Abstract
In the past, we showed differences in heterophil function between parental broilers (A [fast feathering] > B [slow feathering]) and their F1 reciprocal crosses (D [fast feathering] > C [slow feathering]). In the present study, we evaluated the linkage of the feathering gene to heterophil function, pro-inflammatory cytokine/chemokine mRNA expression levels, and resistance to Salmonella enteritidis organ invasion. Heterophils were isolated from 2-day-old chickens (C and D) separated into males and females—slow males and females (SM and SF), and fast males and females (FM and FF). Heterophil functions of degranulation and oxidative burst were measured. Heterophils from FF chickens (183±8.9) released more (P < 0.05) β-d-glucuronidase (µM) than heterophils from SF chickens (149±3.7); FF heterophils (4.6×104) generated a significantly (P < 0.05) greater oxidative burst (mean relative fluorescent units) compared with SF heterophils (4.2×104). Interleukin-6, CXCLi2, and interferon-α mRNA expression levels were quantitated by real-time quantitative reverse transcriptase-polymerase chain reaction. No differences were observed between SM and FM or between SF and FF heterophils. Finally, 1-day-old chickens were administered S. enteritidis and liver/spleen organ invasion was quantitated. No differences were observed between the number of S. enteritidis-positive FF and SF chickens, but FM were significantly (P < 0.05) more resistant to S. enteritidis organ invasion than SM chickens. The data indicate degranulation and oxidative burst were linked with the feathering gene; however, interleukin-6, CXCLi2, and interferon-α mRNA expression levels were not. Furthermore, susceptibility to in vitro S. enteritidis organ invasion was not linked to the feathering gene.
Dans le passé, nous avons démontré des différences dans la fonction hétérophile entre les parentales des poulets de chair (A [emplumement rapide] >B [emplumement lent]) et leurs croisements réciproques de leurs descendants à la génération F1 (D [emplumement rapide] >C [emplumenment lent]). Dans la présente étude, nous avons évalué la relation entre le gène de l'emplumement et la fonction hétérophile, les niveaux d'expression de l'ARNm des cytokines/chémokines pro-inflammatoires et de la résistance à l'invasion des organes par Salmonella enteritidis (SE). Les hétérophiles ont été isolés chez les poussins C et D, mâles et femelles âgés de deux jours (mâles et femelles à emplumement lent [SM et SF] et mâles et femelles à emplumement rapide [FM et FF]). Les fonctions hétérophiles de dégranulation et d'explosion respiratoire ont été mesurées. Les hétérophiles des poussins FF (183±8,9) ont libéré plus de β-D-glucuronidase (µM) que les hétérophiles des poulets SF (149±3,7), (P<0,05); les hétérophiles des poulets FF (4,6×104) ont généré significativement plus d'explosion respiratoire (moyenne RFU) comparés aux hétérophiles de poulets SF (4,2×104), (P<0,05). Les niveaux d'expression de l'ARNm de l'interleukine (IL)-6, du CXCLi2, et de l'interféron (IFN)-α ont été mesurés par RT PCR quantitative en temps réel. Aucune différence n'a été observée entre les hétérophiles des poulets SM et FM ou entre ceux des poulets SF et FF. Enfin, les poussins âgés de deux jours qui ont reçu SE, ont fait l'objet d'une quantification de l'invasion du foie et de la rate par la bactérie. Aucune différence n'a été observée entre le nombre de poulets FF et SF positifs vis-à-vis de SE mais les poussins FM ont été significativement plus résistants à l'invasion des organes par SE que les poulets SM (P<0,05). Ces données indiquent que la dégranulation et l'explosion respiratoire sont liées au gène de l'emplumement; cependant les niveaux d'expression de l'ARNm de l'IL-6, du CXCLi2, et de l'IFN-a ne le sont pas. De plus, la sensibilité à l'invasion des organes in vitro n'est pas liée au gène de l'emplumement.
In vorherigen Untersuchungen konnten wir Unterschiede in der Heterophilenfunktion zwischen Mastelterntieren (A [schnell befiedernd] >B [langsam befiedernd]) und ihren reziproken F1-Kreuzungsprodukten (D [schnell befiedernd] >C [langsam befiedernd]) darstellen. In der jetzigen Studie untersuchten wir die Verbindung des Befiederungsgens zur Heterophilenfunktion, zu den pro-inflammatorischen Zytokin/Chemokin-mRNS-Expressionsgehalten und zur Resistenz gegenüber der Salmonella Enteritidis (SE)-Organbesiedlung. Aus zwei Tage alten in männliche und weibliche (langsam befiedernde männliche und weibliche [SM und SF] und schnell befiedernde männliche und weibliche [FM und FF]) getrennte Hühnerküken (C und D) wurden Heterophile isoliert. Die Heterophilenfunktionen der Degranulierung und des oxidativen Ausbruchs wurden bei diesen Zellen bestimmt. Die Heterophilen der FF-Hühnerküken bildeten mehr (p < 0,005) β-D-Glucoronidase (183±8,9 µM) als die Heterophilen der SF-Küken (149±3,7); die FF-Heterophilen (4,6×104) erzeugten im Vergleich zu den SF-Heterophilen (4,2×104) einen signifikant größeren (p < 0,05) oxidativen Ausbruch (mittlerer RFU). Die Interleukin (IL)-6-, CXCLi2- und Interferon (IFN)-α-mRNS-Expressionswerte wurden mittels quantitativer Real Time-RT-PCR ermittelt. Es wurden keine Differenzen zwischen den Heterophilen der SM- und FM- bzw. zwischen den SF- und den FF-Hühnerküken festgestellt. Schließlich wurden Eintagsküken mit SE inokuliert und die Leber/Milz-Organbesiedlung quantifiziert. Zwischen der Anzahl der SE-positiven FF- und SF-Küken bestand kein Unterschied, aber die FM-Küken waren gegenüber der SE-Organbesiedlung signifikant resistenter (p < 0,05) als die SM-Küken. Diese Daten weisen darauf hin, dass zwischen dem Befiederungsgen und der Degranulierung sowie dem oxidativen Ausbruch eine Verbindung besteht, während die IL-6, CXCLi2- und IFN-a-mRNS-Expression nicht mit diesem Gen verbunden sind. Außerdem konnte keine Verbindung zwischen dem Befiederungs-Gen und der Empfänglichkeit gegenüber einer SE-Organbesiedlung.
Anteriormente, mostramos las diferencias en las funciones heterofílicas entre pollos de engorde parentales (A [emplume rápido] >B [emplume lento]) y sus cruces recíprocos F1 (D [emplume rápido] >C [emplume lento]). En este estudio, evaluamos la relación del gen del emplume con la función heterofílica, los niveles de expresión de mRNA de citoquinas/quimioquinas proinflamatorias, y la resistencia a la invasión de órganos por Salmonella enteritidis (SE). Los heterófilos se aislaron de pollos de dos días de vida (C y D) separados en machos y hembras (machos y hembras lentos [SM y SF] y machos y hembras rápidos [FM y FF]). Se valoraron las funciones heterofílicas de degranulación y estallido oxidativo. Los heterófilos de pollos FF (183±8.9) excretaron más (P<0.05) β-D-glucuronidasa (µM) que los heterófilos de pollos SF(149±3.7); los heterófilos FF (4.6×104) generaron un estallido oxidativo (media RFU) significativamente mayor (P<0.05) que los heterófilos SF (4.2×104). Se cuantificaron los niveles de expresión de mRNA de interleuquina (IL)-6, CXCLi2, e interferón (IFN)-α mediante RT-PCR cuantitativa a tiempo real. No se observaron diferencias entre heterófilos SM y FM o entre heterófilos SF y FF. Finalmente, se administró SE a pollos de un día de vida y se cuantificó la invasión de hígado/bazo. No se observaron diferencias significativas entre el número de pollos FF y SF positivos a SE, pero los FM fueron significativamente más resistentes (P<0.05) a la invasión de órganos por SE que los pollos SM. Estos resultados indican que la degranulación y el estallido oxidativo están ligados con el gen del emplume; sin embargo los niveles de expresión de mRNA de IL-6, CXCLi2, y IFN-α no lo están. Además, la susceptibilidad a la invasión de órganos in vitro de SE no estuvo ligada al gen del emplume.
Introduction
The poultry industry suffers major economic losses from both mortality due to infectious diseases and potential contamination of food products due to transfer of microorganisms. Our laboratories have been evaluating the innate immune system of pedigree broilers in order to assess their capacity to protect against pathogen invasion, including Salmonella enteritidis. We have extensively characterized the innate immune response of two parental broiler lines—A (fast feathering) and B (slow feathering). To date, we have shown that an increased in vitro heterophil function (Swaggerty et al., Citation2003a,Citationb) corresponds with an increased in vivo resistance to organ invasion by Gram-positive (Swaggerty et al., Citation2005b) and Gram-negative bacterial infections (Ferro et al., Citation2004; Swaggerty et al., Citation2005a). In addition, we have shown increased mRNA expression levels of pro-inflammatory cytokines in heterophils isolated from the more resistant lines compared with the susceptible lines (Swaggerty et al., Citation2004). Straight-run, not separated by sex, F1 reciprocal crosses (C = B sire×A dam; D = A sire×B dam) have a differential heterophil response (D [fast feathering] > C [slow feathering]) (Swaggerty et al., Citation2003b) and cytokine profile (Ferro et al., Citation2004). Collectively, our data indicate there are clear and measurable differences in heterophil function and innate response that are under specific genetic control.
The feathering gene is located on the short arm of the Z sex chromosome and codes for feather growth. The fast feather allele, k, is the wild-type recessive trait responsible for rapid feather growth, whereas the slow feather allele, K, is dominant and responsible for slow feather growth. Our previous results suggest the presence of the fast feathering gene (k) may enhance heterophil functional efficiency while the slow feathering gene (K) may reduce the functional efficiency of isolated heterophils.
Based on in vitro heterophil functional differences, we decided to evaluate chickens from the F1 reciprocal crosses separated by sex to determine whether in vitro heterophil function is linked to or merely associated with the feathering gene. The objective of this study was to determine whether there was linkage between the feathering gene and the in vitro heterophil functions of degranulation and oxidative burst, cytokine/chemokine mRNA expression levels in heterophils, or in vivo organ invasion by S. enteritidis. With respect to the feathering gene, the F1 reciprocal cross males are the same in both lines (slow males [SM] and fast males[FM] = K/k) whereas the females are different (slow females [SF] = K/–; fas females [FF] = k/–). Therefore, if in vitro heterophil functional efficiency was associated with the fast feathering gene, we would expect heterophils from FF to be functionally more efficient than heterophils from SF, while the functional efficiency of heterophils from SM and FM would be the same.
Materials and Methods
Chickens
F1 reciprocal cross chickens (F1 cross C = B sire×A dam; F1 cross D = A sire×B dam) were hatched at a commercial hatchery and separated into males and females. F1 cross D chickens were feather sexed, while F1 cross C chickens were vent sexed. The male and female progeny from slow feathering cross C were referred to as slow male (SM) and slow female (SF), while the fast feathering cross D males and females were FM and FF, respectively. With respect to the feathering gene, the F1 reciprocal cross males are the same in both lines (SM and FM = K/k + ) whereas the females are different (SF = K/–; FF = k + /–). Chickens were transported to our facility on the day of hatch in standard chick boxes containing hydration gel packs in an enclosed, temperature-controlled vehicle. Upon arrival chickens were placed in 2m×2m floor pens containing wood shavings, provided supplemental heat, water, and un-medicated corn and soybean meal-based chick starter diet ad libitum. The feed met or exceeded standards established by the National Research Council (Citation1994).
Bacteria
A poultry isolate of S. enteritidis (#97-11771) was obtained from the National Veterinary Services Laboratory (Ames, Iowa, USA) and approved by the United States Department of Agriculture (USDA). S. enteritidis was cultured in tryptic soy broth (Difco Laboratories, Becton Dickinson Co., Sparks, Maryland, USA) overnight at 41°C. Stock S. enteritidis (1×109 colony forming units [CFU]/ml) were prepared as previously described (Swaggerty et al., Citation2003b). S. enteritidis was prepared in RPMI or phosphate-buffered saline for in vitro and in vivo assays, respectively. S. enteritidis were opsonized with normal chicken serum (NCS-OpSE) as previously described (Kogut et al., Citation2001).
Heterophil isolation
Heterophils were isolated from peripheral blood of 150 chickens per group, 2 days post-hatch. Blood was collected in vacutainer tubes containing disodium ethylenediamine tetraacetic acid (BD vacutainer, Franklin Lakes, New Jersey, USA) and mixed thoroughly. Following blood collection, heterophils were isolated as previously described (Swaggerty et al., Citation2003b). Heterophil preparations were consistently 95% pure and > 95% viable. All tissue culture reagents and chemicals were obtained from Sigma Chemical Company (St Louis, Missouri, USA), unless otherwise noted.
Phagocytosis
Heterophils were assayed for the ability to phagocytize live S. enteritidis as previously described (Kogut et al., Citation2001). Briefly, heterophils (2 ml of 5×106 cells/ml) and S. enteritidis (2 ml of 5×107 CFU/ml) were added to diluted heterophils (10 S. enteritidis:1 heterophil) and centrifuged at 2000×g for 15 min. The pelleted heterophils and S. enteritidis were incubated 1 h at 39°C and then submerged in ice for 15 min. The samples were washed with equal volume of ice-cold RPMI and centrifuged at 2000×g for 15 min. The pellets were resuspended in ice-cold gentamicin (100 µg/ml in RPMI) and incubated for 1 h at 37°C on a rotary shaker. Heterophils were washed three times with ice-cold RPMI (3 to 5 ml per wash and centrifuged at 2000×g for 15 min), and the pellet was re-suspended in 2 ml RPMI. Cytospin smears were prepared from each sample in a Shandon Cytospin 3 (Shandon Inc., Pittsburgh, Pennsylvania, USA), stained with Hematology 3-step stain (Biochemical Sciences, Inc., Swedesboro, New Jersey, USA), and examined by light microscopy with an oil immersion objective (100×).
Degranulation
The release of intracellular granules was monitored by quantifying the amount (µM) of β-d-glucuronidase in the supernatant of heterophils as previously described (Kogut et al., Citation2001). Heterophils (8×106 cells/ml) were treated with OpSE or RPMI for 1 h at 39°C on a rotary shaker. The samples were immersed in ice for 10 min. Cells were removed by centrifugation (250×g for 15 min at 4°C), the supernatants collected then assayed for β-d-glucuronidase activity. Briefly, samples and standards (25 µl) were added to a black flat-bottom enzyme-linked immunosorbent assay plate and incubated with 50 µl freshly prepared substrate (10 mM 4-methylumbelliferyl-β-d-glucuronide dissolved in 0.1 M sodium acetate buffer containing 0.1% Triton X-100; pH 4.0) for 4 h at 41°C. The reaction was stopped by the addition of 200 µl stop solution (0.05 M glycine, 5 mM ethylenediamine tetraacetic acid; pH 10.4). Released 4-methylumbelliferone was measured on a GENios Plus Fluorescence Microplate Reader (TECAN US Inc, Research Triangle Park, North Carolina, USA) (excitation = 355 nm, emission = 460 nm) and converted using a standard curve of known concentrations.
Oxidative burst
Production of reactive oxygen species by heterophils during oxidative burst was measured by oxidation of DCFH-DA to fluorescent DCF as previously described (He et al., Citation2003). Briefly, heterophils (1 ml, 8×106 cells/ml) were incubated with 5% chicken serum, DCFH-DA (10 µg/ml), and phorbol A-myristate 13-acetate (1 µg/ml) for 1 h at 37°C and 5% CO2. Heterophils (150 µl) were then dispensed to a black 96-well plate and the fluorescence intensity measured using a GENios Plus Fluorescence Microplate Reader at 485 nm excitation and 530 nm emission wavelengths (manually set gain at 50). The mean relative fluorescent units (RFU) were recorded after 1 h.
RNA isolation
Heterophils (1×107) were treated with 300 µl RPMI or S. enteritidis, for 30 min at 39°C on a rotary shaker. Treated heterophils were pelleted, washed with RPMI (485×g for 15 min at 4°C), the supernatant discarded, the cells re-suspended in lysis buffer (Qiagen RNeasy mini RNA extraction kit; Qiagen Inc., Valencia, California, USA), and frozen. Total RNA was extracted from homogenized (QIAshredder columns) lysates according to the manufacturer's instructions.
Quantitative real-time reverse transcriptase-polymerase chain reaction
Cytokine/chemokine mRNA expression levels in control and agonist-treated heterophils were quantitated using a method described by Kaiser and colleagues (Kaiser et al., Citation2000). Primers and probes have been described previously (Kaiser et al., Citation2000; Kogut et al., Citation2003; Swaggerty et al., Citation2004). Quantitative reverse transcriptase-polymerase chain reaction (RT-PCR) was performed using the Reverse Transcriptase qPCR Master Mix RT-PCR kit (Eurogentec, San Diego, California, USA). Amplification and detection of products were performed using the ABI PRISM 7700 Sequence Detection System (PE Applied Biosystems, Warrington, UK). All samples were standardized using 28S RNA. Results were shown as control 40-cycle threshold (Ct), S. enteritidis-treated 40-Ct values, and the fold-change from control. Fold change is calculated using the following formula 2(S. enteritidis corrected mean – control corrected mean).
Organ invasion
Thirty 2-day-old chickens from each group were challenged orally with 5×106 CFU S. enteritidis (0.5 ml, 1×107 CFU/ml stock). Twenty-four hours post-challenge, chickens were euthanized and necropsied. At necropsy, livers and spleens were aseptically removed and incubated overnight at 41°C in tetrathionate enrichment broth. Following enrichment, 10 µl were streaked ontobrilliant green agar plates with carbenicillin and novobiocin, incubated 24 h at 41°C, then the plates examined for non-lactose fermenting carbenicillin- and novobiocin-resistant Salmonella colonies. Representative colonies were confirmed S. enteritidis-positive by plate agglutination using specific Group D1 antisera (Difco Laboratories). Non-challenged chickens from each group were included as negative controls. Three experimental trials were conducted and the data pooled for presentation and statistical analysis.
Statistical analyses
Anti-coagulated blood from 150 chickens per group was pooled and heterophils isolated for the in vitro assays. Each blood collection and heterophil isolation was conducted on three separate days; therefore, heterophils were pooled from a total of 450 chickens. Data from 90 chickens per line (30 for three separate challenges) were used for S. enteritidis organ invasion. For both in vitro and in vivo studies, the mean and standard error of the mean were calculated from pooled data. Comparisons were made only between the females (SF and FF), and then a separate analysis for males (SM and FM). Statistical analyses (Student's t test) were performed using Microsoft® Excel 2000 (P < 0.05).
Results
Phagocytosis
Heterophils isolated from FF, FM, SF, and SM chickens were evaluated for the ability to phagocytize S. enteritidis. Specifically the percentage of heterophils with S. enteritidis and the average number of S. enteritidis per heterophil was determined. There were no differences between any of the groups with regard to percentage of heterophils that engulfed S. enteritidis, nor in the number of S. enteritidis per heterophil. The percentage of heterophils from males and females that engulfed S. enteritidis was all between 78.7 and 80.7%, and all groups phagocytized seven to nine S. enteritidis per heterophil (data not shown).
Degranulation
The ability of heterophils to release bactericidal granules (degranulation) following stimulation was also measured in heterophils isolated from FF, FM, SF, and SM chickens (). Heterophils isolated from FF chickens released a significantly (P < 0.05) higher concentration (µM) of β-d-glucuronidase, the enzyme associated with heterophil-mediated degranulation, than heterophils from SF chickens (183±8.9 and 149±3.7 µM, respectively). Comparisons between heterophils from FM and SM showed no differences in degranulation (156±5.1 and 146±2.2 µM, respectively).
Figure 1. Degranulation of heterophils isolated from 2-day-old chickens was compared to determine their ability to release toxic granules when stimulated with opsonized S. enteritidis. Data are pooled and presented as the mean±standard error of the mean of the µM β-d glucuronidase released from triplicate assays from three separate experiments (n = 450 chickens). All statistical differences are for comparisons between females (FF vs SF) or between males (FM vs SM) (*P < 0.05).
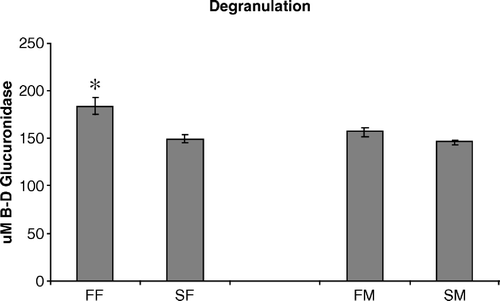
Oxidative burst
Oxidative burst was measured in heterophils from FF, SF, FM, and SM chickens following stimulation with phorbol A-myristate 13-acetate (). As seen with degranulation, heterophils from FF chickens underwent a significantly greater (P < 0.05) oxidative burst response compared with heterophils from SF chickens (4.6×104 and 4.2×104 RFU, respectively). Additionally, there were no differences in the oxidative burst response between heterophils isolated from the males (FM = 4.1×104 RFU; SM = 4.0×104 RFU).
Figure 2. Heterophils isolated from FF, SF, FM, and SM 2-day-old chickens were compared to determine their ability to generate an oxidative burst response following stimulation with the inflammatory agonist phorbol A-myristate 13-acetate. Data are pooled and presented as the mean±standard error of the mean of the RFU of triplicate assays from three separate experiments (n = 450 chickens). All statistical differences are for comparisons between females (FF vs SF) or between males (FM vs SM) (*P < 0.05).
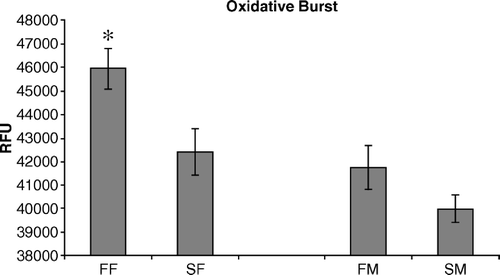
Cytokine mRNA expression
We also evaluated heterophils for mRNA expression levels of the pro-inflammatory cytokine interleukin (IL)-6, the pro-inflammatory chemokine CXCLi2 (formerly IL-8), and interferon (IFN)-α (). There were no differences in the control or S. enteritidis-stimulated mRNA expression levels of IL-6, CXCLi2, or IFN-α between heterophils from the FF and SF chickens, therefore indicating there was no linkage with the feathering gene. Likewise, no differences were observed for IL-6 and CXCLi2 for FM and SM heterophils (control or S. enteritidis-stimulated). There was a statistical (P < 0.05) difference in the IFN-α mRNA expression levels observed between the control males (FM > SM) and a change in mRNA expression levels following S. enteritidis-stimulation (SM > FM).
Table 1. Control, S. enteritidis-treated, and fold change of IL-6, CXCLi2, and IFN-α mRNA expression levels in heterophils isolated from F1 cross C and D chickens separated by sexa
Organ invasion
In addition to the in vitro heterophil functional studies, an in vivo S. enteritidis challenge was conducted to determine whether there were differences in extraintestinal organ invasion between the FF and SF chickens and the FM and SM chickens (). The FF chickens had numerically (P = 0.09) fewer positive chickens compared with the S. enteritidis-infected SF (36.7% and 49.4%, respectively). However, there was a statistical (P < 0.05) difference between the males. There were 15.6% S. enteritidis-positive FM chickens, while 34.4% from the SM were positive for S. enteritidis organ invasion. All control chickens were negative for S. enteritidis. There was no linkage between the feathering gene and protection against S. enteritidis organ invasion (liver and spleen).
Figure 3. Two-day-old chickens were challenged orally with S. enteritidis (5×106 CFU/chicken) and liver/spleen organ invasion was determined using standard microbiological techniques. Data are pooled from 90 chickens and presented as the mean±standard error of the mean of triplicate organ invasion experiments. Data shown are the percentages obtained from the number of S. enteritidis-positive chickens/90. All comparisons are between females (FF vs SF) or between males (FM vs SM) (*P < 0.05).
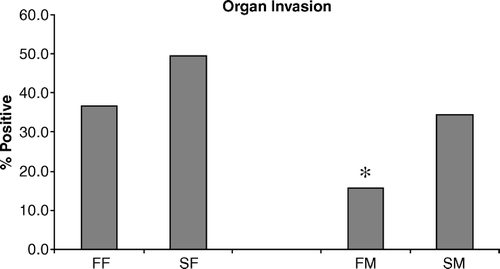
Discussion
Previous studies by our laboratory examined in vitro heterophil function and resistance/susceptibility to bacterial challenge with S. enteritidis and Enterococcus gallinarum in parental broilers (Line A [fast feathering] and Line B [slow feathering]) and their F1 reciprocal crosses (C and D) (Swaggerty et al., Citation2003b, Citation2005b; Ferro et al., Citation2004). With respect to the feathering gene, the F1 reciprocal cross males are the same in both lines (SM and FM = K/k + ) whereas the females are different (SF = K/–; FF = k + /–). The initial studies by our group, as well as all subsequent evaluations, have shown a dichotomy of innate immune responsiveness between fast and slow feathering lines at the parent level and F1 generations. In all immunologic evaluations of the lines of these chickens, fast-feathering chickens have a more effective heterophil-mediated innate immune response with increased resistance to bacterial challenges compared with their slow-feathering counterparts (Swaggerty et al., Citation2003a,Citationb, Citation2004, Citation2005a,Citationb, Citation2006; Ferro et al., Citation2004). The objective of the present study was to determine whether the feathering gene was actually linked to, not just associated with, increased in vitro heterophil function, the cytokine/chemokine mRNA expression levels produced by heterophils, or increased resistance to in vivo liver/spleen organ invasion by S. enteritidis.
To our knowledge and with the exception of our initial report (Swaggerty et al., Citation2003a), there are no studies evaluating the role of the feathering gene in either innate immune responsiveness or heterophil function in chickens. Therefore, our results showing that the bactericidal killing mechanisms of degranulation and oxidative burst were linked to the recessive k fast feathering gene were the first report to show direct linkage between the feathering gene and innate immunity in broilers. These data do not indicate that the feathering gene is the sole gene responsible for degranulation and oxidative burst as heterophils from both fast and slow feathering chickens have a response.
Slow feathering in poultry is a result of a qualitative Z-sex-linked dominant gene (K), while fast feathering is actually the wild-type trait associated with the recessive allele (k) (Moreng and Avens, Citation1985; Austic and Nesheim, Citation1990). Slow feathering is a mutation resulting from the insertion of endogenous retroviral DNA (ev21) into the genome, which then becomes inherited like any other host gene (Bacon et al., Citation1988; Iraqi & Smith, Citation1995). In addition to the feathering alleles K and k mentioned in the present study, there are two other feathering alleles, K n and K s , which are associated with varying degrees of feathering (Somes, Jr., Citation1969; Chambers et al., Citation1994). Ultimately, K n is the dominant feathering allele and the most severe, but none of the chickens utilized in the present study fall into this genotype. Therefore, for the chickens we evaluated in this study, the slow-feathering K allele was dominant. There are numerous reports relating the feathering gene to nutrition and growth (Ajang et al., Citation1993; Fotsa et al., Citation2001; Hamoen et al., Citation2001; Kalinowski et al., Citation2003; Sikur et al., Citation2004). However, most of the studies involving the immune function of fast-feathering and slow-feathering chickens are directed towards viral immunity or antibody production (Bacon et al., Citation1986; Smith & Fadly, Citation1988; Fadly & Smith, Citation1997; Smith & Crittenden, Citation1998). Slow-feathering progeny from a slow-feathering dam displayed an “inadequate immune response” based on increased viral infections and decreased antibody production indicating a poor acquired immune response (Harris et al., Citation1984). The equivalent slow-feathering progeny from slow-feathering dams in the present study were the SM and SF. Similarly, we observed a decreased immune function as demonstrated by a decreased bactericidal killing and increased susceptibility to S. enteritidis organ invasion. As we now know the acquired immune response is controlled by the innate immune response, therefore, a poor acquired response would be directly related to an initial poor innate response (Bendelac et al., Citation1997; Janeway, Jr. & Medzhitov, Citation2002; Pasare & Medzhitov, Citation2005).
The observation that phagocytosis and cytokine/chemokine mRNA expression levels were not under genetic control of the feathering gene was not surprising. These actions are very complex and are under the control of numerous genes and gene families and redundant pathways (Underhill and Ozinsky, Citation2002; Jutras and Desjardins, Citation2005; Stuart and Ezekowitz, Citation2005). In fact, IL-6 and CXCLi2 are encoded on chromosomes 2 and 4, respectively (Kaiser et al., Citation2005); therefore, it is logical that there is no linkage between the feathering gene that is on the Z chromosome. Even though IFN-α is encoded on the Z sex chromosome, the data indicate its mRNA expression levels are not linked to the feathering gene, also present on the same chromosome. The results presented herein showed S. enteritidis liver/spleen organ invasion in young chickens was not under control of the feathering gene. Numerous studies indicate S. enteritidis organ invasion is controlled by multiple genes (Bumstead & Barrow, Citation1988; Mariani et al., Citation2001; Lamont et al., Citation2002; Wigley et al., Citation2002; Wigley Citation2004; Beal et al., Citation2005). Lamont and colleagues show there are at least four genes associated with S. enteritidis colonization of the spleen in 1-day-old chickens; however, the role of the feathering gene is not addressed in that study (Lamont et al., Citation2002). Even though the feathering gene was not linked to S. enteritidis organ invasion in young broilers, our previous studies indicate there is an association between fast feathering and increased resistance to bacterial infections (Ferro et al., Citation2004; Swaggerty et al., Citation2005a,Citationb). Additional studies are required to further characterize the innate immune responsiveness of the parent lines (A and B) and the F1 reciprocal crosses (C and D) including the role of the sire in conferring resistance/susceptibility to S. enteritidis organ invasion.
Acknowledgments
The authors thank Ms Gena Lowry, Ms Lisa Rothwell, Ms Kayla Sagebiel and Dr Laura Ripley for technical assistance and/or help with animal care. Experiments were conducted according to regulations established by USDA animal care committee. Mention of commercial products is for the sole purpose of providing specific information, not recommendation/endorsement by the USDA. Experiments were funded, in part, by the US Poultry & Egg Association (MHK and CLS, project #612).
References
- Ajang , O.A. , Prijono , S. and Smith , W.K. 1993 . Effect of dietary protein content on growth and body composition of fast and slow feathering broiler chickens . British Poultry Science , 34 : 73 – 91 .
- Austic , R.E. and Nesheim , M.C. 1990 . Poultry breeding . Poultry Production , 13 ( 3 ) : 61 – 88 .
- Bacon , L.D. , Fadly , A.M. and Crittenden , L.B. 1986 . Absence of influence on immune competence by the sex-linked gene (K) determining slow feathering in white Leghorn chickens . Avian Diseases , 30 : 751 – 760 .
- Bacon , L.D. , Smith , E. and Crittenden , L.B. 1988 . Association of the slow feathering (K) and an endogenous viral (ev21) gene on the Z chromosome of chickens . Poultry Science , 67 : 191 – 197 .
- Beal , R.K. , Powers , C. , Wigley , P. , Barrow , P.A. , Kaiser , P. and Smith , A.L. 2005 . A strong antigen-specific T-cell response is associated with age and genetically dependent resistance to avian enteric Salmonellosis . Infection and Immunity , 73 : 7509 – 7516 .
- Bendelac , A. and Fearon , D.T. 1997 . Innate immunity: innate pathways that control acquired immunity . Current Opinion in Immunology , 9 : 1 – 3 .
- Bumstead , N. and Barrow , P.A. 1988 . Genetics of resistance to Salmonella tyPhimurium in newly hatched chicks . British Poultry Science , 29 : 521 – 529 .
- Chambers , J.R. , Smith , E.J. , Dunnington , E.A. and Siegel , P.B. 1994 . Sex-linked feathering (K, k + ) in chickens: A review . Poultry Science Reviews , 5 : 97 – 116 .
- Fadly , A.M. and Smith , E.J. 1997 . Role of contact and genetic transmission of endogenous virus-21 in the susceptibility of chickens to avian leukosis virus infection and tumors . Poultry Science , 76 : 968 – 973 .
- Ferro , P.J. , Swaggerty , C.L. , Kaiser , P. , Pevzner , I.Y. and Kogut , M.H. 2004 . Heterophils isolated from chickens resistant to extraintestinal Salmonella enteritidis infection express higher levels of pro-inflammatory cytokine mRNA following infection than heterophils from susceptible chickens . EPidemiology and Infection , 132 : 1029 – 1037 .
- Fotsa , J.-C. , Mérat , P. and Bordas , A. 2001 . Effect of the slow (K) or rapid (k+) feathering gene on body and feather growth and fatness according to ambient temperature in a Leghorn×brown egg type cross . Genetics Selection Evolution , 33 : 659 – 670 .
- Hamoen , F.F.A. , Van Kaam , J.B.C.H.M. , Groenen , M.A.M. , Vereijken , A.L.J. and Bovenhuis , H. 2001 . Detection of genes on the Z-chromosome affecting growth and feathering in broilers . Poultry Science , 80 : 527 – 534 .
- Harris , D.L. , Garwood , V.A. , Lowe , P.C. , Hester , P.Y. , Crittenden , L.B. and Fadly , A.M. 1984 . Influence of sex-linked feathering phenotypes of parents and progeny upon lymphoid leukosis virus infection status and egg production . Poultry Science , 63 : 401 – 413 .
- He , H. , Farnell , M.B. and Kogut , M.H. 2003 . Inflammatory agonist stimulation and signal pathway of oxidative burst in neonatal chicken heterophils . ComParative Biochemistry and Physiology , 135 : 177 – 184 .
- Iraqi , F. and Smith , E.J. 1995 . Organization of the sex-linked late-feathering haplotype in chickens . Animal Genetics , 26 : 141 – 146 .
- Janeway , C.A. Jr and Medzhitov , R. 2002 . Innate immune recognition . Annual Review of Immunology , 20 : 197 – 216 .
- Jutras , I. and Desjardins , M. 2005 . Phagocytosis: at the crossroads of innate and adaptive immunity . Annual Review of Cell and Developmental Biology , 21 : 511 – 527 .
- Kaiser , P. , Rothwell , L. , Galyov , E.E. , Barrow , P.A. , Burnside , J. and Wigley , P. 2000 . Differential cytokine expression in avian cells in response to invasion by Salmonella typhimurium, Salmonella enteritidis, and Salmonella gallinarum . Microbiology , 146 : 3217 – 3226 .
- Kaiser , P. , Yeow-Poh , T. , Rothwell , L. , Avery , S. , Balu , S. , Pathania , U.S. , Hughes , S. , Goodchild , M. , Morrell , S. , Watson , M. , Bumstead , N. , Kaufman , J. and Young , J.R. 2005 . A genomic analysis of chicken cytokines and chemokines . Journal of Interferon & Cytokine Research , 25 : 467 – 484 .
- Kalinowski , A. , Moran , E.T. Jr and Wyatt , C.L. 2003 . Methionine and cystine requirements of slow-and fast-feathering broiler males from three to six weeks of age . Poultry Science , 82 : 1428 – 1437 .
- Kogut , M.H. , Genovese , K.J. and Lowry , V.K. 2001 . Differential activation of signal transduction pathways mediating phagocytosis, oxidative burst, and degranulation by chicken heterophils in response to stimulation with opsonized Salmonella enteritidis . Inflammation , 25 : 7 – 15 .
- Kogut , M.H. , Rothwell , L. and Kaiser , P. 2003 . Differential regulation of cytokine gene expression by avian heterophils during receptor-mediated phagocytosis of opsonized and non-opsonized Salmonella enteritidis . Journal of Interferon and Cytokine Research , 23 : 319 – 327 .
- Lamont , S.J. , Kaiser , M.G. and Liu , W. 2002 . Candidate genes for resistance to Salmonella enteritidis colonization in chickens as detected in a novel genetic cross . Veterinary Immunology and Immunopathology , 87 : 423 – 428 .
- Mariani , P. , Barrow , P.A. , Cheng , H.H. , Groenen , M.A.M. , Negrini , R. and Bumstead , N. 2001 . Localization to chicken chromosome 5 of a novel locus determining salmonellosis resistance . Immunogenetics , 53 : 786 – 791 .
- Moreng , R.E. and Avens , J.S. 1985 . Genetics, breeding, and culling and selection . Poultry Science & Production , 4 : 85 – 114 .
- National Research Council . ( 1994 ). Nutrient Requirements of Poultry 9th edn (pp. 19 – 34 ). Washington , DC : National Academy Press .
- Pasare , C. and Medzhitov , R. 2005 . Toll-like receptors: linking innate and adaptive immunity . Advances in Experimental Medicine and Biology , 560 : 11 – 18 .
- Sikur , V.R. , Robinson , F.E. , Korver , D.R. , Renema , R.A. and Zuidhof , M.J. 2004 . Effects of nutrient density on growth and carcass traits in fast- and slow-feathering female turkeys . Poultry Science , 83 : 1507 – 1517 .
- Smith , E.J. and Crittenden , L.B. 1998 . Genetic cellular resistance to subgroup E avian leukosis virus in slow-feathering dams reduces congenital transmission of an endogenous retrovirus encoded at locus ev 21 . Poultry Science , 67 : 1668 – 1673 .
- Smith , E.J. and Fadly , A.M. 1988 . Influence of congenital transmission of endogenous virus-21 on the immune response to avian leukosis virus infection and the incidence of tumors in chickens . Poultry Science , 67 : 1674 – 1679 .
- Somes , R.G.Jr. 1969 . Delayed feathering, a third allele at the K locus of the domestic fowl . Journal of Heredity , 60 : 281 – 286 .
- Stuart , L.M. and Ezekowitz , R.A. 2005 . Phagocytosis: elegant complexity . Immunity , 22 : 539 – 550 .
- Swaggerty , C.L. , Pevzner , I.Y. , Ferro , P.J. , Crippen , T.L. and Kogut , M.H. 2003a . Association between in vitro heterophil function and the feathering gene in commercial broiler chickens . Avian Pathology , 32 : 483 – 488 .
- Swaggerty , C.L. , Pevzner , I.Y. , Lowry , V.K. , Farnell , M.B. and Kogut , M.H. 2003b . Functional comparison of heterophils isolated from commercial broiler chickens . Avian Pathology , 32 : 95 – 102 .
- Swaggerty , C.L. , Kogut , M.H. , Ferro , P.J. , Rothwell , L. , Pevzner , I.Y. and Kaiser , P. 2004 . Differential cytokine mRNA expression in heterophils isolated from Salmonella-resistant and -susceptible chickens . Immunology , 113 : 139 – 148 .
- Swaggerty , C.L. , Ferro , P.J. , Pevzner , I.Y. and Kogut , M.H. 2005a . Heterophils are associated with resistance to systemic Salmonella enteritidis infection in genetically distinct lines of chickens . FEMS Immunology and Medical Microbiology , 43 : 149 – 154 .
- Swaggerty , C.L. , Lowry , V.K. , Ferro , P.J. , Pevzner , I.Y. and Kogut , M.H. 2005b . Disparity in susceptibility to vancomycin-resistant Enterococcus organ invasion in commercial broiler chickens that differ in innate immune responsiveness . Food and Agricultural Immunology , 16 : 1 – 15 .
- Swaggerty , C.L. , Kaiser , P. , Rothwell , L. , Pevzner , I.Y. and Kogut , M.H. 2006 . Heterophil cytokine mRNA profiles from genetically distinct lines of chickens with differential heterophil-mediated innate immune responses . Avian Pathology , 35 : 102 – 108 .
- Underhill , D.M. and Ozinsky , A. 2002 . Phagocytosis of microbes: complexity in action . Annual Review of Immunology , 20 : 825 – 852 .
- Wigley , P. 2004 . Genetic resistance to Salmonella infection in domestic animals . Research in Veterinary Science , 76 : 165 – 169 .
- Wigley , P. , Hulme , S.D. , Bumstead , N. and Barrow , P.A. 2002 . In vivo and in vitro studies of genetic resistance to systemic salmonellosis in the chicken encoded by the SAL1 locus . Microbes and Infection , 4 : 1111 – 1120 .