Abstract
A reverse transcription-polymerase chain reaction is described, which amplified the full-length σC-encoding and σNS-encoding genes of avian reovirus (ARV). DNA fragments of 1022 and 1152 base pairs were amplified among ARV isolates, respectively, indicating that there were no apparent deletions or insertions in these regions. Fragments amplified from vaccine strains and field isolates were digested with five different restriction enzymes Bcn I, Hae III, Taq I, Dde I, and Hinc II, respectively. Restriction fragment profiles observed on polyacrylamide gels showed heterogeneity between vaccine and Taiwanese isolates. All ARV isolates tested showed different restriction enzyme cleavage patterns and could be clearly distinguished. The strain-typing based on the cleavage sites in the σC-encoding gene of ARV showed that viruses could be classified into four distinct groups. A phylogenetic tree based on the nucleotide sequences of the σC-encoding gene revealed that Taiwanese ARV isolates were classified into four distinct groups, indicating that the genotyping is consistent with typing based on restriction enzyme fragment length polymorphism of the σC-encoding gene of ARV. The results suggested that polymerase chain reaction followed by restriction enzyme analysis provided a simple and rapid approach for characterization of ARV isolates. Also, it is possible to determine whether a new variant strain has been introduced into a flock or a given virus strain has spread from one flock to another.
Résumé
Caractérisation rapide des réovirus aviaires en utilisant l'analyse phylogénétique, l'amplification en chaîne par polymérase et le polymorphisme de taille des fragments de restrictionUne méthode de transcription reverse et d'amplification en chaîne par polymérase (RT-PCR) qui amplifie toute la longueur des gènes codant σC et σNS des réovirus aviaires (ARV) est décrite. Les fragments d'ADN de 1022 et 1152 paires de bases ont été amplifiés parmi les souches ARV isolées indiquant qu'il n'y avait pas de délétions apparentes ou d'insertions au niveau de ces régions. Les fragments amplifiés à partir des souches vaccinales et celles isolées du terrain ont fait l'objet de digestion avec cinq différentes enzymes de restriction Bcn I, Hae III, Taq I, Dde I, et Hinc II. Les profils des fragments de restriction observés sur gel de polyacrylamide ont montré une hétérogénéité entre les souches terrain taiwanaises et les vaccins. Tous les ARV, isolés du terrain, testés, ont présenté des profils de restriction enzymatique différents et peuvent être distingués clairement. Le typage des souches, basé sur les sites de clivage du gène codant σC des ARV, a montré que les virus pouvaient être classés en 4 groupes. L'arbre phylogénétique, basé sur la séquence nucléotidique du gène codant σC, a montré que les souches terrain taiwanaises d'ARV étaient classées en 4 groupes, indiquant que le génotypage était en accord avec le typage basé sur le polymorphisme de taille des fragments de restriction (RFLP) du gène codant σC des ARV. Ces résultats suggèrent que la PCR suivie d'une analyse de restriction enzymatique apporte une approche simple et rapide de la caractérisation des souches terrain d'ARV. Ainsi, il est possible de déterminer si une nouvelle souche variante a été introduite dans un troupeau ou si une souche de virus a diffusé d'un troupeau à un autre.
Zusammenfassung
Schnelle Charkterisierung von aviären Reoviren mittels phylogenetischer Analyse, Reverse Transkriptase-Polymerasekettenreaktion und RestriktionsenzymfragmentlängenpolymorphismusEine Reverse Transkriptase-Polymerasekettenreaktion (RT-PCR), die die Gesamtlänge der σC-und σNS-kodierenden Gene des aviären Reovirus (ARV) amplifiziert, wird beschrieben. Bei verschiedenen ARV-Isolaten wurden DNS-Fragmente von 1022 bzw. 1152 Basenpaaren wurden amplifiziert. Es zeigten sich keine offensichtlichen Deletionen oder Insertionen in diesen Regionen. Aus Vakzine- und Feldstämmen amplifizierte Fragmente wurden mit fünf verschiedenen Restriktionsenzymen (Bcn I, Hae III, Taq I, Dde I und Hinc II) verdaut. Die auf Polyacrylamidgelen zu beobachtenden Restriktionsfragmentprofile ließen eine Heterogenität zwischen den Vakzinestämmen und den taiwanesischen Isolaten erkennen. Alle untersuchten ARV-Isolate wiesen verschiedene Restriktionsenzymspaltungsmuster auf und konnten deutlich unterschieden werden. Die auf den Spaltungsstellen im σC-kodierenden ARV-Gen basierende Stammtypisierung zeigte, dass die Viren in vier unterschiedliche Gruppen klassifiziert werden konnten. Ein auf der Basis der Nukleotidsequenzen des σC-kodierenden Gens erstellter phylogenetischer Baum ließ erkennen, dass die taiwanesischen ARV-Isolate ebenfalls in vier verschiedene Gruppen eingeordnet wurden, was darauf hin weist, dass die Genotypisierung mit der Typisierung übereinstimmt, die auf dem Restriktionsenzymfragmentlängenpolymorphismus (RFLP) des im σC-kodierenden ARV-Gens basiert. Die Ergebnise legen nahe, dass die PCR mit nachfolgender Restriktionsenzymanalyse eine einfache und schnelle Methode zur Charakterisierung von ARV-Isolaten darstellt. Ebenso ist es mögich festzustellen, ob eine neuer Variantstamm in einer Herde aufgetreten ist oder ein bekannter Virusstamm sich von einer auf eine andere Herde ausgebreitet hat.Caracterización rápida de los reovirus aviares mediante análisis filogenético, transcripción reversa- reacción en cadena de la polimerasa, y polimorfismo de la longitud de los fragmentos de restricción enzimática
Resumen
Caracterización rápida de los reovirus aviares mediante análisis filogenético, transcripción reversa- reacción en cadena de la polimerasa, y polimorfismo de la longitud de los fragmentos de restricción enzimáticaSe describe una técnica de transcripción reversa- reacción en cadena de la polimerasa (RT-PCR), que amplifica en su totalidad los genes σC- y σNS- codificantes de los reovirus aviares (ARV). Se amplificaron fragmentos de DNA de 1022 y 1152 pares de bases respectivamente a partir de los aislamientos de ARV, lo que indica que no hay deleciones o inserciones aparentes en estas regiones. Los fragmentos amplificados de cepas vacunales y aislamientos de campo se digirieron con cinco enzimas de restricción diferentes Bcn I, Hae III, Taq I, Dde I, and Hinc II, respectivamente. Los patrones de los fragmentos de restricción observados en geles de poliacrilamida mostraron heterogeneicidad entre los aislamientos vacunales y de taiwaneses. Todos los aislamientos de ARV analizados mostraron diferentes patrones de corte de las enzimas de restricción, y podían distinguirse claramente. La tipificación de cepas basada en los lugares de corte en el gen σC-codificante de los ARV mostró que los virus se podían clasificar en cuatro grupos distintos. El árbol filogenético basado en las secuencias nucleotídicas del gen σC-codificante reveló que los aislamientos de ARV de Taiwan se clasificaban en cuatro grupos distintos, lo cual indica que la genotipificación es consistente con la tipificación basada en el polimorfismo de la longitud de los fragmentos de los enzimas de restricción (RFLP) del gen σC-codificante de los ARV. Los resultados sugirieron que la PCR seguida del análisis de la restricción enzimática permitía una simple y rápida aproximación para la caracterización de los aislamientos de ARV. También, que es posible determinar si una nueva cepa variante se ha introducido en una manada o una cepa vírica ya presente se ha diseminado de una manada a otra.
Introduction
Avian reoviruses (ARVs), physically and biochemically similar to mammalian reovirus, have a genome consisting of 10 segments of double-stranded (ds) RNA. The RNA is packaged into a non-enveloped icosahedral double capsid (Spandidos & Graham, Citation1976; Schnitzer et al., Citation1982). The genomic segments can be separated by polyacrylamide gel electrophoresis into three size classes (large, medium, and small). ARVs differ from their mammalian counterparts in their lack of haemagglutination activity (Glass et al., Citation1973), ability to induce cell fusion (Wilcox & Compans, Citation1982), and their association with naturally occurring pathological conditions (Robertson & Wilcox, Citation1986). ARV is an important cause of disease in poultry. In particular, reovirus-induced arthritis, chronic respiratory diseases, and malabsorption syndrome (Fahey & Crawley, Citation1954; Hieronymus et al., Citation1983; Kibenge & Wilcox, Citation1983) cause considerable economic losses.
All ARV-encoded proteins, including eight structural proteins (λA, λB, λC, μA, μBC, σA, σB and σC) and four non-structural proteins (μNS, σNS, P10 and p17), have been demonstrated (Varela & Benavente, Citation1994; Bodelon et al., Citation2001). The σC protein encoded by the ARV S1 gene (Varela & Benavente, Citation1994; Shapouri et al., Citation1995) is 326 amino acids in length and possesses both type-specific and broadly-specific epitopes (Wickramasinghe et al., Citation1993; Shapouri et al., Citation1996). This protein appears to be analogous to mammalian reovirus 1 protein, which has been identified as the virus attachment protein and a haemagglutinin (Schnitzer et al., Citation1982). The σB protein encoded by the ARV S3 gene (Varela & Benavente, Citation1994; Yin et al., Citation1997) is 367 amino acids in length and carries group-specific neutralizing epitopes (Wickramasinghe et al., Citation1993; Shapouri et al., Citation1996). Recently, ARV protein σA encoded by the S2 gene (Yin et al., Citation2000) has been identified as a double-stranded RNA binding protein (Martinez-Costas et al., Citation2000; Yin et al., Citation2000) and with possible involvement in resistance to interferon (Martinez-Costas et al., Citation2000). Another protein of ARV, σNS encoded by the S4 gene (Chiu & Lee, Citation1997), has been reported for its single-stranded RNA binding activity (Yin & Lee, Citation1998).
No reports regarding rapid differentiation and classification of ARV isolates by molecular-based methods have been reported. In this paper, restriction fragment profiles obtained from two cDNA fragments of the σC-encoding and σNS-encoding genes were used to differentiate and classify ARV isolates. ARV strains, including vaccine strains as well as the past and recent Taiwanese strains, were classified into four groups and could be clearly differentiated based on the restriction enzyme fragment length polymorphism (RFLP) patterns.
Materials and Methods
Virus strains and virus propagation
Two commercial vaccine strains of ARV (S1133, Vineland Laboratories; and 2408, Solvay Inc.) and seven local strains (750505, 601SI, 601G, R2/TW, 916, 918, and 1017-1) were used. Based on a cross-neutralization test in Vero cells, S1133, 2408, and 601SI strains have been grouped into serotype I, while 918 represents another serotype (serotype II). The other four ARV strains remain unclassified (Lee et al., Citation1992). ARV strains were propagated in Vero cells. Upon development of 70 to 80% cytopathic effect, the cell cultures were frozen and stored at –70°C. Viral particles were purified as described previously (Liu et al., Citation1999a,Citationb, Citation2002). Briefly, the viruses propagated in Vero cells were concentrated by polyethylene glycol-6000, the pellets resuspended in TNE buffer (0.01 M Tris–HCl [pH 7.6] 0.1 M NaCl and 0.001 M ethylenediamine tetraacetic acid [EDTA]), followed by extraction with Freon (1,1,2-trichlorotrifluoroethane; Sigma Co., St Louis, MO, USA). The supernatants containing virions were centrifuged at 50 000×g at 4°C for 2 h through a 35% sucrose cushion, and the viral pellets obtained were centrifuged isopycnically in stepwise gradients comprising 40%, 30%, and 20% (w/w) CsCl. The virus particles banding at a buoyant density of 1.34 g/ml were withdrawn and concentrated by centrifugation at 132 000×g at 4°C for 2 h.
RNA preparation
Purified viral particles were resuspended in TNE buffer containing 5% sodium dodecyl sulfate. Proteinase K was added to a concentration of 1 mg/ml. After incubation for 1 h at 37°C, the viral RNA was isolated by two consecutive phenol:chloroform:isoamyl alcohol (25:24:1) extractions and recovered by precipitation with ethanol containing 0.4 M LiCl. The dsRNA was further purified by LiCl fractionation precipitation (Diaz-Ruiz & Kaper, Citation1978). Viral RNA was washed twice with 70% ethanol to remove LiCl and suspended in diethylpyrocarbonate-treated water.
Reverse transcription-polymerase chain reaction
To amplify the full-length σC-encoding and σNS-encoding genes of ARV isolates, purified genomic dsRNA from the aforementioned ARV isolates was used to generate cDNA clones by reverse transcription-polymerase chain reaction (RT-PCR). Primers were chosen based on the cDNA sequences of genomic segments S1 and S4 of ARV S1133 (Shapouri et al., Citation1995; Chiu & Lee, Citation1997). Two sets of primer pairs (S1A-S1H and S1A1-S1B1) were used to amplify the ARV σC-encoding gene (1022 base pairs [bp]) of vaccine and field strains. The sequences of S1A1 and S1B1 primers, used to amplify local variant strains, were modified from the primer pair S1A-S1H (Liu et al., Citation1999b, Citation2002). The primer sequences were as follows: S1A, 5′-CTTGTCTTATAGTTCATTGGG-3′ (identical to nucleotides 601 to 621); S1H, 5′-TCCCAGTACGGCGCCACACC-3′ (complementary to nucleotides 1622 to 1603); S1A1, 5′-C/ATTA/GTA/TG/ATTCAT/CTGGGATGG-3′ (identical to nucleotides 601 to 625); S1B1, 5′-T/CCC/GCA/CGT/CACGGCGCCACACC-3′ (complementary to nucleotides 1622 to 1603). Oligodeoxynucleotide primers (S41 and S42) complementary to 24 nucleotides at the 5′ terminus and 24 nucleotides at the 3′ terminus of the ARV S4 gene were used to amplify the full-length cDNA (1152 bp) of the ARV σNS-encoding gene (Liu et al., Citation2003). The primer sequences are as follows: S41, 5′-TTGAATTCTTGTGCAGCCATGGAC-3′ (identical to nucleotides 6 to 29); S42, 5′-GCGAATTCTCACCCGCACCATGGG-3′ (complementary to nucleotides 1157 to 1134). In the RT-PCR test, 1 μg purified dsRNA was denatured in boiling water for 10 min, chilled on ice for 5 min, and then used as a template. RT-PCR reactions were performed according to procedures provided by Perkin Elmer Co. (Branchburg, NJ, USA). Reverse transcription was performed at 50°C for 30 min. PCR reactions were subjected to 35 cycles consisting of denaturation at 94°C for 1 min, annealing at 55°C for 1 min, and extension at 72°C for 2 min and one final extension cycle at 60°C for 7 min.
Analysis of amplified products
After completion of the PCR, 10 μl reaction mixture was loaded onto a 1.2% agarose gel for 1 h in TBE buffer (90 mM Tris–HCl, 90 mM boric acid, 2 mM EDTA, pH 8.3), containing 5 μg/ml ethidium bromide, for electrophoresis and subsequent visualization by ultraviolet transillumination. A DNA ladder of 1 kb was electrophoresed as a size marker for determination of the length of the amplified fragments.
Restriction enzyme analysis of PCR products
The PCR-amplified products were purified according to Magic™ PCR Preps. DNA purification Kit procedures (Promega, Madison, WI, USA). Five enzymes, Bcn I, Hae III, Taq I, Hinc II, and Dde I, were chosen by referring to the published S1 and S4 gene sequences of ARV (Shapouri et al., Citation1995; Chiu & Lee, Citation1997). Each purified cDNA was digested with Bcn I, Hae III, Taq I, Hinc II, and Dde I, respectively. With the exception of the Taq I at 65°C for 3 h, the other enzyme digestions were incubated for 3 h at 37°C in a buffer mixture supplied by the manufacture (Takara Shuzo Co., Shiga, Japan). The digested cDNA was subjected to vertical gel electrophoresis through a 10% polyacrylamide gel using Tris-borate buffer (90 mM Tris-borate, 2 mM EDTA). The DNA fragments on polyacrylamide gels were stained with ethidium bromide, visualized by ultraviolet illumination, and photographed.
cDNA cloning and sequencing
To further confirm the PCR products and the restriction sites in the σC-encoding and σNS-encoding genes of ARV, the amplified cDNA was cloned and sequenced. The full-length σC-encoding and σNS-encoding genes were cloned into pUC18 vectors. PCR products (1022 bp) from the σC-encoding gene of ARV strains were treated with Klenow polymerase and inserted into the Sma I site of pUC18 vector (Sambrook et al., Citation1989). PCR products (1152 bp) derived from the σNS-encoding gene of ARV strains were digested with Eco RI and then ligated into the corresponding site of pUC18 vector. Recombinant plasmid DNA was transformed Escherichia coli competent cells (DH5.). DNA minipreps using an alkaline lysis method were performed on white colonies suspected of containing an insert. Recombinant plasmid DNA was purified using a purification kit (QIAGEN, Valencia, CA, USA). To ensure that the sequences did not contain PCR-based errors, three clones of each gene were sequenced with an Automated Laser Fluorescence DNA Sequencer (ABI).
The nucleotide sequence data of the σC-encoding and σNS-encoding genes reported in the present study have been submitted to the GenBank database and assigned accession numbers: 2408-6C, AF204945; T6-6C, AF204948; OS161-6C, AF204946; 919-6C, AF204949; 601SI-6C, AF204947; 750505-6C, AF204950; 916-6C, AF297214; 601G-6C, AF297217; R2/TW-6C, AF297213; 918-6C, AF297215; 1017-1-6C, AF297216; 2408-.6NS, AF213468; 1733-.6NS, AF294772; T6-.6NS, AF213469; OS161-.6NS, AF294777; 919-6NS, AF294776; 601SI-6NS, AF294773; 750505-6NS, AF213470; 601G-6NS, AF311322; R2/TW-6NS, AF294778; 918-6NS, AF294775; 916-6NS, AF294774; and 1017-1-6NS, AF294771. Accession numbers for other sequences were as follows: S1133-6C, L39002; 1733-6C, AF004857; 176-6C, AF218358; 138-6C, AF218359; and S1133-6NS, U95952.
Phylogenetic analysis
The σC-encoding and σNS-encoding gene nucleotide sequences of ARV were aligned using a Clustal method of DNASTAR software (DNASTAR Inc., Madison, WI, USA). Nucleotide sequences from the C-encoding gene of ARV isolates were used to create a phylogenetic tree for either epidemiological or phylogenetic relationship studies.
Results
RT-PCR and sequence analysis
PCR amplification using primer pairs S1A/S1H and S1A1/ S1B1 to amplify the σC-encoding gene, and primers S41 and S42 to amplify the σNS-encoding genes of ARV, resulted in expected sizes of 1022 bp () and 1152 bp (), respectively. Examination of the amplified PCR products following electrophoresis in agarose gels revealed that all amplified cDNAs showed almost identical mobilities in 1.2% agarose gels. These isolates could not be distinguished on the basis of the length of these amplified cDNAs. Restriction profiles and sequence analysis revealed genetic variations among ARV isolates. No amplification occurred in PCR reactions using only diethylpyrocarbonate-treated water. In addition, misincorporation of nucleotides may occur and result in different RFLP. This possibility was examined by repeating the PCR reactions and restriction enzyme digestion as well as sequencing of amplification products. Therefore, the amplified DNA did not originate from a DNA contamination. No deletions or insertions in the genome segment S1 and S4 of ARV isolates were observed. No fragment could be amplified using primers S1A1 and S1B1 from the S1133, 2408, 750505, and 601SI strains of ARV (). On the other hand, no cDNA fragment could be amplified from the 916, 918, and 1017-1 strains of ARV, when a primer pair of S1A–S1H was used ().
Figure 1. Amplification of the σC-encoding and σNS-encoding genes of ARV isolates. The PCR product (1022 bp) was amplified from the σC-encoding gene of ARV isolates using primer pairs S1A/S1H (1a) and S1A1/ S1B1 (1b). The PCR product (1152 bp) was amplified from the σNS-encoding gene of ARV isolates using primers S41 and S42 (c). Lane M, molecular weight marker of 1 kb DNA ladder; lane 1, S1133; lane 2, 2408; lane 3, 750505; lane 4, 601SI; lane 5, 601G; lane 6, R2/TW; lane 7, 916; lane 8, 918; lane 9, 1017-1; lane C, negative control.
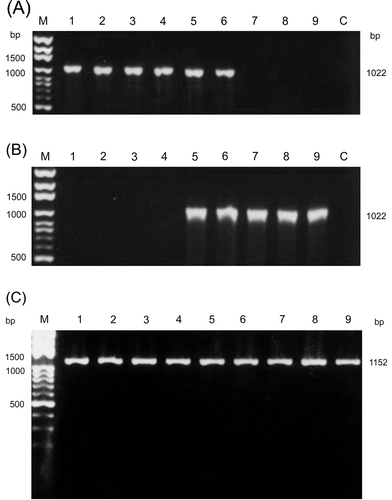
Restriction enzyme digestions of the amplified cDNAs
To differentiate and classify ARV isolates, purified cDNA from each strain was digested with five restriction enzymes. Restriction profiles showed genomic variations among the ARV strains. In the case of Bcn I digestion of σC-encoding gene, the cleavage profiles can be classified into three types ( and ). The first type was a group, which had no cleavage site (S1133, 2408, 750505, 601SI, 601G, and R2/TW strains). The second and third types were two groups with distinct patterns, which had one cleavage site (916, 918, and 1017-1 strains). Digestion of the σNS-encoding gene with Bcn I ( and ) gave five distinct profiles and showed more divergences than that of the σC-encoding gene.
Figure 2. Polyacrylamide gel electrophoretic patterns of the PCR product (1022 bp) from the σC-encoding gene of each isolates digested with restriction enzymes Bcn I (1a), Hae III (1b), Taq I (1c), Dde I (1d), and Hinc II (1e). Lane M, molecular weight marker of 1 kb DNA ladder; lane 1, S1133; lane 2, 2408; lane 3, 750505; lane 4, 601SI; lane 5, 601G; lane 6, R2/TW; lane 7, 916; lane 8, 918; lane 9, 1017-1 The arrows indicate the faint bands. A diagram showing the location of restriction siteis is shown in .
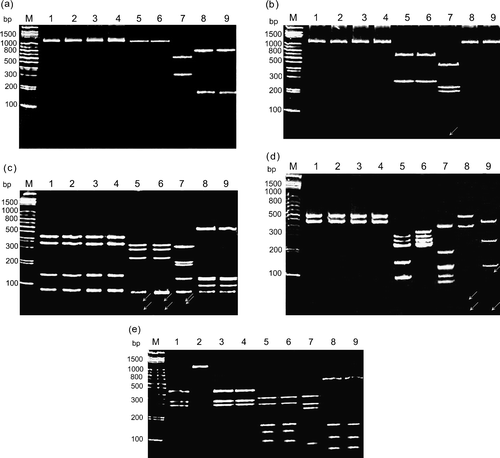
Figure 3. Diagram of restriction enzyme digestions of the σC-encoding gene of ARV isolates. The genome segment S1 of ARV S1133 is indicated by the box. The amplified cDNA fragment (1022 bp) is numbered from 601 to 1622. The restriction sites of the amplified cDNA fragments from each strain are shown as Bcn I (B), Dde I (D), Hae III (Ha), Hinc II (H), and Taq I (T). The classification of ARV isolates is indicated one the right-hand side.
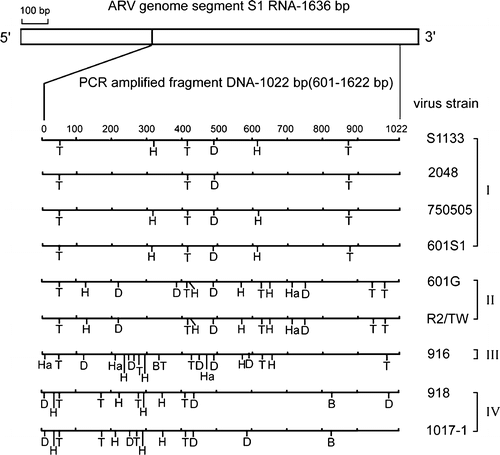
Figure 4. Polyacrylamide gel electrophoretic patterns of the PCR product (1152 bp) from the σNS-encoding gene of each isolate digested with restriction enzymes Bcn I (4a), Hae III (4b), and Taq I (4c). Lane M, molecular weight marker of 1 kb DNA ladder; lane 1, S1133; lane 2, 2408; lane 3, 750505; lane 4, 601SI; lane 5, 601G; lane 6, R2/TW; lane 7, 916; lane 8, 918; lane 9, 1017-1. The arrows indicate the faint bands. A diagram showing the location of restriction site is shown in .
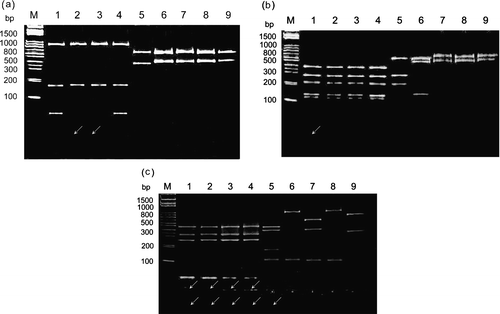
Figure 5. Diagram of restriction enzyme digestions of the σNS-encoding gene of ARV isolates. The genome segment S4 of ARV S1133 is indicated by the box. The amplified cDNA fragment (1152 bp) is numbered from 6 to 157. The restriction sites of the amplified cDNA fragments from each strain are shown as Bcn I (B), Dde I (D), Hae III (Ha), Hinc II (H), and Taq I (T).
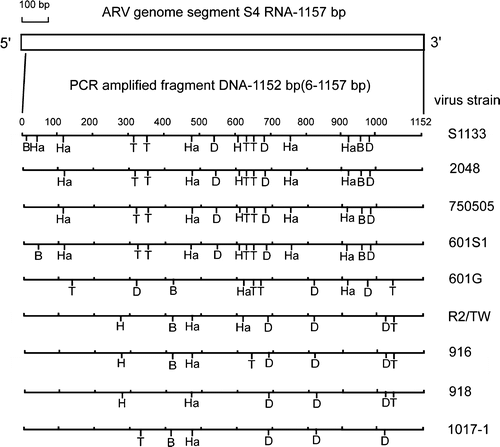
In the case of Hae III digestion of the σC-encoding gene, cleavage profiles could be classified into three types ( and ). The first type is a group, which has no cleavage site (S1133, 2408, 750505, 601SI, 918, and 1017-1 strains). The second type was a group with only one cleavage site (601G and R2/TW). The 916 strain could be classified into the third group with three cleavage sites. In contrast, digestion of the σNS-encoding gene with Hae III ( and ) gave five different profiles and showed more variability than that of the σC-encoding gene.
In the case of Taq I digestion of the σC-encoding gene, cleavage profiles could be classified into four types ( and ). The first type had three cleavage sites (S1133, 2408, 750505, and 601SI strains), the second type had four cleavage sites (918 and 1017-1), and the third type had five cleavage sites (601G and R2/TW). The 916 strain was in the fourth group with six cleavage sites. In the case of Taq I digestion of the σNS-encoding gene, cleavage profiles could be classified into six types ( and ). The first and second types were two distinct groups, which had one cleavage site (R2/TW, 918, and 1017-1 strains). The third type with two cleavage sites included only a 916 strain. The remaining three types with four cleavage sites included the S1133, 2408, 750505, 601SI, and 601G strains.
In the case of Dde I digestion of the σC-encoding gene, cleavage profiles could be classified into six types ( and ). The first type had one cleavage site (S1133, 2408, 750505, and 601SI strains), the second and third types with distinct RFLP patterns had three cleavage sites (R2/TW and 918 strains), and the fourth and fifth types with distinct RFLP patterns had four cleavage sites (601G and 1017-1 strains). The 916 strain was in the sixth group with six cleavage sites. In the case of Dde I digestion of the σNS-encoding gene, cleavage profiles could be classified into three types, which had three cleavage sites in all tested strains and showed more conservation than that of the C-encoding gene ().
In the case of Hinc II digestion of the σC-encoding gene, the cleavage profiles can be classified into five types ( and ). The 2408 strain was in the first group with no cleavage site. The second types had two cleavage sites (S1133, 750505, and 601SI strains). The remaining three types with four cleavage sites included the 601G, R2, 918, 1017-1, and 916 strains. Digestion of the σNS-encoding gene with Hinc II gave three distinct profiles and showed more conservation than that of the C-encoding gene ().
Classification of ARV isolates
Protein σC is structurally equivalent to protein σ1 of mammalian reovirus and is a target for type-specific neutralizing antibodies (Wickramasinghe et al., Citation1993). To obtain better correlation between genetic and serological classifications, differences in cleavage sites in the σC-encoding gene of ARV was used to classify ARV isolates. As shown in , there were 44 cleavage sites with five restriction enzymes in the σC-encoding gene of ARV isolates. For each pair of isolates, differences in restriction sites were counted (0 to 30), as presented in . A low rating (a difference of 0 to 3) signified a group of strong relationships. A medium rating (a difference of 4 to 10) signified a group of moderate relationships. A high rating (a difference of more than 10) signified a group of weak relationships. In the present study, ARV isolates could be classified into four groups, based on cleavage patterns of the σC-encoding gene. There were four low rating areas in . The largest one contains vaccine strains (S1133 and 2408) and local field isolates (750505 and 601SI). Two Taiwanese isolates (750505 and 601SI) were closely related to vaccine strains used in Taiwan. The second low rating area contained the 601G and R2/TW strains, the third only the 916 strains, and the fourth the 918 and 1017-1 strains. Based on the score of different sites, these ARV isolates were classified into at least four groups. The results agree with a phylogenetic profile created on the basis of the σC-encoding gene ().
Figure 6. A phylogenetic tree based on nucleotide sequences of the σC-encoding gene (1022 bp). The ARV isolates in each group are indicated on the right-hand side.
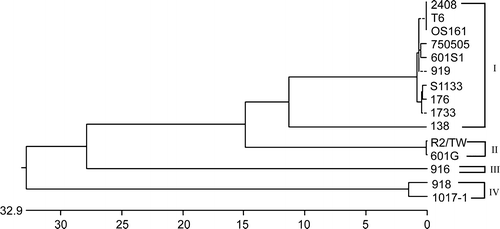
Table 1. Reciprocal restriction sites in the σNS-encoding gene of ARV isolates
Differentiation of ARV isolates
Reciprocal comparison of restriction sites in the σC-encoding and σNS-encoding genes of ARV isolates are presented in and 2. As shown in and , analysis of the amplified DNA fragments by combination of restriction enzyme digestions and cDNA sequencing resulted in a total of 44 and 26 cleavage sites in the σC-encoding and σNS-encoding genes. The distinct types could be differentiated by RFLP patterns of the σC-encoding gene generated by Hae III and Taq I ( and ). Therefore, cleavage patterns by Hae III and Taq I are suitable as makers for differentiation and classification of different ARVs. Restriction profiles showed genomic variations among ARV strains. Although little differences can be detected in some isolates (2408, 750505, and 601SI) with a single enzyme, five endonucleases cleavage profiles of the σC-encoding and σNS-encoding genes made a clear differentiation and classification of different ARVs. Restriction profiles showed genomic variations among ARV strains. Although little differences can be detected in some isolates (2408, 750505, and 601SI) with a single enzyme, combination of five endonucleases cleavage profiles of the σC-encoding and σNS-encoding genes made a clear differentiation possible ( –).
Phylogenetic relationships of ARV isolates
To evaluate evolutionary relationships of ARV isolates, we constructed a phylogenetic tree based on the σC-encoding gene of ARV (). The term lineages, which is defined as a cluster of genetically related viruses with less than 12% nucleotide divergence across the σC-encoding gene nucleotide sequences, has been used for differentiation of genetic linkages of the σC-encoding gene, whereas less than 2% nucleotide divergence between strains has been considered to indicate a direct epidemiological linkage. The σC-encoding gene of ARV has evolved into four distinct lineages. The σC-encoding gene displayed the feature of highest level of sequence divergence and rapid evolution, suggesting that the gene could be used in rapid differentiation and classification of ARV isolates. Nine Taiwanese isolates, isolated in different dates, have evolved into four lineages. Taiwanese strains 750505, 919, 601SI, and T6 as well as a Japanese OS161 strain were clustered into lineage I and phylogenetically related to US vaccine strains, suggesting that they may have originated from a common ancestor.
Discussion
Several methods have been used to identify ARV isolates and detect antibodies against ARV, including immunodiffusion, virus neutralization, enzyme-linked immunosorbent assay, in situ hybridization, in situ RT-PCR, and immunoblot assays (Sahu & Olson, Citation1975; Slaght et al., Citation1978, Citation1979; Lee et al., Citation1994; Liu et al., Citation1999a,Citationb, Citation2000, Citation2002). ARV has multiple serotypes, and new variant serotypes continue to be recognized (Takase et al., Citation1987; Giambrone & Solano, Citation1988; Lee et al., Citation1992). When designing a vaccination programme for ARV, it is important to identify the serotype of field strains, as cross-protection may be limited between different serotypes. The classification of ARV strains has been performed serologically using cross-neutralization tests (Giambrone, Citation1980; Giambrone & Solano, Citation1988; Lee et al., Citation1992). However, classification of ARV isolates is not well established. Because serological analyses of viruses are influenced by various factors, such as standardization of the procedure and method of antiserum production, more tests with monoclonal antibodies raised against different serotypes will be required to confirm the existence of truly distinct serotypes. Furthermore, ARV isolates can rapidly evolve, resulting in a wider heterogeneity in pathogenicity and their neutralizing antigens as well as considerable cross-reaction among heterologous types (Clark et al., Citation1990; Robertson & Wilcox, Citation1986; Rosenberger et al., Citation1989; Sterner et al., Citation1989). To meet the need for a more practical and accurate method of identifying ARV isolates, PCR-RFLP and phylogenetic analysis were established to characterize vaccine and field isolates of ARV.
In recent years, molecular-based methods were effective in the characterization of viruses (Lin et al., Citation1991; Heppell et al., Citation1992; Liu et al., Citation1994, Citation1999a,Citationb). Differentiation and classification of ARV isolates have yet to be established at the genomic level. In the present study, both σC-encoding and σNS-encoding genes were used as markers to differentiate ARV isolates based on previous observations, that the ARV genome segments S1 and S4 showed higher variability than other genome segments (Liu et al., Citation2003). Genetic divergence made it possible to rapidly differentiate ARV isolates, based on restriction profiles of S1 and S4 genomic segments. Therefore, both genes were appropriate for differentiating ARV isolates and studying genomic variations. Although amplified cDNAs were almost identical in size, many variations were observed in the RFLP patterns among ARV isolates. This allowed vaccine strains to be differentiated from local field isolates. Therefore, the PCR-RFLP provided a rapid and useful tool for studying ARV isolates in the field and estimating the origin of an outbreak strain.
It is well known that neutralizing and serotype-specific epitopes are located on the σC of ARV (Wickramasinghe et al., Citation1993; Shapouri et al., Citation1996), but their exact location has yet to be determined. By working on the full-length σC-encoding gene, which is responsible for serotype specificity in ARV, it is possible to obtain a better correlation between genetic and serological classification. It follows that the RE sites identified in the present study may be located in areas within the σC-encoding gene that are important in determining the serotype of the virus. In the present study, a cluster analysis of RFLP patterns and phylogenetic analysis showed that ARV strains could be divided into four groups (groups I to IV) ( and ). The RFLP data and phylogenetic analysis indicated that Taiwanese variant isolates 601G, R2/TW, 916, 918, and 1017-1 belong to groups II, III, and IV, which are distantly related to group I viruses (S1133, 2408, 1733, 919, OS161, 750505, 601SI, 176, and 138 strains). The 750505, 601G, 916, and 1017-1 strains, which had not been serologically classified, were distinct from each other and belonged to groups I, II, III, and IV, respectively. These data were consistent with serologically classification using the virus neutralization test (Lee et al., Citation1994). It is interesting to note that Taiwanese strains T6, 750505, 919, and 601SI were closely related to US vaccine strains, based on RFLP patterns, sequences of the σC-encoding gene, and phylogenetic analysis, suggesting that they might have originated from a common ancestor. Since the strain typing based on a cross-neutralization test is not yet well established, it is difficult to relate the observed lineages for σC to the serotype assignments of these viruses.
Results showed that PCR-RFLP and phylogenetic analysis provided a valuable approach for the differentiation and classification of ARV isolates. This technique will aid in the study of the epidemiology of the virus.
Acknowledgments
This work was supported by grants from the National Science Council (NSC91-2313-B-020-029 and NSC92-2313-B-020-016), Taiwan.
References
- Bodelon , G , Labrada , L , Martinez-Costas , J and Benavente , J . 2001 . The avian reovirus genome segment S1 is a functionally tricistronic gene that express one structural and two nonstructural proteins in infected cells . Virology , 290 : 181 – 191 .
- Chiu , CJ and Lee , LH . 1997 . Cloning and nucleotide sequencing of the S4 genome segment of avian reovirus S1133 . Archives of Virology , 142 : 2515 – 2520 .
- Clark , FD , Ni , Y , Collisson , EW and Kemp , MC . 1990 . Characterization of avian reovirus strain-specific polymorphism . Avian Diseases , 34 : 304 – 314 .
- Diaz-Ruiz , JR and Kaper , JM . 1978 . Isolation of viral double-stranded RNAs using a LiCl fractionation procedure . Preparative Biochemistry , 8 : 1 – 17 .
- Fahey , JE and Crawley , JF . 1954 . Studies on chronic respiratory disease of chickens II. Isolation of a virus . Canadian Journal of Comparative Medicine , 18 : 13 – 21 .
- Giambrone , JJ . 1980 . Microculture neutralization test for serodiagnosis of three avian viral infections . Avian Diseases , 24 : 284 – 287 .
- Giambrone , JJ and Solano , W . 1988 . Serological comparison of avian reovirus isolates using virus neutralization and an enzyme-linked immunosorbent assay . Avian Diseases , 32 : 678 – 680 .
- Glass , SE , Naqi , SA , Hall , CF and Kerr , KM . 1973 . Isolation and characterization of a virus associated with arthritis of chickens . Avian Diseases , 17 : 415 – 424 .
- Heppell , J , Berthiaume , L , Trarrab , E , Lecomte , J and Arella , M . 1992 . Evidence of genomic variations between infectious pancreatic necrosis virus strains determined by restriction fragment profiles . Journal of General Virology , 73 : 2863 – 2870 .
- Hieronymus , DRK , Villegas , P and Kleven , SH . 1983 . Identification and serological differentiation of several reovirus strains isolated from chickens with suspected malabsorption syndrome . Avian Diseases , 27 : 246 – 254 .
- Kibenge , FSB and Wilcox , GE . 1983 . Tenosynovitis in chickens . Veterinary Bulletin , 53 : 431 – 443 .
- Lee , LH , Wang , YH and Shien , JH . 1992 . Serological characterization of reoviruses isolates from avian species in Taiwan . Journal of Chinese Society of Veterinary Science , 18 : 69 – 72 .
- Lee , LH , Wang , YW and Shien , JH . 1994 . Comparison of the labeled avidin–biotin and the conventional enzyme-linked immunosorbent assay for detecting antibody to reovirus in chickens . Journal of Virological Methods , 48 : 343 – 347 .
- Lin , Z , Kato , A and Ueda , S . 1991 . A new typing method for the avian infectious bronchitis virus using polymerase chain reaction and restriction enzyme fragment length polymorphism . Archives of Virology , 116 : 19 – 31 .
- Liu , HJ , Giambrone , JJ and Dormitorio , T . 1994 . Detection of genetic variations in serotype I isolations of infectious bursal disease virus using polymerase chain reaction and restriction endonuclease analysis . Journal of Virological Methods , 48 : 281 – 291 .
- Liu , HJ , Liao , MH , Chang , CD , Chen , JH , Lin , MY and Tung , MC . 1999a . Comparison of two techniques for the detection of avian reoviruses in formalin-fixed paraffin-embedded chicken tissues . Journal of Virological Methods , 80 : 197 – 201 .
- Liu , HJ , Chen , JH , Liao , MH , Lin , MY and Chang , GN . 1999b . Identification of the C-encoded gene of avian reovirus by nested PCR and restriction endonuclease analysis . Journal of Virological Methods , 81 : 83 – 89 .
- Liu , HJ , Giambrone , JJ , Wu , YH , Liao , MH and Lu , CF . 2000 . The use of monoclonal antibody probes for the detection of avian reovirus antigens . Journal of Virological Methods , 86 : 115 – 119 .
- Liu , HJ , Kuo , LC , Hu , YC , Liao , MH and Lien , YY . 2002 . Development of an ELISA for detection of antibodies to avian reovirus in chickens . Journal of Virological Methods , 102 : 129 – 138 .
- Liu , HJ , Lee , LH , Hsu , HW , Kuo , LC and Liao , MH . 2003 . Molecular evolution of avian reovirus: evidence for genetic diversity and reassortment of the S-class genome segments and multiple cocirculating lineages . Virology , 314 : 336 – 349 .
- Martinez-Costas , J , Gonzalez-Lepez , C , Vakharia , VN and Benabente , J . 2000 . Possible involvement of the double-stranded RNA-binding core protein A in the resistance of avian reovirus to interferon . Journal of Virology , 74 : 1124 – 1131 .
- Robertson , MD and Wilcox , GE . 1986 . Avian reovirus . Veterinary Bulletin , 56 : 154 – 174 .
- Rosenberger , JK , Sterner , FJ , Botts , KP , Lee , KP and Margolin , A . 1989 . In vitro and in vivo characterization of avian reoviruses. I. Pathogenicity and antigenic relatedness of several avian reovirus isolates . Avian Diseases , 33 : 535 – 544 .
- Sahu , SP and Olson , NO . 1975 . Comparison of the characteristics of avian reovirus isolated from the digestive and respiratory tract, with viruses isolated from the synovia . American Journal of Veterinary Research , 36 : 847 – 850 .
- Sambrook J Maniatis T Fritsch EF 1989 Molecular Cloning:A Laboratory Manual 2nd edn Cold Spring Harbour NY Cold Spring Harbour Laboratory
- Schnitzer , TJ , Ramos , T and Gouvea , V . 1982 . Avian reovirus polypeptides: analysis of intracellular virus-specified products of virion, top component, and cores . Journal of Virology , 43 : 1006 – 1014 .
- Shapouri , MRS , Kane , M , Letarte , M , Bergeron , J , Arella , M and Silim , A . 1995 . Cloning, sequencing and expression of the S1 gene of avian reovirus . Journal of General Virology , 76 : 1515 – 1520 .
- Shapouri , MRS , Frentte , D , Larochelle , R , Arella , M and Silma , A . 1996 . Characterization of monoclonal antibodies against avian reovirus strain S1133 . Avian Pathology , 25 : 57 – 67 .
- Slaght , S , Yang , TJ , Van der Heide , L and Fredrickson , TN . 1978 . An enzyme-linked immunsorbent assay (ELISA) for detecting chicken anti-reovirus antibody at high sensitivity . Avian Diseases , 22 : 801 – 805 .
- Slaght , SS , Yang , TJ and Van der Heide , L . 1979 . Adaptation of enzyme-linked immunosorbent assay to the avian system . Journal of Clinical Microbiology , 10 : 698 – 700 .
- Spandidos , DA and Graham , AF . 1976 . Physical and chemical characterization of an avian reovirus . Journal of Virology , 19 : 968 – 976 .
- Sterner , FJ , Rosenberger , JK , Margolin , A and Ruff , MD . 1989 . In vitro and in vivo characterization of avian reovirus. II. Clinical evaluation of chickens infected with two avian reovirus pathotypes . Avian Diseases , 33 : 545 – 554 .
- Takase , K , Nonaka , F , Yamamoto , MF and Yamada , S . 1987 . Serological and pathogenic studies on avian reoviruses isolated in Japan . Avian Diseases , 31 : 464 – 469 .
- Varela , R and Benavente , J . 1994 . Protein coding assignment of avian reovirus S1133 . Journal of Virology , 68 : 6775 – 6777 .
- Wickramasinghe , R , Menager , J , Enriques , CE and Wilcox , GE . 1993 . Avian reovirus proteins associated with neutralization of virus infectivity . Virology , 194 : 688 – 696 .
- Wilcox , GE and Compans , RW . 1982 . Cell fusion induced by Nelson Bay virus . Virology , 123 : 312 – 322 .
- Yin , HS , Shieh , HK and Lee , LH . 1997 . Characterization of the double-stranded RNA genome segment S3 of avian reovirus . Journal of Virological Methods , 67 : 93 – 101 .
- Yin , HS and Lee , LH . 1998 . Identification and characterization of RNA-binding activities of avian reovirus non-structural protein.NS . Journal of General Virology , 79 : 1411 – 1413 .
- Yin , HS , Shien , JH and Lee , LH . 2000 . Synthesis in Escherichia coli of avian reovirus core protein A and its dsRNA-binding activity . Virology , 266 : 33 – 41 .