the studies described comparison conditions when relevant and reported effect size.
Group 3: articles reporting on teachers
Group 3 included articles that reported on teachers’ use of IBCE. The articles were grouped into two sub-categories with respect to whether the studies addressed teachers’ practices or views. The results of the analysis are given in .
Table 8. Overview of the articles categorised in group 3 according to methods and categorisation.
Teachers’ practices
Fifteen of the included articles were studies investigating the inquiry practices of teachers, and several of these (N = 9) were thus categorised as ‘classroom practice’. Three of these articles focused on teachers’ classroom practices after participating in professional development initiatives. These articles typically introduced the teachers to specific teaching models that they later applied with their students. In the United States, Yezierski and Herrington (Citation2011) and Herrington et al. (Citation2011) explored how participation in a professional development programme known as Target Inquiry (TI) influenced chemistry teachers’ practices. The teachers were part of a 2.5-year programme where they first worked with educational research for a time period of six weeks before teaching the following school year. Thereafter, they used their research experience and literature to modify existing material that they revised after piloting the activities in the classroom. Yezierski and Herrington (Citation2011) found that it took two years of TI for observable gains to be detected among the 18 participating teachers. This is in line with Herrington et al. (Citation2011), who found that changes in practice did not occur for all eight teachers in their study before they had worked to adapt the material themselves. Herrington et al. (Citation2011) also investigated how changes in practice corresponded to changes in knowledge and beliefs about IBCE in the affective domain, and they found that both the teachers’ practices and their beliefs changed, but that two teachers were actually ‘less able to distinguish between inquiry and non-inquiry activities’ (p. 82).
In Finland, Juntunen and Aksela (Citation2013a) worked with 20 teachers over two years in order to improve the quality of sustainability education by combining life-cycle analysis (LCA) with IBCE. Through the course, they developed a framework for LCA project work consisting of four steps: 1) familiarising the students with the topic; 2) allowing the students to work in groups following an inquiry pattern (choose a product, make up questions, search for information, collect answers, opponent another group, improve their own work, make a presentation and prepare questions for their opponent group); 3) giving presentations with opponent groups; and 4) engaging in a summary discussion or debate. The study revealed that LCA could be used at all school levels, but that students’ learning outcomes needed to be further investigated.
Still in the category of classroom practice, Li and Arshad (Citation2015) investigated inquiry practices of Malaysian chemistry teachers using a video study. They found that the teachers mostly asked closed questions and typically followed the students’ answers with the correct answer and explanation. The group activities were mostly cookbook experiments related to verbal interaction. Overall, this indicated that inquiry teaching had not been implemented, and the authors suggested the inclusion of an inquiry model based on verbal interaction consisting of the triangle of multiple representations of chemistry teaching (i.e. sub-micro, macro and symbolic), the five features of inquiry teaching (i.e. students asking questions, giving priority to questions, formulating explanations, connecting explanations to knowledge and communicating knowledge) and inquiry-based verbal interactions (i.e. open-ended questions, sequences of verbal interactions after the questions and students’ responses, and a student-centred teaching pattern with the teacher as a facilitator). In China, Wang et al. (Citation2014) examined two teachers’ enactments of scientific inquiry and what factors influenced their enactments. The first teacher taught the topic air (composition, oxygen, carbon dioxide and combustion) in grade 4 and engaged the students in all phases of the inquiry (i.e. asking questions, making hypotheses, making plans, collecting evidence and drawing conclusions) in an explicit way to show students how to do and experience inquiry. The second teacher taught the topic acids and bases in grade 9 and placed more emphasis on IBCE than on learning inquiry. The teaching focused on solving problems in an authentic context and emphasised ways of making plans when solving authentic problems. The authors found that the enactment was influenced by teachers’ understanding of inquiry, textbooks, students, assessment and resources.
The last four studies, categorised as classroom practice, were conducted in the United States. Tolentino et al. (Citation2009) explored two teachers’ implementation of SMALLab (i.e. Situated Multimedia Arts Learning Laboratory) in an acid – base titration with their grade 10 students. They found that the teachers were more successful in framing learner-centred environments in SMALLab than during regular teaching and argued that this could be due to the active collaboration between the teachers and the scaffolding in SMALLab that framed student – teacher relationships and student discussions. The students also showed significant learning gains. Patchen and Smithenry (Citation2014) followed a chemistry teacher in her day-to-day work throughout a year, teaching chemistry in a total of three classes (grades 10–11). The authors used cultural historical activity theory to analyse how the teacher used traditional teaching methods to scaffold IBCE in order to explore the practical integration of more student-directed inquiry. In another article, Smithenry (Citation2010) presented a year-long case study of a high-school chemistry teacher’s implementation of guided inquiry. The author concluded that the integration of guided inquiry goes beyond the actual inquiry activity, and also includes the introduction to and the departure from the activity, and warned that extra time spent on inquiry could be at the expense of other parts of teaching. Finally, Criswell and Rushton (Citation2014) identified patterns related to classroom practice by analysing 14 inquiry-based lessons in the classrooms of five high-school chemistry teachers. They found that there were two general patterns across the lessons. The expansive pattern displayed by two of the teachers was characterised by an exploration of students’ ideas in small groups, no clear links between ideas generated by the students, discussing the validity of these ideas before testing them and that the solution was seldom connected to the students’ proposals. The open pattern, on the other hand, had the majority of explanations in the whole-class mode, where the teacher challenged the students to elaborate on their ideas before testing them (i.e. ties between the empirical and logical analyses) and the solution was connected to both the correct and alternative proposals.
Three of the remaining articles were categorised into epistemic or social domains. In the epistemic domain, Strippel and Sommer (Citation2015) interviewed 14 German secondary school teachers to explore how they incorporated learning about scientific inquiry into laboratory work in chemistry. They found that the nature of scientific inquiry was not a primary focus of the teachers but that some aspects were reconcilable with the laboratory work in chemistry. Furthermore, they found that the teachers holding PhDs made regular links between the topic and their own research experience, but at the same time, they struggled to include it in their teaching. In the social domain, McNeill (Citation2009) and McNeill and Krajcik (Citation2008) focused on how middle school teachers facilitated students’ argumentation in chemistry. McNeill and Krajcik (Citation2008) explored 13 teachers’ instructional practices when introducing scientific explanations. The instructional practices involved defining scientific explanations, making the rationale of scientific explanations explicit, modelling scientific explanations and connecting scientific explanations to everyday explanations. The authors found that the teachers varied in their practices when it came to both types of practice and quality of use. They also found that these practices influenced students’ ability to construct scientific explanations (McNeill & Krajcik, Citation2008). In the other study, McNeill (Citation2009) investigated instructional practices more in depth by exploring the support that six teachers gave to their students when writing scientific arguments. She found that the teachers’ definitions of scientific argumentation differed and that their definitions affected their classroom practice, which in turn affected the learning gain (McNeill, Citation2009).
The final three articles were categorised as ‘other’ and two of these focused on assessment. Bernard et al. (Citation2019) conducted a case study to explore how experienced chemistry teachers with a positive attitude towards IBCE integrated inquiry-based teaching with formative assessment. They found that the inquiry integration was done satisfactorily by the two teachers involved, but that the integration of assessments was challenging, especially when attempting to do so in a formative way. This was linked to the lack of experience of the teachers and to contextual issues related to formative assessment being undermined in the country of study (i.e. Poland). Harshman and Yezierski (Citation2015) interviewed 19 chemistry teachers to identify how they used assessment and classroom data to inform their teaching. They found limitations in the teachers’ practices when they examined them through a process called data-driven enquiry: the learning goals were not specific and conducive to informing instruction; the teachers based their evaluation solely on test scores, presuming that a correct answer equalled understanding; the conclusions were mainly focused on students and their learning, rather than on the teacher’s instructions; and few teachers specified concrete actions based on the analysed data. In the final article, Gao and Wang (Citation2014) examined whether the establishment of centralised curriculum standards and school-based teacher learning communities influenced two teachers’ inquiry-based teaching. They found that the curriculum standard and relevant material did not assist the teachers’ practice change due to limited experience and knowledge, limited time and a discrepancy between the new curriculum and the assessment system. District support was not found useful by any of the teachers, either. However, during the two years, the participants developed differently in both understanding and practice. One of the teachers embraced the inquiry method and engaged the students in asking questions and developing experiments. She argued that IBCE was a better method to teach the content and perceived the goal of the teaching to be both learning chemistry and understanding the process. This teacher was also closely supported in her school chemistry teaching research group, where they collaboratively developed teaching lessons and benefited from observing and discussing other teachers’ lessons. The other teacher, on the other hand, did not fully understand IBCE and found it to be less effective than traditional teaching. Her school chemistry teaching research group allowed her to teach as she used to and the group spent more time complaining about problems they encountered (Gao & Wang, Citation2014).
Teachers’ views
Five articles focused on teachers’ views and beliefs and were hence placed in the affective domain. In Israel, Peleg et al. (Citation2017) investigated chemistry teachers’ views on using storytelling in connection with mysteries in the science classroom and the factors promoting and hindering implementation. They found that even though it could enrich inquiry learning, there was a reluctance among some of the teachers to use fictitious stories. This was both related to their image and to the telling of stories that were not entirely true. In Hong Kong, Cheung (Citation2008) investigated seven chemistry teachers’ major concerns about implementing inquiry-based laboratory work in their schools and strategies that could be used to reduce these concerns. The three most common concerns were a lack of time, a lack of inquiry learning material and large class sizes. The author suggested several strategies to assuage each of these concerns, such as specific material and designing shorter inquiry activities. In a later article, Cheung (Citation2011) developed a guided-inquiry scale to measure teachers’ beliefs about implementing guided-inquiry labs in secondary schools and how their beliefs differed. The scale had three dimensions: the value of the guided-inquiry labs, limitations of regular labs (i.e. cookbook style) and issues with implementing guided-inquiry labs. The items were first tested with 50 teachers and, thereafter, 150 teachers. The second sample consisted of both users of guided inquiry (N = 111) and non-users (N = 39). The items provided valid and reliable data.
Two of the articles were categorised within classroom practice as well. Roehrig and Luft (Citation2004) explored factors influencing the classroom practices of 10 beginning secondary chemistry teachers in the United States. Only five of the teachers were observed implementing inquiry activities, and only one of the teachers used it frequently. The authors found a connection between teaching beliefs and classroom practice; teachers with more constructivistic teaching beliefs included more IBCE. When it comes to content knowledge in chemistry, there was an opposite effect. The three teachers with the highest degrees in chemistry had the most traditional teaching, and the authors argued that this was due to them teaching as they themselves had been taught. The final factor was the presence of teaching and learning resources, which was especially needed by the science teachers without a background in chemistry. Donnelly et al. (Citation2013) conducted case studies investigating four teachers’ perceptions of their implementation of inquiry-based approaches and their reasoning underpinning this when using virtual chemistry laboratories in guided inquiry. They found that the use of virtual laboratories could overcome many of the ‘typical’ challenges teachers face, such as time, safety and resource demands, allowing the teachers to focus instead on analysing the findings and on students’ learning. Furthermore, it allowed for a shift in focus from procedures to more problem-solving – and a more authentic picture of science. However, the teachers also pointed out some challenges related to classroom management, such as distracted students and students giving up on tasks, emphasising the need to address the culture of the classroom (Donnelly et al., Citation2013).
Summary of the articles in group 3
Many of the studies in this group were from the United States (N = 10) and, as with the prior groups, they were mainly conducted at secondary level (N = 18). The articles in this group differed from those in the other groups in several ways, since the focus was on teachers rather than on students. They differed in terms of the methodology applied, as most of the articles followed a limited number of teachers in qualitative studies. Moreover, in the case of the mixed studies, they were more qualitative in character, and only three of them were experimental studies, all without a control group, but reporting effect size (i.e. D1). When it comes to categorisation into domains, many studies were, in line with the other groups, categorised in the affective domain. However, one difference was that many studies were categorised as classroom practice, describing how IBCE was implemented in the classroom. The category ‘other’ included three articles focusing on assessment (N = 2) and teachers’ experiences (N = 1).
Group 4: additional relevant studies
Group 4 consists of the 28 remaining studies that were not covered by Groups 1–3, but that were still relevant for this review article. The group is presented with a focus on domains; articles that were categorised into more than one domain are presented in the domain where the emphasis of the article is placed. The results of the analysis are given in .
Table 9. Overview of the articles categorised in group 4 according to methods and categorisation.
Conceptual domain
As with the prior studies focusing on students (i.e. Groups 1 and 2), many studies in Group 4 investigated the effect of IBCE on students’ understanding and were therefore placed in the conceptual domain (N = 12). Two articles were placed in the conceptual domain only. Doucerain and Schwartz (Citation2010) investigated the effect of guided inquiry and argumentation on 8th-grade students’ conceptual understanding of the conservation of matter. They found that guided inquiry led to a significant gain in students’ understanding but was less effective than argumentation sessions. The second article was from Sola and Ojo (Citation2007), who compared the effectiveness of three methods called the project method, the lecture-demonstration method and the inquiry method for teaching experimental aspects of chemistry with the ‘separation of mixtures’ topic in chemistry. Most studies in this review reported positive conceptual outcomes from IBCE, but a couple of articles, including this one, differed by reporting on studies where IBCE had poor outcomes. They found that students taught with the inquiry method performed poorer than those taught with either the project- or lecture-demonstration methods. However, an interesting question here was what type of inquiry the students conducted, as in their introduction section, the authors concluded with the following description of the inquiry method: ‘inquiry is the way people learn when they’re left alone’ (Sola & Ojo, Citation2007, p. 125). Furthermore, the four treatment groups (i.e. lecture-demonstration method, project method, survey method and control group) were from four different schools (one group in each school), which makes it more difficult to control variables and rule out the influence of the school/teacher. The authors also mentioned limitations related to the inquiry group, such as a lack of experience and equipment.
The remaining studies were categorised in another domain as well, but five of them are presented here, since the articles emphasised conceptual learning. In three of the articles, the conceptual domain was combined with the affective domain. Sesen and Tarhan (Citation2013) investigated the effects of inquiry-based laboratory activities on students’ understanding of electrochemistry and attitudes towards chemistry and laboratory work and found a significant increase in both areas compared to the control group. Özgür and Yilmaz (Citation2017) examined the effect of guided IBL on gifted grade 8 students and found a significant difference both with respect to their understanding of acids and bases and their motivation towards science. Chairam et al. (Citation2015) included IBCE in order to include more student-centred learning in Thailand and investigated the effect on students’ understanding of kinetics and science process skills and their attitudes towards IBL activities (i.e. conceptual, procedural and affective domains). They found that the students enjoyed IBCE and that they made learning progress in some areas, but not all.
Finally, in some of the articles, the development of specific teaching sessions was described, and these were placed in classroom practice in addition to the conceptual domain. Kimberlin and Yezierski (Citation2016) designed two inquiry-based lessons to facilitate students’ conceptual understanding of stoichiometry; the lessons resulted in a significantly increased understanding when students from both grades 11 and 12 were tested. Bridle and Yezierski (Citation2012) described the implementation of inquiry-based, particulate modelling experiences and investigated their effectiveness at improving students’ conceptual understanding of chemistry. The study was a pilot study without a control group, but showed positive effects within this population.
Epistemic domain
Two articles were placed in the epistemic domain. In Bhutan, Das et al. (Citation2018) investigated how an explicit focus on NOS influenced students’ views on the topic. They found that the students’ views changed from naïve to more informed when considering NOS. In Zimbabwe, Vhurumuku (Citation2011) investigated the relationship between students’ scientific epistemologies and their perceptions of the nature of laboratory inquiry. They found that most students had quite traditional views of NOS and perceived the level of inquiry to be low.
Social domain
Five articles were categorised in the social domain. Three of these focused on argumentation and are described here, whereas the remaining two focused on the presentation of data in relation to the procedural domain and are described in the next section. Katchevich et al. (Citation2013) explored how high-school chemistry students’ argumentation skills differed in confirmatory and open-ended experiments. They found that the students developed fewer arguments in the confirmatory experiments and that the arguments were also of lower quality. The authors further explored the students’ argumentation across experiments by comparing the hypothesis stage with the analysis and conclusion stages and found that the arguments were better in the analysis and conclusion stages. Sampson and Clark (Citation2009) examined the value of collaboration when producing scientific argumentation and found, much to their surprise, that arguments produced by groups of students in terms of quality were equal to the arguments from the middle-performing individuals. However, the students working in groups learned ‘both from and about scientific argumentation’ (Sampson & Clark, Citation2009, p. 473) when they engaged in a task that required them to evaluate alternative explanations and create an argument that justified the explanation. Finally, Juntunen and Aksela (Citation2014) investigated how students used scientific, ecological, socio-economical and ethical argumentation connected to a project where they conducted LCAs of products. They found that the students used socio-economical argumentation to a similar extent both before and after the project, but that the use of scientific and ecological arguments increased during the project. When it comes to ethical argumentation, it was low both before and after the project.
Procedural domain
The articles categorised in the procedural domain (N = 12) focused on different phases of the inquiry process. Some were connected to the first phases of inquiry, such as three articles from Israel that focused on the learners’ ability to ask researchable questions. Hofstein et al. (Citation2005) investigated students’ ability to ask questions both during experiments and after reading scientific articles. They found that the students in the experimental inquiry group asked higher-level-type questions about the experiment and also asked more questions in general after reading the articles. Furthermore, they found that the students in the inquiry group displayed a different attitude towards the tasks and devoted more time and attention to the questionnaires. Dkeidek et al. (Citation2011) studied the effect of different cultures of student – teacher interaction on students’ questions. The study was conducted with 12th-grade students in the Arab and Jewish sectors of Israel. They found that the students from the Jewish sector tended to work independently with the teacher as a guide, whereas the students from the Arab sector were highly dependent on their teachers in formulating research questions. Thus, the authors concluded that ‘cultures, traditions, norms, social structure, modes of living, and related factors play a significant role’ (Dkeidek et al., Citation2011, p. 1326) and such factors must be taken into consideration. Similarly, Blonder et al. (Citation2015) investigated how an inquiry-based chemistry laboratory programme affected the questions of students from different sectors in Israel (i.e. Arabic and Jewish, both religious and secular) – and whether there was gender-differences. As opposed to earlier studies, they found the differences to be minor and inconsistent. When it comes to the gender differences, girls were found to ask more questions, but otherwise, there were no significant differences (Blonder et al., Citation2015).
Still in the planning phase and based on a claim that this phase is typically neglected, Neber and Anton (Citation2008) investigated the cognitive and motivational effects of explicitly focusing on and supporting cognitive activities in this phase (i.e. observation, accessing prior knowledge, raising questions, anticipating answers and planning for evidence). In their study conducted in Germany, they found that the experimental group had stronger preferences for open experiments, more intense reflections and were better at formulating questions. In Spain, Crujeiras-Pérez and Jiménez-Aleixandre (Citation2017) investigated what actions students included when designing their experiments and the patterns in the students’ experimental design over two consecutive school years (grades 9 and 10). The actions included in the design by groups of students were selecting materials and equipment, deciding on the measurement criteria, proposing a procedure, and considering fair testing and reproducibility. Even though all of the actions were not considered by all groups of students, they showed progress throughout the two years. In Hungary, Szalay and Tóth (Citation2016) proposed a model for IBCE in which, in the introduction to a topic, students first performed a step-by-step experiment and thereafter designed an experiment by modifying the first experiment in order to answer a given question (e.g. the effect of different factors on the rate of reaction). This model was tested by implementing three lesson plans based on the model in 12 schools in order to explore 9th-grade students’ ability to design experiments and their development of content knowledge of the topic. The authors found changes in their ability to design experiments that were higher in the experimental group compared to the control group. However, when it came to content knowledge, there was no significant difference, and some students in both groups even had poorer results on the post-test compared to the pre-test. As a follow-up, Szalay et al. (Citation2020) tested the model with younger students (grade 7), but with poor results. They found a weak positive effect on content knowledge in one of the groups, and no effect in terms of designing experiments and attitudes towards chemistry.
The remaining four studies presented in this section focused on experiments and the process of drawing conclusions from the data. Three of these studies covered other domains in addition to the procedural domain, such as the two articles mentioned earlier, which focused on different aspects of how students discussed or presented their data, and these were placed in the social domain in addition to the procedural domain. van Brederode et al. (Citation2020) investigated the effect of the pre-laboratory activities of an inquiry assignment on students’ critical thinking. The students were supposed to distinguish between different reaction mechanisms for a substitution reaction and were divided into two groups: the students in Group 1 answered pre-laboratory questions and were provided with laboratory instructions, while the students in Group 2 designed an experimental plan and got a hint on starting combinations for the experiment. Otherwise, the experiments were equal. The students’ critical thinking was measured in their reports, focusing on a comparison between the data and models, representing the extent to which the students had considered the meaning of their experiments and had understood what had been observed. The results showed that, to a larger extent, the students given the critical thinking pre-laboratory activity tried to understand unexpected observations and thought ‘more deeply about the meaning of their measurements’ (van Brederode et al., Citation2020, p. 1173). In the other study related to the social domain, Hug and McNeill (Citation2008) compared the quality of classroom conversations when students analysed data that they had collected themselves (i.e. first-hand data) compared to their analysis of second-hand data. They found that the two types of data had both benefits and limitations and could be used for different purposes. First-hand data offered opportunities to discuss how data should be collected and organised and to discuss data limitations, whereas the students more frequently discussed patterns and drew conclusions based on the second-hand data. Thus, the authors argued that both types of data are important when developing skills in scientific inquiry and what data to use depends on the learning goal.
The two final studies focused on inquiry skills. Nehring et al. (Citation2015) investigated what the cognitive, motivational and socio-demographic characteristics of students were that contributed to their scientific inquiry skills. They included a total of 12 covariates and found that, to a large extent, the characteristics predicted their inquiry skills. In particular, their cognitive variables predicted their inquiry skills, but the motivational variables were also important. Finally, Tornee et al. (Citation2019) examined the effectiveness of traditional versus guided inquiry on students’ problem-solving competence and chemistry learning. They found that the guided-inquiry group increased their skills more than the other group did, and argued that the increase could be due to the design, as the teachers were more flexible in their supervision and the students engaged more with the problems and collaborated more.
Affective domain
Eight articles were categorised in the affective domain, but seven of these focused on other domains and are presented elsewhere. The only Group 4 article emphasising the affective domain is an article where Juntunen and Aksela (Citation2013b) investigated how an inquiry-based LCA project affected grade 9 students’ attitudes towards chemistry and their environmental literacy. The authors found that the students’ attitudes towards chemistry increased and their impressions about chemistry changed from the subject being non-useful to arguing that they learned beneficial things. When it comes to environmental literacy, the students’ awareness increased, but half of them also expressed that it would not change their behaviour.
Classroom practice
Four articles were placed in the category of classroom practice, and one of them focused mainly on the teaching activity, which is described here. In Sweden, Högstrom et al. (Citation2010) studied the teacher’s objectives for lab work and how these affected the learning experiences of the students. They found that teacher – student interactions, both through what the teacher said and how she acted, were particularly important, as this guided the students in what they were supposed to learn from the lab work. The authors concluded the article by suggesting the following:
a laboratory exercise for secondary school students should not include too many aspects. Some aspects, like procedural skills and skills to interpret observations, ought to be dealt with often. Other learning experiences for students, e.g. to plan experiments, should be provided in laboratory exercises designed for such purposes. (Högstrom et al., Citation2010, p. 520)
Other
Of the 28 studies in Group 4, six of them were placed in the category ‘other’. Three of these have already been mentioned in prior categories and they focused on students’ environmental literacy (Juntunen & Aksela, Citation2013b) and the effect of different cultures of student – teacher interaction on students’ questions (Blonder et al., Citation2015; Dkeidek et al., Citation2011). Dkeidek and colleagues explored the differences between Arab and Jewish students in another study as well (Dkeidek et al., Citation2012). They explored the students’ perceptions of the laboratory classroom learning environment in an inquiry-oriented chemistry laboratory and found that the student – teacher relationship and the pre-inquiry phase differed.
The two final studies focused on students’ metacognitive skills and learning styles, respectively. Kipnis and Hofstein (Citation2008) investigated the potential of a lab programme that involved all inquiry phases on Israeli grade 12 students’ metacognitive skills. They used two different models for analysing the data, one that focused on cognition that differed between their knowledge of cognition (declarative, procedural and conditional) and their regulation of cognition (planning, monitoring and evaluating) that was relevant for the inquiry activity – and another with components that can be expressed in conversations (Kipnis & Hofstein, Citation2008). The authors found that the students practiced different parts of metacognition during the inquiry process, both in the specific activities, such as asking and choosing questions, performing experiments and drawing conclusions – and throughout the activities. Sudria et al. (Citation2018) identified students’ learning styles when working with inductive guided-inquiry learning and compared these to their achievement. They found that guided-inquiry learning was fruitful for all types of learners, but that the learning styles significantly affected their achievement.
Summary of the articles in group 4
The articles in Group 4 were quite similar to the studies in Groups 1 and 2. The studies were mostly quantitative or mixed with a quantitative character, and in line with Group 1, some studies had firm conclusions based on a few respondents. However, there were also several studies with more than 100 respondents (N = 11) and the quality of the studies was in general better than those in Group 1, as can be seen from the typology. There were only four studies without a control group, and three of these reported effect size (i.e. D1). There were also seven studies with level 3 control group, and only three studies that did not described the control conditions (A4 and B3).
The studies in Group 4 differed from the other groups in terms of geographical distribution and, to some extent, also age and their categorisation into domains. When it comes to the origin of the studies, there were six studies from Israel and four from the United States, but otherwise, the studies were conducted in diverse countries. Regarding age, in total, there were five studies from grades 7 and 8, which was half of the studies from grades 6–8. Finally, when it comes to the domains, the conceptual and procedural domains were strongly addressed (both with N = 12). There were also several articles categorised in the affective domain (N = 8), the social domain (N = 5), classroom practice (N = 4) and ‘other’ (N = 6). The articles in the ‘other’ category addressed cultural issues, learning styles, metacognitive skills and environmental literacy.
Discussion
The aim of this study was to examine research on IBCE in primary and secondary schools and to discuss how it is addressed in the research literature. The analyses revealed a relatively constant publication rate since 2008. These studies were conducted with varied foci, represented by the categorisation into groups and domains. In line with the RQs, this section will include a discussion of the most prominent findings reported in the reviewed articles. The findings will be discussed related to the distribution of the included articles according to domain, the learning outcomes from the studies across domains and some overall characteristics of the reviewed articles.
Distribution of articles according to domain
Looking at the distribution of the articles according to domain, almost half of the included articles (N = 46) were categorised in the conceptual domain. This is in line with the study by Breslyn and McGinnis (Citation2012), who found that chemistry teachers tended to focus on content knowledge when enacting inquiry-based education. Moreover, even though the topics addressed varied, traditional topics, such as electrochemistry, acids and bases, the particulate nature of matter and stoichiometry were frequently addressed. Thus, the focus seemed to be on ready-made science, and the students were, to a minor extent, allowed to study science-in-the-making (Osborne, Citation2010), allowing them to understand the uncertainty of knowledge and why scientists occasionally disagree. The only exceptions to the traditional topics were studies focusing on chemistry in real-world consumer products (Duffin et al., Citation2016), including LCA (Juntunen & Aksela, Citation2013a, Citation2013b, Citation2014). Furthermore, most studies, except the articles related to PBL and real-world consumer products, seemed to focus mostly on the sub-micro level of chemistry. Students often struggle to make connections between the sub-micro and macro levels, which is important for learning chemistry (Talanquer, Citation2011). As a response, Sarıtaş et al. (Citation2021) suggest to also include observations at the macro level before modelling and drawing preliminary conclusions at the submicro level, in order to articulate the relationship and help the students move across the levels.
There were also many studies in the affective (N = 37) and procedural (N = 21) domains. Studies in the affective domain were almost as frequent as studies in the conceptual domain and were often addressed in combination with this domain. These studies typically focused on students’ perspectives on the teaching activity or how their views on or motivation for chemistry changed as a result of participating in the activity, mostly with positive results. In the procedural domain, several studies focused on the quality of students’ inquiry questions, finding that participation in inquiry led to better questions (e.g. Hofstein et al., Citation2005; Neber & Anton, Citation2008), but that the achievement level of the students (Blonder et al., Citation2008) and their culture (Dkeidek et al., Citation2011) affected their ability to pose questions. There were also studies focusing on students’ ability to plan experiments after participating in IBCE and the development of students’ practical skills, including graphical skills and modelling skills, which showed positive results (e.g. Dori & Sasson, Citation2008; Kaberman & Dori, Citation2009b; Smith, Citation2012; Szalay & Tóth, Citation2016).
The epistemic and social domains were less frequently addressed in research on IBCE. These results are similar to the results of a recently conducted literature review on inquiry-based science education in teacher education (Strat et al., Citation2023), indicating a trend in science education in general. The epistemic domain was addressed in eight articles, including three articles focusing on authentic science experiences to give students experiences in the epistemic domain (van Rens et al., Citation2004, Citation2013, Citation2014), and two articles finding that an explicit focus on NOS was beneficial for students’ conceptions of NOS (Das et al., Citation2018; Eymur, Citation2019). The social domain was addressed in 14 articles, including articles focusing on students’ argumentation related to scientific quality (Sampson & Clark, Citation2009; Sampson et al., Citation2011), chemical representations (Stieff, Citation2011) and the content of their arguments (Juntunen & Aksela, Citation2014), students’ writing skills (Çetin & Eymur, Citation2017; Çetin et al., Citation2018) and discussions and the presentation of data (Hug & McNeill, Citation2008; van Brederode et al., Citation2020).
A limited focus on the epistemic and social domains can be perceived as problematic, given that science education needs to shift from its dominant focus on conceptual learning to a more balanced focus on learning, where epistemic and social learning goals are included as well (Duschl, Citation2008). Furthermore, according to Duschl (Citation2008), the scientific domains are intertwined, and knowledge developed in one domain is necessary in order to engage in the other domains. Thus, a limited emphasis on the epistemic and social domains may in turn affect the conceptual domain and science knowledge overall. Furthermore, the limited focus on the epistemic domain, combined with an emphasis on traditional knowledge in the conceptual domain, may give the students a limited picture of chemistry. This, in turn, can restrain the students and make them ‘only able to justify their beliefs by reference to the teacher or textbook as an authority’ (Osborne et al., Citation2001, p. 10).
Learning outcomes from IBCE
In line with previous studies on IBL (e.g. Furtak et al., Citation2012; Minner et al., Citation2010; Savelsbergh et al., Citation2016), the articles included in this review mainly reported favourable learning outcomes from IBCE. The authors point to the benefits of students being actively engaged in constructing explanations through IBCE (e.g. Chairam et al., Citation2015) and the social interactions both between the students, and the students and the teacher (e.g. Kingir et al., Citation2013). However, the positive results can also indicate a bias, where the experimental group is given favourable conditions compared to the control group – an issue returned to in the next section.
Different perspectives are emphasised to increase learning from IBCE. Högstrom et al. (Citation2010) emphasised focusing on some aspects of the inquiry process at a time, and that some aspects, such as procedural skills and interpretations of observations, should be included more often than other aspects. An explicit focus on specific elements also seems to be beneficial for learning. In line with the literature arguing that NOS needs to be addressed explicitly (Bell et al., Citation1998), the included studies reported that explicit NOS was beneficial for students’ conceptions regarding NOS (Das et al., Citation2018; Eymur, Citation2019). Based on the positive learning outcomes in the studies, it can also be concluded that the explicit focus on modelling skills or graphing skills or the specific parts of the inquiry process that the various teaching models provide affect the learning outcomes beneficially.
There were also studies emphasising the role of digital learning environments in students’ learning, as could be seen in Group 2. Some of these studies discussed virtual laboratories as alternatives to physical laboratories (e.g. Pyatt & Sims, Citation2012), providing student-centred learning environments. The highlighted benefits of virtual laboratories included more time to focus on the content, reduced challenges, such as safety and resource demands, and higher-quality data for the students to discuss and learn from (Donnelly et al., Citation2013; Pyatt & Sims, Citation2012; Tolentino et al., Citation2009). As part of the digital learning environment, visualisation tools were also found to be beneficial, especially when it came to the use of sub-micro representations (Stieff, Citation2011) and students’ understanding of chemical reactions, particularly when combined with drawings (Zhang & Linn, Citation2011).
Although most articles in this review concluded with positive learning outcomes from IBCE, there were also articles pointing to challenges related to the approach. In some studies, they found that the students were not used to or ‘ready for’ the method (e.g. Donnelly et al., Citation2013; Tarhan & Acar-Sesen, Citation2013; Tarhan et al., Citation2008), whereas other studies pointed to a lack of familiarity among the teachers, and that their implementation was affected by their pedagogical beliefs and their understanding of inquiry and the related concepts (Gao & Wang, Citation2014; Li & Arshad, Citation2015; McNeill, Citation2009; Roehrig & Luft, Citation2004; Wang et al., Citation2014). There were also studies focusing on how power relations in the classroom affected the implementation of IBCE and that both culture (Dkeidek et al., Citation2011, Citation2012) and the traditional expectations of the teacher – student relationship (Donnelly et al., Citation2014) could stifle IBCE. Finally, among the challenges, Smithenry (Citation2010) warned, in line with Teig et al. (Citation2018), that IBCE was a time-consuming method that may be included at the expense of other useful learning activities. However, this indicates an emphasis on conceptual learning instead of other types of learning, such as the learning of 21st-century skills and other learning aspects that are important and related to IBL. This coincides with the results of this review, with limited focus being placed on creativity, collaboration, critical thinking and other 21st-century skills.
Some characteristics of the reviewed articles
The studies included in this review were mainly conducted in the United States, Turkey and Israel, with a total of 52 of the 102 included articles, followed by Thailand and Germany with 6 and 5 articles, respectively. The limited geographical distribution may be a result of two methodological choices: search terms and language. Inspired by Dobber et al. (Citation2017), PBL was used as a search term in addition to IBL, since PBL is often used as a synonym for IBL and the aim was to include all articles related to IBCE, and not only articles that the authors had labelled as IBCE. The term enquiry was also included since it is widely used in the British language. However, the lack of articles from Australia and also the few articles from Western Europe may indicate that there were some studies that were not caught by the search terms and that used other terms (c.f., Crawford, Citation2014). Further, the choice of limiting the review to articles written in English may be an explanation for the relatively few articles from countries that work extensively with chemistry education (e.g. Germany and countries in South America and Asia). However, this was a deliberate choice as the aim was to review the research available for the wider research community. There might also be relevant articles that were not labelled with ‘inquiry’, which therefore have not been captured by the search.
Most studies in this review were targeted towards older students (grades 9 to 12). This can also be explained by the search terms, since ‘chemi’ had to be present in either the title, abstract or keywords. Thus, studies in chemistry embedded in science education might have been lost if they did not explicitly talk about chemistry, especially in the younger years. However, this also indicates that research is mostly conducted with older students. This is not surprising, given that prior reviews of research trends have shown that chemistry education research is primarily conducted in higher education. Teo et al. (Citation2014) found that 54.3% of the articles published between 2004 and 2013 had research participants from higher education, whereas participants from the primary (grades 1–6), lower secondary (grades 7–9) and upper secondary (grades 10–12/13) levels appeared in 4%, 14% and 25% of research articles, respectively. Similarly, Sozbilir et al. (Citation2016) found that less than 5% of the articles published between 1997 and 2013 related to primary schools, while 15–20% related to secondary schools.
From a methodological perspective, there is a large variation in the quality of the studies included in this review. Some of the studies were thorough and were published in highly reputable journals. However, several of the quantitative studies in particular were quite superficial, lacked crucial information (e.g. about how randomisation was achieved and control group conditions) and the number of respondents was typically low. Furthermore, there was an issue related to the design of the studies, as many studies were designed as what Taber (Citation2019) labels ‘rhetorical experiments’ (p. 107). In these studies, a teaching approach with a well-established effect is compared with ‘traditional teaching’. This is problematic, both from a methodological perspective and an ethical perspective. First, it is problematic because the teaching approach is tested against conditions that are known as being ineffective for learning, facilitating favourable conditions and the before-mentioned bias. Secondly, it is problematic because the students in the control group are expected to learn less than those in the experimental group, and perhaps less than usual, if the teacher limits his or her teaching (Taber, Citation2019).
There were also studies that did not have a control group (i.e. all studies labelled D1/D2) or had issues with the control group. These issues could be comparing two relatively small groups taught by different teachers or failing to describe the control groups’ teaching (i.e. the articles labelled A3/A4 or B3/B4) or favouring the experimental group in the post-test compared to the control group. With regard to the latter, there were some examples where the experimental group was assessed in a similar way in the post-test throughout the year, while the control group was not – or where the control group did not have any lessons on the topic under study. Finally, different interpretations of inquiry may also have consequences for the validity of the studies (Briggs, Citation2008; Furtak et al., Citation2012), since some authors may have had a limited understanding of IBCE. This might for instance be the case in the study from Sola and Ojo (Citation2007), referring to inquiry being ‘the way people learn when they’re left alone’ (p. 125). Some articles lacked information about the teaching activities, thus making any cases of limited understanding difficult to uncover during the review process.
Since interventions should go on for some time and include many teachers to reduce ‘the teacher effect’ (Cheung & Slavin, Citation2013), there seems to be a need for larger studies on the topic. However, it can be questioned whether experimental studies are the best way to explore conceptual understanding. Most of the included studies reported higher learning outcomes from IBCE than from traditional education, but as mentioned earlier, the value of comparing new teaching methods with active students and perhaps more engaged teachers with teaching employing traditional methods is questionable. Some authors (e.g. Tornee et al., Citation2019) take this into account, arguing that such positive results could also be due to teachers who are more flexible in their supervision and students who collaborate and engage more deeply in the problems. Taber (Citation2019) argues that such studies have a value if they are conducted in a specific and new context, but rather suggests conducting studies with level 3 control conditions. In this review, level 3 control conditions were conducted in 20 of the 66 experimental studies, and mostly related to group 2 about technology, where for instance one group used virtual labs, while the control group used physical labs. A study to highlight in this respect is the one from Pyatt and Sims (Citation2012), that both applied level 3 control conditions, and a cross-over design, where both groups experienced both treatments, another design move advocated in the literature (Taber, Citation2019).
Conclusions and suggestions for future research and practice
This article examined 102 empirical studies on IBCE in primary and secondary schools and discussed how it is addressed in the research literature. The analyses revealed that research on IBCE was conducted in a range of ways, with varied foci. Approximately half of the studies tested different teaching approaches, teaching models or learning environments, which resulted in the creation of Groups 1 and 2. The remaining studies were either articles in which teachers reported on their implementation of IBCE (Group 3) or other relevant studies focusing on IBCE that were not included in the first three groups (Group 4). Based on the results of this review, some suggestions for future research and practice will be given.
The review included many quantitative studies with older students (grades 9 to 12), often with few respondents and varied quality due to limited durations, random sampling and little attention being given to the teacher effect. Thus, in terms of empirical research, there is a need for more qualitative studies involving students – and not only teachers. There is also a need for carefully designed and thoroughly described quantitative studies with larger numbers of participants including at least two teachers in each group, to separate the treatment effect from the teacher effect (c.f., Cheung & Slavin, Citation2013). These studies should have level 3 control conditions, preferably with a cross-over design. Quantitative studies with a qualitative component are also suggested so as to achieve a better understanding of the concept under study (Creswell, Citation2014). Furthermore, more research into IBCE with younger students is needed, as it is important to study the initial learning of chemistry, as well as the more advanced learning. As a part of this, it could be interesting to conduct a similar review in primary education, including science as a search term, in order to capture studies in chemistry from primary schools that are labelled as ‘science’ rather than ‘chemistry’. Finally, as described above, there is a need to focus more on IBCE related to the epistemic and social domains, since the main emphasis in the research was on the conceptual and affective domains.
When it comes to suggestions for chemistry teaching, the varied teaching models and approaches available for IBCE illustrate several ways that IBCE could be included in schools; both the described models and approaches and the articles categorised as ‘classroom practice’ are suitable starting points for the development of teaching. However, since both the research discussed in this review and the actual activities largely focus on the conceptual domain, there seems to be a lack of emphasis on other domains, also in teaching. The students may have worked with several domains, while focusing on others – for instance, the epistemic domain while focusing on the procedural domain – but as discussed earlier and related to NOS, the implementation needs to be done explicitly in order for the students to see the connections (Bell et al., Citation1998).
Acknowledgments
This study is a part of the TRELIS-project, financed by the Norwegian research council. I would like to thank librarians at OsloMet for support in the literature search and colleagues at OsloMet and in TRELIS for commenting on drafts of the article.
Disclosure statement
No potential conflict of interest was reported by the author(s).
Additional information
Funding
Notes on contributors
Kirsti Marie Jegstad
Kirsti Marie Jegstad is a professor in science education at Department of Primary and Secondary Teacher Education, OsloMet – Oslo Metropolitan University. Her research interests include chemistry education, inquiry-based science education, critical thinking, education for sustainable development and research-based teacher education.
Notes
1. The age limit was set in accordance with the country’s school system. This means that the age of the oldest participants differs slightly since some countries have secondary levels up to the age of 18 (e.g. UK and USA), while others have students up to the age of 19 (e.g. Germany and the Nordic countries). There are differences in when children start their schooling as well, but this was not relevant to this article since no included studies were conducted with children of that age. Studies focusing on teachers were included if the focus was on the teachers’ reported practice in schools and not if the focus was on teachers themselves.
2. Several of the articles in the query presented teaching activities with some kind of reported results (from questionnaires or informal conversations). In these articles, the methods and results chapters were assessed in order to judge whether they could be categorised as research or just as evaluations.
References
- Abd-El-Khalick, F., Boujaoude, S., Duschl, R., Lederman, N. G., Mamlok-Naaman, R., Hofstein, A., Niaz, M., Treagust, D., & Tuan, H.-L. (2004). Inquiry in science education: International perspectives. Science Education, 88(3), 397–419. https://doi.org/10.1002/sce.10118
- *Abubakar, A. B., & Arshad, M. Y. (2015). Self-directed learning and skills of problem-based learning: A case of Nigerian secondary schools chemistry students. International Education Studies, 8(12), 70–78. https://doi.org/10.5539/ies.v8n12p70
- *Adesoji, F. A., & Idika, M. I. 2015. Effects of 7E learning cycle model and case-based learning strategy on secondary school students’ learning outcomes in chemistry. Journal of the International Society for Teacher Education. Vol. 19. 1. 7–17 (EJ1177065. ERIC. https://eric.ed.gov/?id=EJ1177065
- Aditomo, A., & Klieme, E. (2020). Forms of inquiry-based science instruction and their relations with learning outcomes: Evidence from high and low-performing education systems. International Journal of Science Education, 42(4), 504–525. https://doi.org/10.1080/09500693.2020.1716093
- *Affeldt, F., Meinhart, D., & Eilks, I. (2018). The use of comics in experimental instructions in a non-formal chemistry learning context. International Journal of Education in Mathematics, Science and Technology, 6(1), 93–104. https://doi.org/10.18404/ijemst.380620
- Agustian, H. Y., & Seery, M. K. (2017). Reasserting the role of pre-laboratory activities in chemistry education: A proposed framework for their design. Chemistry Education Research and Practice, 18(4), 518–532. https://doi.org/10.1039/C7RP00140A
- *Apedoe, X. S., Reynolds, B., Ellefson, M. R., & Schunn, C. D. (2008). Bringing engineering design into high school science classrooms: The heating/cooling unit. Journal of Science Education and Technology, 17(5), 454–465. https://doi.org/10.1007/s10956-008-9114-6
- *Ayyildiz, Y., & Tarhan, L. (2018). Problem-based learning in teaching chemistry: Enthalpy changes in systems. Research in Science & Technological Education, 36(1), 35–54. https://doi.org/10.1080/02635143.2017.1366898
- Bain, K., & Towns, M. H. (2016). A review of research on the teaching and learning of chemical kinetics. Chemistry Education Research and Practice, 17(2), 246–262. https://doi.org/10.1039/C5RP00176E
- Banchi, H., & Bell, R. 2008. The many levels of inquiry. Science and Children. 46(2). 26–29 ( EJ815766. ERIC. https://eric.ed.gov/?id=EJ815766
- *Baran, M. (2016). Teaching gases through problem-based learning. Journal of Education and Training Studies, 4(4), 281–294. https://doi.org/10.11114/jets.v4i4.1498
- Barrow, L. H. (2006). A brief history of inquiry: From Dewey to standards. Journal of Science Teacher Education, 17(3), 265–278. https://doi.org/10.1007/s10972-006-9008-5
- *Barthlow, M. J., & Watson, S. B. (2014). The effectiveness of process-oriented guided inquiry learning to reduce alternative conceptions in secondary chemistry. School Science and Mathematics, 114(5), 246–255. https://doi.org/10.1111/ssm.12076
- Bell, R. L., Lederman, N. G., & Abd-El-Khalick, F. (1998). Implicit versus explicit nature of science instruction: An explicit response to Palmquist and Finley. Journal of Research in Science Teaching, 35(9), 1057–1061. https://doi.org/10.1002/(SICI)1098-2736(199811)35:9%3C1057::AID-TEA6%3E3.0.CO;2-C
- Bernard, P., Dudek-Rozycki, K., & Orwat, K. (2019). Integration of inquiry-based instruction with formative assessment: The case of experienced chemistry teachers. Journal of Baltic Science Education, 18(2), 184–196. https://doi.org/10.33225/jbse/19.18.184
- *Blonder, R., Mamlock-Naaman, R., & Hofstein, A. (2008). Analyzing inquiry questions of high-school students in a gas chromatography open-ended laboratory experiment. Chemistry Education Research and Practice, 9(3), 250–258. https://doi.org/10.1039/b812414k
- *Blonder, R., Rap, S., Mamlok-Naaman, R., & Hofstein, A. (2015). Questioning behavior of students in the inquiry chemistry laboratory: Differences between sectors and genders in the Israeli context. International Journal of Science and Mathematics Education, 13, 705–732. https://doi.org/10.1007/s10763-014-9580-7
- Breslyn, W., & McGinnis, J. R. (2012). A comparison of exemplary biology, chemistry, earth science, and physics teachers’ conceptions and enactment of inquiry. Science Education, 96(1), 48–77. https://doi.org/10.1002/sce.20469
- *Bridle, C. A., & Yezierski, E. J. (2012). Evidence for the effectiveness of inquiry-based, particulate-level instruction on conceptions of the particulate nature of matter. Journal of Chemical Education, 89(2), 192–198. https://doi.org/10.1021/ed100735u
- Briggs, D. C. (2008). Comments on Slavin: synthesizing causal inferences. Educational Researcher, 37(1), 15–22. https://doi.org/10.3102/0013189X08314286
- Bybee, R. W., Taylor, J. A., Gardner, A., Van Scotter, P., Powell, J. C., Westbrook, A., & Landes, N. (2006). The BSCS 5E instructional model: Origins and effectiveness. Colorado Springs, Co: BSCS, 5, 88–98. https://fremonths.org/ourpages/auto/2008/5/11/1210522036057/bscs5efullreport2006.pdf
- *Çetin, P. S., & Eymur, G. (2017). Developing students’ scientific writing and presentation skills through argument driven inquiry: An exploratory study. Journal of Chemical Education, 94(7), 837–843. https://doi.org/10.1021/acs.jchemed.6b00915
- *Çetin, P. S., Eymur, G., Southerland, S. A., Walker, J., & Whittington, K. (2018). Exploring the effectiveness of engagement in a broad range of disciplinary practices on learning of Turkish high-school chemistry students. International Journal of Science Education, 40(5), 473–497. https://doi.org/10.1080/09500693.2018.1432914
- *Chairam, S., Klahan, N., & Coll, R. K. (2015). Exploring secondary students’ understanding of chemical kinetics through inquiry-based learning activities. EURASIA Journal of Mathematics, Science & Technology Education, 11(5), 937–956. https://doi.org/10.12973/eurasia.2015.1365a
- *Chan, C. K. K., Lam, I. C. K., & Leung, R. W. H. (2012). Can collaborative knowledge building promote both scientific processes and science achievement?. International Journal of Educational Psychology, 1(3), 199–227. https://doi.org/10.4471/ijep.2012/12
- *Chee, Y. S., & Tan, K. C. D. 2012. Becoming chemists through game-based inquiry learning: The case of “Legends of Alkhimia. Electronic Journal of e-Learning. Vol. 10. 2. 185–198 (EJ985421. ERIC. https://files.eric.ed.gov/fulltext/EJ985421.pdf
- Cheung, A. C., & Slavin, R. E. (2013). The effectiveness of educational technology applications for enhancing mathematics achievement in K-12 classrooms: A meta-analysis. Educational Research Review, 9, 88–113. https://doi.org/10.1016/j.edurev.2013.01.001
- *Cheung, D. (2008). Facilitating chemistry teachers to implement inquiry-based laboratory work. International Journal of Science and Mathematics Education, 6(1), 107–130. https://doi.org/10.1007/s10763-007-9102-y
- *Cheung, D. (2011). Teacher beliefs about implementing guided-inquiry laboratory experiments for secondary school chemistry. Journal of Chemical Education, 88(11), 1462–1468. https://doi.org/10.1021/ed1008409
- Cooper, M. M., & Stowe, R. L. (2018). Chemistry education research: From personal empiricism to evidence, theory, and informed practice. Chemical Reviews, 118(12), 6053–6087. https://doi.org/10.1021/acs.chemrev.8b00020
- Crawford, B. A. (2014). From inquiry to scientific practices in the science classroom. In N. G. Lederman & S. K. Abell (Eds.), Handbook of research on science education (Vol. 2, pp. 515–544). Routledge.
- Crawford, B. A., & Capps, D. K. (2018). Teacher cognition of engaging children in scientific practices. Y. J. Dori, Z. R. Mevarech, & D. R. Baker(Eds.), Cognition, metacognition, and culture in STEM education. (Vol. Vol. 24, pp. 9–32). Springer. https://doi.org/10.1007/978-3-319-66659-4_2
- Creswell, J. W. (2014). A concise introduction to mixed methods research. SAGE.
- *Criswell, B. A., & Rushton, G. T. (2014). Activity structures and the unfolding of problem-solving actions in high-school chemistry classrooms. Research in Science Education, 44(1), 155–188. https://doi.org/10.1007/s11165-013-9374-x
- *Crujeiras-Pérez, B., & Jiménez-Aleixandre, M. (2017). High school students’ engagement in planning investigations: Findings from a longitudinal study in Spain. Chemistry Education Research and Practice, 18(1), 99–112. https://doi.org/10.1039/C6RP00185H
- *Das, P. M., Faikhamta, C., & Punsuvon, V. (2018). Enhancing Bhutanese students’ views of the nature of science in matter and its composition and study of gas laws through an explicit and reflective approach. Science Education International, 29(1), 20–28. https://doi.org/10.33828/sei.v29.i1.3
- *Davenport, J. L., Rafferty, A. N., & Yaron, D. J. (2018). Whether and how authentic contexts using a virtual chemistry lab support learning. Journal of Chemical Education, 95(8), 1250–1259. https://doi.org/10.1021/acs.jchemed.8b00048
- *De Gale, S., & Boisselle, L. N. 2015. The effect of POGIL on academic performance and academic confidence. Science Education International. Vol. 26. 1. 56–79 (EJ1056455. ERIC. https://eric.ed.gov/?id=EJ1056455
- *Deng, F., Chen, W. L., Chai, C. S., & Qian, Y. Y. (2011). Constructivist-oriented data-logging activities in Chinese chemistry classroom: Enhancing students’ conceptual understanding and their metacognition. Asia-Pacific Education Researcher, 20(2), 207–221. https://www.ejournals.ph/uploads/archive/TAPER/Vol.%2020%20No.%202%20(2011)/SSPAL/2_FENG%20DENG.pdf
- Dewey, J. (1910). Science as subject-matter and as method. Science, 31(787), 121–127. https://www.jstor.org/stable/1634781
- *Dkeidek, I., Mamlok-Naaman, R., & Hofstein, A. (2011). Effect of culture on high-school students’ question-asking ability resulting from an inquiry-oriented chemistry laboratory. International Journal of Science and Mathematics Education, 9(6), 1305–1331. https://doi.org/10.1007/s10763-010-9261-0
- *Dkeidek, I., Mamlok-Naaman, R., & Hofstein, A. (2012). Assessment of the laboratory learning environment in an inquiry-oriented chemistry laboratory in Arab and Jewish high schools in Israel. Learning Environments Research, 15(2), 141–169. https://doi.org/10.1007/s10984-012-9109-3
- Dobber, M., Zwart, R., Tanis, M., & van Oers, B. (2017). Literature review: The role of the teacher in inquiry-based education. Educational Research Review, 22, 194–214. https://doi.org/10.1016/j.edurev.2017.09.002
- Domin, D. S. (1999). A review of laboratory instruction styles. Journal of Chemical Education, 76(4), 543. https://doi.org/10.1021/ed076p543
- *Donnelly, D., O’Reilly, J., & McGarr, O. (2013). Enhancing the student experiment experience: Visible scientific inquiry through a virtual chemistry laboratory. Research in Science Education, 43(4), 1571–1592. https://doi.org/10.1007/s11165-012-9322-1
- *Donnelly, D. F., McGarr, O., & O’Reilly, J. (2014). “Just be quiet and listen to exactly what he’s saying”: Conceptualising power relations in inquiry-oriented classrooms. International Journal of Science Education, 36(12), 2029–2054. https://doi.org/10.1080/09500693.2014.889867
- *Dori, Y. J., & Sasson, I. (2008). Chemical understanding and graphing skills in an honors case-based computerized chemistry laboratory environment: The value of bidirectional visual and textual representations. Journal of Research in Science Teaching, 45(2), 219–250. https://doi.org/10.1002/tea.20197
- Dorman, J. P. (2012). The Impact of Student Clustering on the Results of Statistical Tests. B. Fraser, K. Tobin, & C. McRobbie. Eds., Second International Handbook of Science Education. 1333–1348: Springer International Handbooks of Education. Vol. 24. Springer. https://doi.org/10.1007/978-1-4020-9041-7_86.
- *Doucerain, M., & Schwartz, M. S. (2010). Analyzing learning about conservation of matter in students while adapting to the needs of a school. Mind, Brain, and Education, 4(3), 112–124. https://doi.org/10.1111/j.1751-228X.2010.01090.x
- Drake, K. N., & Long, D. (2009). Rebecca’s in the dark: A comparative study of problem-based learning and direct instruction/experiential learning in two 4th-grade classrooms. Journal of Elementary Science Education, 21(1), 1–16. https://doi.org/10.1007/BF03174712
- *Duffin, L. C., Starling, M. P., Day, M. M., & Cribbs, J. D. (2016). The effects of a consumer chemistry intervention on urban at-risk high school students’ performance, utility value, and intentions to pursue STEM. School Science and Mathematics, 116(7), 356–365. https://doi.org/10.1111/ssm.12188
- Duschl, R. (2008). Science education in three-part harmony: Balancing conceptual, epistemic, and social learning goals. Review of Research in Education, 32(1), 268–291. https://doi.org/10.3102/0091732X07309371
- Duschl, R. A. (2003). Assessment of inquiry. In J. M. Atkin & J. Coffey (Eds.), Everyday assessment in the science classroom (pp. 41–59). NSTA Press.
- Eberlein, T., Kampmeier, J., Minderhout, V., Moog, R. S., Platt, T., Varma‐Nelson, P., & White, H. B. (2008). Pedagogies of engagement in science: A comparison of PBL, POGIL, and PLTL. Biochemistry and Molecular Biology Education, 36(4), 262–273. https://doi.org/10.1002/bmb.20204
- Egger, M., Juni, P., Bartlett, C., Holenstein, F., & Sterne, J. (2003). How important are comprehensive literature searches and the assessment of trial quality in systematic reviews? Empirical study. Health Technology Assessment, 7(1), 1–76. https://doi.org/10.3310/hta7010
- *Eymur, G. (2018). Developing high school students’ self-efficacy and perceptions about inquiry and laboratory skills through argument-driven inquiry. Journal of Chemical Education, 95(5), 709–715. https://doi.org/10.1021/acs.jchemed.7b00934
- *Eymur, G. (2019). The influence of the explicit nature of science instruction embedded in the argument-driven inquiry method in chemistry laboratories on high school students’ conceptions about the nature of science. Chemistry Education Research and Practice, 20(1), 17–29. https://doi.org/10.1039/c8rp00135a
- *Ferreira, M. M., & Trudel, A. R. (2012). The impact of problem-based learning (PBL) on student attitudes toward science, problem-solving skills, and sense of community in the classroom. Journal of Classroom Interaction, 47(1), 23–30. https://www.jstor.org/stable/43858871
- Furtak, E. M., Seidel, T., Iverson, H., & Briggs, D. C. (2012). Experimental and quasi-experimental studies of inquiry-based science teaching: A meta-analysis. Review of Educational Research, 82(3), 300–329. https://doi.org/10.3102/0034654312457206
- *Gao, S., & Wang, J. (2014). Teaching transformation under centralized curriculum and teacher learning community: Two Chinese chemistry teachers’ experiences in developing inquiry-based instruction. Teaching and Teacher Education, 44, 1–11. https://doi.org/10.1016/j.tate.2014.07.008
- Gericke, N., Högström, P., & Wallin, J. (2022). A systematic review of research on laboratory work in secondary school. Studies in Science Education, 1–41. https://doi.org/10.1080/03057267.2022.2090125
- Grant, M. J., & Booth, A. (2009). A typology of reviews: An analysis of 14 review types and associated methodologies. Health Information & Libraries Journal, 26(2), 91–108. https://doi.org/10.1111/j.1471-1842.2009.00848.x
- Graulich, N. (2015). The tip of the iceberg in organic chemistry classes: How do students deal with the invisible?. Chemistry Education Research and Practice, 16(1), 9–21. https://doi.org/10.1039/C4RP00165F
- Gyllenpalm, J., Wickman, P.-O., & Holmgren, S.-O. (2010). Secondary science teachers’ selective traditions and examples of inquiry-oriented approaches. Nordic Studies in Science Education, 6(1), 44–60. https://doi.org/10.5617/nordina.269
- Hanson, D. M. (2006). Instructor’s guide to process-oriented guided-inquiry learning. Pacific Crest Lisle.
- *Harshman, J., & Yezierski, E. (2015). Guiding teaching with assessments: High school chemistry teachers’ use of data-driven inquiry. Chemistry Education Research and Practice, 16(1), 93–103. https://doi.org/10.1039/C4RP00188E
- Herranen, J., & Aksela, M. (2019). Student-question-based inquiry in science education. Studies in Science Education, 55(1), 1–36. https://doi.org/10.1080/03057267.2019.1658059
- *Herrington, D. G., Yezierski, E. J., Luxford, K. M., & Luxford, C. J. (2011). Target inquiry: Changing chemistry high school teachers’ classroom practices and knowledge and beliefs about inquiry instruction. Chemistry Education Research and Practice, 12(1), 74–84. https://doi.org/10.1039/C1RP90010B
- Hmelo-Silver, C. E., Duncan, R. G., & Chinn, C. A. (2007). Scaffolding and achievement in problem-based and inquiry learning: A response to Kirschner, Sweller, and Clark (2006). Educational Psychologist, 42(2), 99–107. https://doi.org/10.1080/00461520701263368
- *Hofstein, A., Navon, O., Kipnis, M., & Mamlok-Naaman, R. (2005). Developing students’ ability to ask more and better questions resulting from inquiry-type chemistry laboratories. Journal of Research in Science Teaching, 42(7), 791–806. https://doi.org/10.1002/tea.20072
- Högstrom, P., Ottander, C., & Benckert, S. (2010). Lab work and learning in secondary school chemistry: The importance of teacher and student interaction. Research in Science Education, 40(4), 505–523. https://doi.org/10.1007/s11165-009-9131-3
- *Hug, B., & McNeill, K. L. (2008). Use of first-hand and second-hand data in science: Does data type influence classroom conversations?. International Journal of Science Education, 30(13), 1725–1751. https://doi.org/10.1080/09500690701506945
- *Itzek-Greulich, H., & Vollmer, C. (2017). Emotional and motivational outcomes of lab work in the secondary intermediate track: The contribution of a science center outreach lab. Journal of Research in Science Teaching, 54(1), 3–28. https://doi.org/10.1002/tea.21334
- Johnstone, A. H. (1991). Why is science difficult to learn? Things are seldom what they seem. Journal of Computer Assisted Learning, 7(2), 75–83. https://doi.org/10.1111/j.1365-2729.1991.tb00230.x
- *Juntunen, M., & Aksela, M. (2013a). Life-cycle thinking in inquiry-based sustainability education: Effects on students’ attitudes towards chemistry and environmental literacy. Center for Educational Policy Studies Journal, 3(2), 157–180. https://doi.org/10.26529/cepsj.244
- *Juntunen, M., & Aksela, M. 2013b. Life-cycle analysis and inquiry-based learning in chemistry teaching. Science Education International. Vol. 24. 2. 150–166 (EJ1015764. ERIC. https://files.eric.ed.gov/fulltext/EJ1015764.pdf
- *Juntunen, M., & Aksela, M. (2014). Improving students’ argumentation skills through a product life-cycle analysis project in chemistry education. Chemistry Education Research and Practice, 15(4), 639–649. https://doi.org/10.1039/C4RP00068D
- *Kaberman, Z., & Dori, Y. J. (2009a). Metacognition in chemical education: Question posing in the case-based computerized learning environment. Instructional Science: An International Journal of the Learning Sciences, 37(5), 403–436. https://doi.org/10.1007/s11251-008-9054-9
- *Kaberman, Z., & Dori, Y. J. (2009b). Question posing, inquiry, and modeling skills of chemistry students in the case-based computerized laboratory environment. International Journal of Science and Mathematics Education, 7(3), 597–625. https://doi.org/10.1007/s10763-007-9118-3
- *Katchevich, D., Hofstein, A., & Mamlok-Naaman, R. (2013). Argumentation in the chemistry laboratory: Inquiry and confirmatory experiments. Research in Science Education, 43(1), 317–345. https://doi.org/10.1007/s11165-011-9267-9
- Keys, C. W., Hand, B., Prain, V., & Collins, S. (1999). Using the science writing heuristic as a tool for learning from laboratory investigations in secondary science. Journal of Research in Science Teaching, 36(10), 1065–1084. https://doi.org/10.1002/(SICI)1098-2736(199912)36:10%3C1065::AID-TEA2%3E3.0.CO;2-I
- *Kimberlin, S., & Yezierski, E. (2016). Effectiveness of inquiry-based lessons using particulate level models to develop high school students’ understanding of conceptual stoichiometry. Journal of Chemical Education, 93(6), 1002–1009. https://doi.org/10.1021/acs.jchemed.5b01010
- King, D. (2012). New perspectives on context-based chemistry education: Using a dialectical sociocultural approach to view teaching and learning. Studies in Science Education, 48(1), 51–87. https://doi.org/10.1080/03057267.2012.655037
- *Kingir, S., Geban, O., & Gunel, M. (2012). How does the science writing heuristic approach affect students’ performances of different academic achievement levels? A case for high school chemistry. Chemistry Education Research and Practice, 13(4), 428–436. https://doi.org/10.1039/C2RP20013A
- *Kingir, S., Geban, O., & Gunel, M. (2013). Using the science writing heuristic approach to enhance student understanding in chemical change and mixture. Research in Science Education, 43(4), 1645–1663. https://doi.org/10.1007/s11165-012-9326-x
- *Kipnis, M., & Hofstein, A. (2008). The inquiry laboratory as a source for development of metacognitive skills. International Journal of Science and Mathematics Education, 6(3), 601–627. https://doi.org/10.1007/s10763-007-9066-y
- Kirschner, P., Sweller, J., & Clark, R. E. (2006). Why unguided learning does not work: An analysis of the failure of discovery learning, problem-based learning, experiential learning and inquiry-based learning. Educational Psychologist, 41(2), 75–86. https://doi.org/10.1207/s15326985ep4102_1
- *Kumdang, P., Kijkuakul, S., & Chaiyasith, W. C. (2018). An action research on enhancing grade 10 student creative thinking skills using argument-driven inquiry model in the topic of chemical environment. Journal of Science Learning, 2(1), 9–13. https://doi.org/10.17509/jsl.v2i1.11995
- Lederman, N. G., & Lederman, J. S. (2012). Nature of scientific knowledge and scientific inquiry: Building instructional capacity through professional development. In B. J. Fraser, K. Tobin, & C. J. McRobbie (Eds.), Second international handbook of science education (pp. 335–359). Springer. https://doi.org/10.1007/978-1-4020-9041-7_24
- *Li, W. S. S., & Arshad, M. Y. (2015). Inquiry practices in Malaysian secondary classroom and model of inquiry teaching based on verbal interaction. Malaysian Journal of Learning and Instruction, 12, 151–175. https://doi.org/10.32890/mjli2015.12.8
- *Lu, S., Bi, H., & Liu, X. (2018). The effects of explanation-driven inquiry on students’ conceptual understanding of redox. International Journal of Science Education, 40(15), 1857–1873. https://doi.org/10.1080/09500693.2018.1513670
- *McNeill, K. L. (2009). Teachers’ use of curriculum to support students in writing scientific arguments to explain phenomena. Science Education, 93(2), 233–268. https://doi.org/10.1002/sce.20294
- *McNeill, K. L., & Krajcik, J. (2008). Scientific explanations: Characterizing and evaluating the effects of teachers’ instructional practices on student learning. Journal of Research in Science Teaching, 45(1), 53–78. https://doi.org/10.1002/tea.20201
- Minner, D. D., Levy, A. J., & Century, J. (2010). Inquiry‐based science instruction: What is it and does it matter? Results from a research synthesis years 1984 to 2002. Journal of Research in Science Teaching: The Official Journal of the National Association for Research in Science Teaching, 47(4), 474–496. https://doi.org/10.1002/tea.20347
- National Research Council. (2012). A framework for K-12 science education: Practices, crosscutting concepts, and core ideas. T. N. A. Press. https://www.nap.edu/read/13165/chapter/1
- *Neber, H., & Anton, M. (2008). Promoting pre-experimental activities in high-school chemistry: Focusing on the role of students’ epistemic questions. International Journal of Science Education, 30(13), 1801–1821. https://doi.org/10.1080/09500690701579546
- *Nehring, A., Nowak, K. H., Zu Belzen, A. U., & Tiemann, R. (2015). Predicting students’ skills in the context of scientific inquiry with cognitive, motivational, and sociodemographic variables. International Journal of Science Education, 37(9), 1343–1363. https://doi.org/10.1080/09500693.2015.1035358
- Osborne, J. (2010). Science for citizenship. In J. Osborne & J. Dillon (Eds.), Good practice in science teaching: What research has to say (pp. 46–67). McGraw-Hill Education.
- Osborne, J. (2014a). Scientific practices and inquiry in the science classroom. In N. G. Lederman & S. K. Abell (Eds.), Handbook of research on science education (Vol. II, pp. 593–613). Routledge.
- Osborne, J. (2014b). Teaching scientific practices: Meeting the challenge of change. Journal of Science Teacher Education, 25(2), 177–196. https://doi.org/10.1007/s10972-014-9384-1
- Osborne, J., Ratcliffe, M., Collins, S., Millar, R., & Duschl, R. (2001). What should we teach about science: A Delphi study. Evidence-based Practice in Science Education (EPSE) Report. https://www.researchgate.net/publication/249748934_What_should_we_teach_about_science_A_Delphi_study
- *Osokoya, M. M., & Nwazota, C. C. 2018. Problem-based and school-type as contributory factors to the senior secondary school students’ practical skills in chemistry. Journal of the International Society for Teacher Education. Vol. 22. 1. 7–18 (EJ1237537. ERIC. https://eric.ed.gov/?id=EJ1237537
- Overton, T. L., Byers, B., & Seery, M. K. (2009). Context-and problem-based learning in higher level chemistry education. In I. Eilks & B. Byers (Eds.), Innovative methods of teaching and learning chemistry in higher education (pp. 43–59). RSC Publishing.
- *Özgür, S. D., & Yilmaz, A. (2017). The effect of inquiry-based learning on gifted and talented students’ understanding of acids-bases concepts and motivation. Journal of Baltic Science Education, 16(6), 994–1008. https://www.researchgate.net/publication/251122647_Improving_Practice_with_Target_Inquiry_High_School_Chemistry_Teacher_Professional_Development_That_Works
- *Özkanbaş, M., & Kırık, Ö. T. (2020). Implementing collaborative inquiry in a middle school science course. Chemistry Education Research and Practice, 21(4), 1199–1217. https://doi.org/10.1039/C9RP00231F
- *Patchen, T., & Smithenry, D. W. (2014). Diversifying instruction and shifting authority: A cultural historical activity theory (CHAT) analysis of classroom participant structures. Journal of Research in Science Teaching, 51(5), 606–634. https://doi.org/10.1002/tea.21140
- Pedaste, M., Mäeots, M., Siiman, L. A., De Jong, T., Van Riesen, S. A., Kamp, E. T., Manoli, C. C., Zacharia, Z. C., & Tsourlidaki, E. (2015). Phases of inquiry-based learning: Definitions and the inquiry cycle. Educational Research Review, 14, 47–61. https://doi.org/10.1016/j.edurev.2015.02.003
- *Peleg, R., Yayon, M., Katchevich, D., Mamlok-Naaman, R., Fortus, D., Eilks, I., & Hofstein, A. (2017). Teachers’ views on implementing storytelling as a way to motivate inquiry learning in high-school chemistry teaching. Chemistry Education Research and Practice, 18(2), 304–309. https://doi.org/10.1039/C6RP00215C
- *Pyatt, K., & Sims, R. (2012). Virtual and physical experimentation in inquiry-based science labs: Attitudes, performance and access. Journal of Science Education and Technology, 21(1), 133–147. https://doi.org/10.1007/s10956-011-9291-6
- *Rahayu, S., Chandrasegaran, A. L., Treagust, D. F., Kita, M., & Ibnu, S. (2011). Understanding acid–base concepts: Evaluating the efficacy of a senior high school student-centred instructional program in Indonesia. International Journal of Science and Mathematics Education, 9, 1439–1458. https://doi.org/10.1007/s10763-010-9272-x
- Rodriguez, J.-M. G., Hunter, K. H., Scharlott, L. J., & Becker, N. M. (2020). A review of research on process oriented guided inquiry learning: Implications for research and practice. Journal of Chemical Education, 97(10), 3506–3520. https://doi.org/10.1021/acs.jchemed.0c00355
- *Roehrig, G. H., & Luft, J. A. (2004). Inquiry teaching in high school chemistry classrooms: The role of knowledge and beliefs. Journal of Chemical Education, 81(10), 1510. https://doi.org/10.1021/ed081p1510
- Rönnebeck, S., Bernholt, S., & Ropohl, M. (2016). Searching for a common ground: A literature review of empirical research on scientific inquiry activities. Studies in Science Education, 52(2), 161–197. https://doi.org/10.1080/03057267.2016.1206351
- *Ryoo, K., Bedell, K., & Swearingen, A. (2018). Promoting linguistically diverse students’ short-term and long-term understanding of chemical phenomena using visualizations. Journal of Science Education and Technology, 27(6), 508–522. https://doi.org/10.1007/s10956-018-9739-z
- *Sampson, V., & Clark, D. (2009). The impact of collaboration on the outcomes of scientific argumentation. Science Education, 93(3), 448–484. https://doi.org/10.1002/sce.20306
- Sampson, V., Enderle, P., Grooms, J., & Witte, S. (2013). Writing to learn by learning to write during the school science laboratory: Helping middle and high school students develop argumentative writing skills as they learn core ideas. Science Education, 97(5), 643–670. https://doi.org/10.1002/sce.21069
- Sampson, V., Grooms, J., & Walker, J. 2009. Argument-driven inquiry. Science Teacher. Vol. 76. 8. 42–47 (EJ862799. ERIC. https://eric.ed.gov/?id=EJ862799
- *Sampson, V., Grooms, J., & Walker, J. P. (2011). Argument‐driven inquiry as a way to help students learn how to participate in scientific argumentation and craft written arguments: An exploratory study. Science Education, 95(2), 217–257. https://doi.org/10.1002/sce.20421
- Sarıtaş, D., Özcan, H., & Adúriz-Bravo, A. (2021). Observation and inference in chemistry teaching: a model-based approach to the integration of the macro and submicro levels. Science & Education, 30(5), 1289–1314. https://doi.org/10.1007/s11191-021-00216-z
- Savelsbergh, E. R., Prins, G. T., Rietbergen, C., Fechner, S., Vaessen, B. E., Draijer, J. M., & Bakker, A. (2016). Effects of innovative science and mathematics teaching on student attitudes and achievement: A meta-analytic study. Educational Research Review, 19, 158–172. https://doi.org/10.1016/j.edurev.2016.07.003
- Schwab, J. J. (1960). Inquiry, the science teacher, and the educator. The School Review, 68(2), 176–195. https://doi.org/10.1086/442536
- *Sen, S., Yilmaz, A., & Geban, Ö. (2015). The effects of process oriented guided inquiry learning environment on students’ selfregulated learning skills. Problems of Education in the 21st Century, 66, 54–66. https://oaji.net/articles/2015/457-1441442208.pdf
- *Sen, S., Yilmaz, A., & Geban, Ö. 2016. The effect of process oriented guided inquiry learning (POGIL) on 11th graders’ conceptual understanding of electrochemistry. Asia-Pacific Forum on Science Learning and Teaching. Vol. 17. 2. EJ1145679. ERIC. https://eric.ed.gov/?id=EJ1145679
- *Sesen, B. A., & Tarhan, L. (2013). Inquiry-based laboratory activities in electrochemistry: High school students’ achievements and attitudes. Research in Science Education, 43(1), 413–435. https://doi.org/10.1007/s11165-011-9275-9
- *Smith, C. J. (2012). Improving the school-to-university transition: Using a problem-based approach to teach practical skills whilst simultaneously developing students’ independent study skills. Chemistry Education Research and Practice, 13(4), 490–499. https://doi.org/10.1039/C2RP20096A
- *Smithenry, D. W. (2010). Integrating guided inquiry into a traditional chemistry curricular framework. International Journal of Science Education, 32(13), 1689–1714. https://doi.org/10.1080/09500690903150617
- *Sola, A. O., & Ojo, O. E. 2007. Effects of project, inquiry and lecture-demonstration teaching methods on senior secondary students’ achievement in separation of mixtures practical test. Educational Research and Reviews. Vol. 2. 6. 124–132 (EJ900156. ERIC. https://eric.ed.gov/?id=EJ900156
- *Sotakova, I., Ganajova, M., & Babincakova, M. (2020). Inquiry-based science education as a revision strategy. Journal of Baltic Science Education, 19(3), 499–513. https://doi.org/10.33225/jbse/20.19.499
- Sozbilir, M., Akilli, M., Yasar, M. D., & Dede, H. (2016). Development of chemistry education research (CER) in Turkey: A comparison of CER papers with international research. Science Education Research and Practice in Asia, 289. https://doi.org/10.1007/978-981-10-0847-4_16
- *Srisawasdi, N., & Panjaburee, P. (2019). Implementation of game-transformed inquiry-based learning to promote the understanding of and motivation to learn chemistry. Journal of Science Education and Technology, 28(2), 152–164. https://doi.org/10.1007/s10956-018-9754-0
- *Stieff, M. (2011). Improving representational competence using molecular simulations embedded in inquiry activities. Journal of Research in Science Teaching, 48(10), 1137–1158. https://doi.org/10.1002/tea.20438
- *Stieff, M. (2019). Improving learning outcomes in secondary chemistry with visualization-supported inquiry activities. Journal of Chemical Education, 96(7), 1300–1307. https://doi.org/10.1021/acs.jchemed.9b00205
- Strat, T. T. S., Henriksen, E. K., & Jegstad, K. M. (2023). Inquiry-based science education in science teacher education: a systematic review. Studies in Science Education, 1–59. https://doi.org/10.1080/03057267.2023.2207148
- *Strippel, C. G., & Sommer, K. (2015). Teaching nature of scientific inquiry in chemistry: How do German chemistry teachers use labwork to teach NOSI?. International Journal of Science Education, 37(18), 2965–2986. https://doi.org/10.1080/09500693.2015.1119330
- *Sudria, I. B. N., Redhana, I. W., Kirna, I. M., & Aini, D. (2018). Effect of Kolb’s learning styles under inductive guided-inquiry learning on learning outcomes. International Journal of Instruction, 11(1), 89–102. https://doi.org/10.12973/iji.2018.1117a
- *Sugiharti, G., & Habeahan, B. J. W. (2018). Influence of learning model using laboratory and numeric ability to student learning result on thermochemical material. International Education Studies, 11(5), 154–160. https://doi.org/10.5539/ies.v11n5p154
- *Supasorn, S. (2015). Grade 12 students’ conceptual understanding and mental models of galvanic cells before and after learning by using small-scale experiments in conjunction with a model kit. Chemistry Education Research and Practice, 16(2), 393–407. https://doi.org/10.1039/C4RP00247D
- *Supasorn, S., & Promarak, V. (2015). Implementation of 5E inquiry incorporated with analogy learning approach to enhance conceptual understanding of chemical reaction rate for grade 11 students. Chemistry Education Research and Practice, 16(1), 121–132. https://doi.org/10.1039/C4RP00190G
- Sutton, A., Clowes, M., Preston, L., & Booth, A. (2019). Meeting the review family: Exploring review types and associated information retrieval requirements. Health Information & Libraries Journal, 36(3), 202–222. https://doi.org/10.1111/hir.12276
- *Szalay, L., & Tóth, Z. (2016). An inquiry-based approach of traditional “step-by-step” experiments. Chemistry Education Research and Practice, 17(4), 923–961. https://doi.org/10.1039/C6RP00044D
- *Szalay, L., Tóth, Z., & Kiss, E. (2020). Introducing students to experimental design skills. Chemistry Education Research and Practice, 21(1), 331–356. https://doi.org/10.1039/C9RP00234K
- Taber, K. S. (2019). Experimental research into teaching innovations: responding to methodological and ethical challenges. Studies in Science Education, 55(1), 69–119. https://doi.org/10.1080/03057267.2019.1658058
- Talanquer, V. (2011). Macro, submicro, and symbolic: The many faces of the chemistry “triplet”. International Journal of Science Education, 33(2), 179–195. https://doi.org/10.1080/09500690903386435
- *Tarhan, L., & Acar, B. (2007). Problem‐based learning in an eleventh grade chemistry class: “Factors affecting cell potential. Research in Science & Technological Education, 25(3), 351–369. https://doi.org/10.1080/02635140701535299
- *Tarhan, L., & Acar-Sesen, B. (2013). Problem based learning in acids and bases: Learning achievements and students’ beliefs. Journal of Baltic Science Education, 12(5), 565–578. http://dx.doi.org/10.33225/jbse/13.12.565
- *Tarhan, L., Ayar-Kayali, H., Urek, R. O., & Acar, B. (2008). Problem-based learning in 9th grade chemistry class: “Intermolecular forces. Research in Science Education, 38(3), 285–300. https://doi.org/10.1007/s11165-007-9050-0
- Teig, N., Scherer, R., & Nilsen, T. (2018). More isn’t always better: The curvilinear relationship between inquiry-based teaching and student achievement in science. Learning and Instruction, 56, 20–29. https://doi.org/10.1016/j.learninstruc.2018.02.006
- Teo, T. W., Goh, M. T., & Yeo, L. W. (2014). Chemistry education research trends: 2004–2013. Chemistry Education Research and Practice, 15(4), 470–487. https://doi.org/10.1039/C4RP00104D
- *Tolentino, L., Birchfield, D., Megowan-Romanowicz, C., Johnson-Glenberg, M. C., Kelliher, A., & Martinez, C. (2009). Teaching and learning in the mixed-reality science classroom. Journal of Science Education and Technology, 18(6), 501–517. https://doi.org/10.1007/s10956-009-9166-210.1007/s10956-009-9166-2
- *Tornee, N., Bunterm, T., Lee, K., & Muchimapura, S. (2019). Examining the effectiveness of guided inquiry with problem-solving process and cognitive function training in a high school chemistry course. Pedagogies: An International Journal, 14(2), 126–149. https://doi.org/10.1080/1554480X.2019.1597722
- *Treagust, D. F., Qureshi, S. S., Vishnumolakala, V. R., Ojeil, J., Mocerino, M., & Southam, D. C. (2020). Process-oriented guided inquiry learning (POGIL) as a culturally relevant pedagogy (CRP) in Qatar: A perspective from grade 10 chemistry classes. Research in Science Education, 50(3), 813–831. https://doi.org/10.1007/s11165-018-9712-0
- *Valdez, J. E., & Bungihan, M. E. (2019). Problem-based learning approach enhances the problem solving skills in chemistry of high school students. Journal of Technology and Science Education, 9(3), 282–294. https://raco.cat/index.php/JOTSE/article/view/364176
- *van Brederode, M. E., Zoon, S. A., & Meeter, M. (2020). Examining the effect of lab instructions on students’ critical thinking during a chemical inquiry practical. Chemistry Education Research and Practice, 21(4), 1173–1182. https://doi.org/10.1039/D0RP00020E
- *van Rens, L., Hermarij, P., Pilot, A., Beishuizen, J., Hofman, H., & Wal, M. (2014). Pre-university chemistry students in a mimicked scholarly peer review. International Journal of Science Education, 36(15), 2514–2533. https://doi.org/10.1080/09500693.2014.895447
- *van Rens, L., Pilot, A., & van der Schee, J. (2010). A framework for teaching scientific inquiry in upper secondary school chemistry. Journal of Research in Science Teaching, 47(7), 788–806. https://doi.org/10.1002/tea.20357
- *van Rens, L., Pilot, A., & van Dijk, H. (2004). Enhancement of quality in chemical inquiry by pre-university students. International Journal of Science and Mathematics Education, 2(4), 493–509. https://doi.org/10.1007/s10763-004-2893-1
- *van Rens, L., van Muijlwijk, J., Beishuizen, J., & van der Schee, J. (2013). Upper secondary chemistry students in a pharmacochemistry research community. International Journal of Science Education, 35(6), 1012–1036. https://doi.org/10.1080/09500693.2011.591845
- *Vhurumuku, E. (2011). High school chemistry students’ scientific epistemologies and perceptions of the nature of laboratory inquiry. Chemistry Education Research and Practice, 12(1), 47–56. https://doi.org/10.1039/C1RP90007B
- *Wang, L., Zhang, R., Clarke, D., & Wang, W. (2014). Enactment of scientific inquiry: Observation of two cases at different grade levels in China Mainland. Journal of Science Education and Technology, 23(2), 280–297. https://doi.org/10.1007/s10956-013-9486-0
- *Yezierski, E. J., & Herrington, D. G. (2011). Improving practice with Target Inquiry: High school chemistry teacher professional development that works. Chemistry Education Research and Practice, 12(3), 344–354. https://doi.org/10.1039/C1RP90041B
- *Zhang, Z. H., & Linn, M. C. (2011). Can generating representations enhance learning with dynamic visualizations?. Journal of Research in Science Teaching, 48(10), 1177–1198. https://doi.org/10.1002/tea.20443