Abstract
Riemerella anatipestifer causes infectious serositis of ducks and geese. The genomic diversity of R. anatipestifer associated with outbreaks in waterfowls was studied using 24 multidrug-resistant R. anatipestifer isolates collected from the visceral organs of ducks and geese from seven outbreaks in four goose farms and one outbreak in one duck farm. Seven methods were used to differentiate these isolates. Plasmid patterns differed in plasmid number and size, ranging from 2.9 kb to 20 kb, and provided seven profiles. Divergent nucleotide sequences (predominant in 670 to 830 base pairs) of the ompA gene categorized the 24 isolates into three groups based on cluster analysis and polymerase chain reaction–restriction fragment length polymorphism. Repetitive-sequence polymerase chain reaction and pulsed-field gel electrophoresis analysis revealed the highest genotypic variations among the isolates. Genotypes and serotypes differed among farms and within the same farm and even within a single goose. In conclusion, a difference in R. anatipestifer genotypes and serotypes was observed for multiple outbreaks in waterfowls.
Diversité génomique et différenciation moléculaire de Riemerella anatipestifer associé à huit cas dans cinq élevages.
Riemerella anatipestifer entraîne une sérosite infectieuse chez le canard et l'oie. La diversité génomique de R. anatipestifer associée à des cas chez les palmipèdes a été étudiée en utilisant vingt-quatre souches multirésistantes de R. anatipestifer isolées à partir d'organes viscéraux de canards et d'oies provenant de sept cas dans quatre élevages d'oie et d'un cas dans un élevage de canard. Sept méthodes ont été utilisées pour différencier ces isolats. Les profils des plasmides ont été différents en ce qui concerne le nombre des plasmides et la taille allant de 2,9 kb à 20 kb et conduisant à sept profils. Les séquences nucléotidiques divergentes du gène ompA (en particulier entre 670–830 pb) ont permis de classer les 24 isolats en trois groupes basés sur l'analyse de groupe et le polymorphisme de taille des fragments de PCR. La Rep-PCR et l'analyse électrophorétique en champ pulsé ont révélé les variations génotypiques les plus élevées parmi les isolats. Les génotypes et les sérotypes ont été différents selon les élevages et pour un même élevage et voire une même oie. En conclusion, les différences de génotype et de sérotype de R. anatipestifer ont été observées dans des cas multiples chez les palmipèdes.
Genomvielfalt und molekulare Differenzierung von Riemerella anatipestifer-Isolaten aus acht Krankheitsausbrüchen in fünf Farmen
Riemerella anatipestifer ist der Erreger der infektiösen Serositis bei Enten und Gänsen. Die Genomvielfalt von R. anatipestifer im Zusammenhang mit Erkrankungen von Wassergeflügel wurde anhand von 24 R. anatipestifer-Isolaten mit hochgradiger Antibiotikaresistenz, die in viszeralen Organen von Enten und Gänsen bei sieben Ausbrüchen in vier Gänsefarmen und bei einem Ausbruch in einer Entenfarm nachgewiesen worden waren, untersucht. Zur Differenzierung dieser Isolate wurden sieben Methoden angewendet. Die Plasmidmuster unterschieden sich in Anzahl und Größe (2,9-20kb) and ließen sieben verschiedenen Profile erkennen. Basierend auf Clusteranalyse und PCR-Restriktionsfragmentlängen-polymorphismus führten divergierende Nukleotidsequenzen (hauptsächlich in der Region 670–830 bp) des ompA-Gens zur Einteilung der Isolate in drei Gruppen. Durch repetitive Sequenz-PCR und Pulsfeld-Gelelektrophorese wurden die größten genotypischen Unterschiede zwischen den Isolaten deutlich. Genotypen und Serovare unterschieden sich zwischen den Farmen, innerhalb derselben Farm und sogar innerhalb einer einzelnen Gans. Zusammenfassend kann festgestellt werden, dass Unterschiede bei den R. anatipestifer-Genotypen und Serovaren bei vielen Krankheitsausbrüchen beim Wassergeflügel vorkommen.
Diversidad genómica y estudio molecular de Riemerella anatipestifer associada con ocho brotes en cinco granjas.
Riemerella anatipestifer causa poliserositis infecciosa en patos y gansos. Se estudió la diversidad genética de R. anatipestifer asociada a brotes en aves acuáticas mediante el uso de veinticuatro aislados de R. anatipestifer resistentes a multiples antibióticos obtenidos de órganos viscerales de patos y gansos de siete brotes clínicos en cuatro granjas de gansos y un brote clínico en una granja de patos. Se utilizaron siete métodos distintos para diferenciar estos aislados. Los patrones de plásmidos variaron en el número de plásmidos y su tamaño entre 2.9 kb y 20 kb y mostraron siete perfiles. Las divergencias nucleotídicas de las secuencias (mayoritariamente entre 670 y 830 bp) del gen ompA clasificaron los 24 aislados en tres grupos según el análisis de grupos y el polimorfismo de la longitud de los fragmentos de restricción. Los estudios de PCR de secuencias repetitivas y de electroforesis en gel de campo pulsado mostraron las mayores variaciones genotípicas entre los aislados. Los genotipos y serotipos variaron entre las granjas y dentro de una misma granja, incluso en un mismo ganso. En conclusión, se observaron diferencias en los genotipos y serotipos de R. anatipestifer de diversos brotes clínicos en aves acuáticas.
Introduction
Riemerella anatipestifer is a non-motile Gram-negative and non-spore-forming rod that causes septicaemia and infectious serositis in waterfowls (Hendrickson & Hilbert, Citation1932; Graham et al., Citation1938; Dougherty et al., Citation1955; Harry, Citation1969). Adverse climate or concomitant disease often predispose the birds to outbreaks of R. anatipestifer infection. For ducklings infected by R. anatipestifer before 5 weeks old, mortality varies from 5% to 75% and morbidity is usually as high as 100%. Older waterfowls usually suffer chronic, non-fatal or subclinical disease (Sandhu, Citation2003). It is therefore difficult to exclude R. anatipestifer infection from waterfowls in a farm rearing multi-age birds. Due to the divergence, 21 serotypes of R. antipestifer have been reported in different countries (Harry, Citation1969; Sandhu & Harry, Citation1981; Loh et al., Citation1992; Subramaniam et al., Citation1997; Pathanasophon et al., Citation2002; Tsai et al., Citation2005). Such divergence contributes to low cross-protection against different R. anatipestifer strains and variations in virulence factors (Sandhu & Harry, Citation1981; Weng et al., Citation1999; Subramaniam et al., Citation2000; Huang et al., Citation2002), resulting in mixed infection of more than one serotype of R. anatipestifer in the same duck and frequent change of serotypes in the same farm (Teo et al., Citation1992). However, understanding the association of genomic variations of R. anatipestifer with the outbreaks in waterfowls in different farms is lacking.
Traditionally, serotyping has been used to discriminate the infectious R. anatipestifer isolates. Molecular methods such as repetitive-sequence polymerase chain reaction (Rep-PCR) have also been used for further differentiation of these serotypes (Huang et al., Citation1999). Recently, a gene ompA encoding for a 42-kDa outer membrane protein (OmpA) that consists of six EF-hand Ca2 + -binding domains (a structure of helix–calcium-binding loop–helix) and two proline (P), glutamic acid (E), erine (S), and threonine (T)-rich (PEST) regions that differs from other Gram-negative bacteria has been shown in R. anatipestifer (Subramaniam et al., Citation2000; Huang et al., Citation2002). The ompA gene may be a potential target for identification and differentiation of R. anatipestifer. Although extensive studies in pathogenesis (Crasta et al., Citation2002) and vaccine development (Higgins et al., Citation2000) play a role in reducing R. anatipestifer infection, the genotypic variations have not been studied in detail. The present study was therefore conducted to determine the genomic diversity of multidrug-resistant R. anatipestifer associated with outbreaks in waterfowls using plasmid profiling, pulsed-field gel electrophoresis (PFGE), Rep-PCR and PCR-restriction fragment length polymorphism (PCR-RFLP).
Materials and Methods
Bacterial strains and media
Various tissues, including the liver, blood, brain, trachea, bone marrow and joint, of 41 sick birds (two Pekin ducks and 39 Roman geese) were sampled from five different farms in Chiayi, Yu-Lin, Tainan and Nanto counties in central and south Taiwan. Twenty-four R. anatipestifer isolates were identified by biochemical reactions using commercial API 20NE and API ZYM galleries as described by the manufacturer (API System; La Balme Les Grottes, France) (). R. anatipestifer were grown on blood agar or in brain heart infusion broth (DIFCO) at 5% CO2 and 37°C for 24 h for further use.
Table 1. Characterization of R. anatipestifer isolates by seven different methods
Antibiotic susceptibility test
The antibiotic susceptibility test was performed by disc diffusion methods according to the manufacturer's recommendation (Oxoid), and Escherichia coli strains ATCC 25922 was used for validating the results of antibiotic susceptibility test (NCCLS, Citation2003). Sixteen tested antibiotics categorized into four different inhibitory functional groups were used, including: tetracycline (30 µg), oxytetracycline (30 µg), doxycycline (30 µg), erythromycin (15 µg), gentamicin (10 µg), and chloramphenicol (30 µg), which inhibit protein synthesis by binding of ribosome; penicillin (10 µg), amoxicillin (30 µg), ampicillin (10 µg), cephalothin (30 µg), and bacitracin (10 µg), whichthat inhibit the cell wall synthesis; novobiocin (30 µg), flumequine (30 µg), enrofloxacin (5 µg), and trimethoprime (5 µg), which prevent DNA synthesis; and colistin (10 µg), which interrupts cell membrane. Resistance to antibiotics was determined according to the interpretation criteria for E. coli established by NCCLS (Citation2003).
Sequence analysis of the ompA gene
PCR amplification of the ompA gene
The forward primer, OmpA-FF (5′-atg ttg atg act gga ctt ggt ct-3′), and reverse primer, OmpA-RR (5′-ctt cac tac tgg aag gtc aga ctt-3′) were designed to amplify a 1119-base pair (bp) PCR product of ompA according to published ompA nucleotide sequences (GenBank accession number AF104936) of R. anatipesifer (Huang et al., Citation2002). Colonies were collected from blood agar and suspended in 500 µl distilled water and boiled at 100°C. After centrifugation at 17,000 x g for 10 min the supernatant was used as the DNA source for PCR. The PCR mixture consisted of 0.25 mM dNTPs, 0.5 µM each forward and reverse primer, 1 µl DNA template and 1 U Taq DNA polymerase (Promega). PCR was performed in a Mastercycler thermocycler (Eppendorf) with running condition of denaturation at 94°C for 2 min, 35 cycles of denaturation at 94°C for 1 min, annealing at 54°C for 1 min, and extension at 72°C for 1 min, and final extension at 72°C for 3 min. The PCR products were separated by a 2% agarose gel at 50 V for 1 h and were visualized after staining with ethidium bromide.
Cluster analysis of amplified ompA gene sequences
After purification by the PCR clean-up kit (Promega), the PCR products were sequenced by the ABI autosequencer 3730 (Applied Biosystems). Sequence assembly of a 1119-bp PCR product and construction of a dendrogram of 24 isolates were performed respectively by Lasergene v7.1 with programs SeqMan and MegAlign using the ClustalV method (gap penalty = 8, Ggap length penalty = 3) (DNASTAR). Nucleotide sequences of five representative isolates of each genotype were deposited under accession numbers EU258702, EU258703, EU258704, EU258705 and EU258706 for isolates 10, 807D, 27A, 730C and 35A, respectively.
Differentiation of R. anatipestifer isolates
PCR-RFLP analysis of amplified ompA gene sequences
The PCR product from each isolate was digested with restriction endonuclease AluI for 3 h. The DNA fragments were separated by a 5% polyacrylamide gel electrophoresis at 80 V for 1.5 h.
Genotyping by Rep-PCR analysis
The primers (REP-1and REP-2) and PCR conditions as described by Huang et al. (Citation1999) were used to differentiate R. anatipestifer isolates. Multiple PCR products were separated by a 0.8% agarose gel at 50 V for 2 h. Staining was performed by ethidium bromide.
Genotyping by PFGE analysis
R. anatipestifer were incubated in brain heart infusion broth at 37°C and 5% CO2 for 24 h. One millilitre of R. anatipestifer was centrifuged at 17,000 x g for 3 min. The pellet was resuspended in 500 µl distilled water and then 500 µl of 1.6% agarose gel was added to make plugs, which were treated with digestion buffer (0.5 M ethylenediamine (EDTA) tetraacetic acid, pH 8.0; 1% lauroyl sarcosine, sodium salt; Sigma) and 1 mg/ml proteinase K (Roche Diagnostics) at 50°C overnight. After washing once with distilled water at 50°C, plugs were washed with 10 mM TE buffer (10mM Tris_HCl, 1mM EDTA, pH 7.0) at 50°C for 30 min. This process was repeated five times. Plugs were then treated with restriction endonuclease BamHI, EcoRI, HindIII or SmaI at 37°C for 4 h and separated by the CHEF-DR III system (BioRad) in 1% agarose gel with 0.5 x TBE running buffer at 14°C under conditions of 3.5 V/cm, 110° separation angle, and switching time of 4∼ to 70 sec for 20 h followed by 4 h with the same conditions but a change of voltages to 4 V/cm. The dendrogram was constructed by the unweighted pair group method with the arithmetic average (UPGMA) by Bio-Gene v11.9 (Vilber Lourmat, France) using the Dice similarity coefficient and a 3% tolerance for the banding migration distance.
Plasmid analysis
Each R. anatipestifer isolate was incubated in brain heart infusion broth at 37°C and 5% CO2 for 24 h. The pellet was collected by centrifugation at 10,000 x g for 5 min and was used to analyse the plasmid profile of each R. anatipestifer isolate by a modified Kado–Liu method (Kado & Liu, Citation1981). The lysis buffer was changed to 1.5% sodium dodecyl sulphate and 0.2 N NaOH without heating compared with the original procedure.
Serotyping
Nine R. anatipestifer isolates were randomly selected for serotyping, which was performed and analysed by the Animal Health Research Institute, Taiwan, using the antibody and antigen agglutination method.
Results
R. antipestifer isolation and antibiotic susceptibility
Twenty-four R. anatipstifer isolates were obtained from various tissues, including the heart, blood, bone marrow, brain, liver, lung, trachea, and joint, from 16 diseased Roman geese and one Pekin duck (). Analysis of seven outbreaks in geese revealed that recurrent outbreaks only occurred in Farm A. All isolates were resistant to between five and 13 antimicrobials. The most antibiotic resistant isolates were found in Farms B and C (). The frequency of drug resistance differed among antibiotics, and was 17% for enrofloxacin, 73% for novobiocin, 81% for flumequine, 87% for trimethoprime, 42% for amoxicillin, 50% for bacitracin, 54% for ampicillin, 58% for penicillin, 65% for cephalothin, 35% for doxycycline, 58% for oxytetracycline, 64% for erythromycin, 69% for tetracycline, 73% for gentamicin, 77% for chloramphenicol and 73% for colistin.
Use of the ompA gene sequence to differentiate R. anatipestifer
Cluster analysis of the 1119-bp ompA PCR products separated the R. anatipestifer isolates into two classes, and most of them belonged to genotype I (). Sequence analysis of the representative strains of each genotype showed that the sequence variations occurred mainly in the region of 670 to 830 bp, which encodes two PEST regions (Subramaniam et al., Citation2000). The nucleotide sequences of ompA of isolate 35C differed from those of other strains mainly due to a 8-bp (ATGGTGTA) insertion at the location of 600 bp to form a pseudogene ().
Figure 1. Cluster analysis of 24 R. anatipestifer isolates constructed using ClustalV to analyse nucleotide sequences of ompA. Bootstrap values were estimated for this tree using the neighbour-joining model for 1000 replicates. Arabic number, bootstrap value of each cluster.
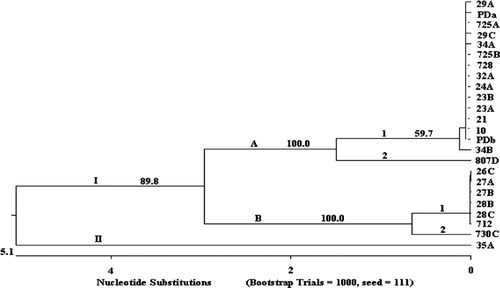
Figure 2. Nucleotide sequence alignment and comparison of the ompA of five representative R. anatipestifer isolates. Underlined region, restriction site of restriction enzyme AluI. Arrow, direct repeat region. Repeats II and III encode for PEST regions (Subramaniam et al., Citation2000), and two are imperfect repeats with a sequence diverse from repeats II and III. –, deletion.
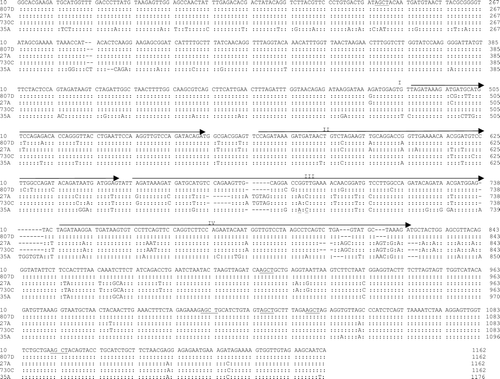
Genotyping of R. anatipestifer by Rep-PCR and PFGE analysis
After Rep-PCR amplification, between five and 15 DNA fragments ranging from 0.2 to 4 kb in size separated the isolates into four genotypes based on the difference of more than five DNA fragments ( and ). Similar to the results of the ompA analysis, 18 R. anatipestifer isolates belonged to genotype I, which was classified into two subtypes defined by a difference in more than two bands (). PFGE analysis was the best method to discriminate genome variations of R. anatipestifer isolates (). Size patterns of restriction DNA fragments were associated with restriction enzymes used. The size ranged from 20 kb to 1 Mb for SmaI-digested DNAs, from 20 kb to 350 kb for BamHI-digested DNAs fragments, and almost all were less than 40 kb for EcoRI-produced and HindIII-produced DNA fragments. Unlike the other enzymes, SmaI-digested DNA fragments distributed broadly and evenly, and were used to separate 19 isolates into seven genotypes with most isolates belonging to genotypes I and IV ().
Figure 3. Rep-PCR patterns of 24 R. anatipestifer isolates. M, 1 kb size marker ranging from 200 bp to 14 kb. N, Negative control (PCR mixture without DNA template).
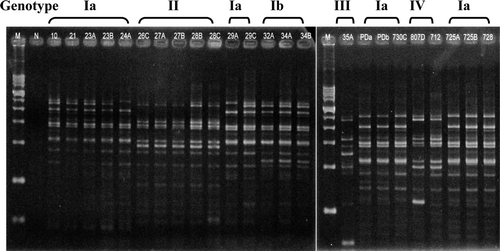
Figure 4. PFGE patterns of R. anatipestifer isolates. Genomic DNAs were digested by restriction endonuclease SmaI. M, DNA size marker (48.5-kb phage ladder; BIO-RAD, USA).The same Arabic number means R. anatipestifer isolates were isolated from the same goose or duck. Different letters indicate R. anatipestifer isolates were isolated from different tissues.
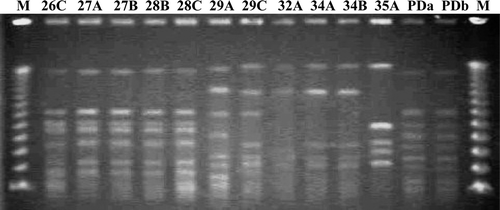
Figure 5. Dendrogram of 24 R. anatipestifer isolates constructed by UPGMA with 3% tolerance to analyse SmaI-digested PFGE pattern. The same Arabic number means R. anatipestifer isolates were isolated from the same goose or duck. Different letters indicate R. anatipestifer isolates were isolated from different tissues.
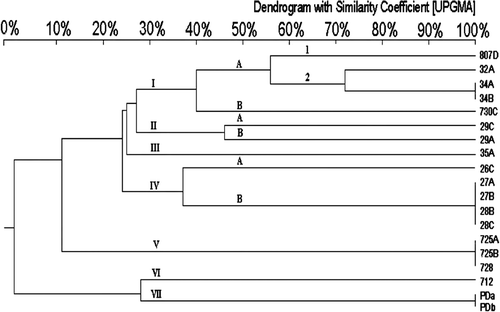
Plasmid profile
Except for six isolates, all R. anatipestifer isolates harboured at least one plasmid with a size ranging from 2.9 to 20 kb. The most common plasmid sizes were 4 kb and 20 kb. Based on the plasmid size and number, these isolates were classified into seven groups (). No association was observed between plasmid pattern and antibiotic resistance pattern.
Serotyping
Nine R. anatipestifer isolates were tested and four serotypes (Serotypes 2, 4, 5, and 6) were observed. Serotypes 2 and 6 were the most predominant serotypes (). Diverse serotypes were found in Farm B.
Discussion
Highly diverse genotypes of R. anatipestifer isolates with different PFGE and plasmid patterns were observed. Infection with two highly multidrug-resistant R. anatipestifer isolates belonging to different serotypes in one goose was compatible with a previous report of multiple infections of different serotypes of R. anatipestifer in the same duck (Teo et al., Citation1992). Changes of genomic pattern and plasmid profile in R. anatipestifer isolates, such as recurrent outbreaks in Farm A, suggest that diverse origins of R. anatipestifer may be responsible for the extent or severity of serotype, infectivity, and drug resistance of R. anatipestifer.
The insufficient bio-security and inadequate husbandry of the goose farms increase the exposure of geese to R. anatipestifer. In addition, R. anatipestifer infection often causes subclinical disease in old waterfowls (Sandhu, Citation2003). Further, improper antibiotic use may increase development of multidrug-resistant isolates. All the above factors may have contributed to the highest multidrug-resistant R. anatipestifer isolates in Farms C and E, gradually to increase antibiotic resistance in the subsequent outbreaks in Farm A. Although antibiotic resistance was not associated with plasmid pattern (), the diverse plasmids may carry additional antibiotic resistance or virulence genes, other than virulence-associated genes vapD1 and vapD2 (Weng et al., Citation1999). Although PFGE analysis demonstrated the highest genomic variations among the isolates, plasmid profiling was the easiest approach to categorize R. anatipestifer isolates.
R. anatipestifer is a highly invasive pathogen, causes septicaemia and polyseositis, and is quickly disseminated to various tissues in infected geese and ducks of different ages. Although infection with Ornithobacterium rhinotracheale or Pasteurella multocida is often involved in septicemia and polyseositis, O. rhinotracheale may cause severe necrotizing suppurative tracheitis and pneumonia (Chin et al., Citation2003) and P. multocida may present severe necrotizing suppurative dermocellulitis along the face and neck (Glisson et al., Citation2003). To differentiate R. anatipestifer from other bacterial species, R. anatipestifers-specific ompA that differs from that of the other Gram-negative bacteria has been proposed as the identification target (Subramaniam et al., Citation1997, Citation2000). Sequence analysis of ompA showed two direct repeats (II and III) responsible for two PEST regions (Subramaniam et al., Citation2000) and two imperfect direct repeats flanking these two regions (). Despite early reports of high divergence in nucleotide and amino acid sequences of the ompA gene (Tsai et al., Citation2005), major sequence variations in the present investigation were located in direct repeat III, the region responsible for divergent genotypes and possibly for formation of separate epitopes to limit OmpA as the vaccine target (Huang et al., Citation2002). Although there seems to be a relation between the R. anatipestifer ompA genotype and serotype, the role that OmpA plays in determining the R. anatipestifer serotypes needs further study.
Acknowledgements
This work was funded by grants from Council of Agriculture under Grants (1)94AS-13.1.2-BQ-B1, (1)95AS-13.1.2-BQ-B1, 95AS-13.2.1-BQ-B8(2), and 96 AS-14.6.1-BQ-B4(92), Executive Yuan, Taiwan.
References
- Chin , R.P. , van Empel , P.C.M. and Hafez , H.M.T.S. 2003 . “ Riemerella anatipestifer infection ” . In Diseases of Poultry , 11th edn , Edited by: Saif , Y.M. , Barner , H.J. , Glisson , J.R. , Fadly , A.M. , McDougald , L.R. and Swayne , D.E. 683 – 690 . Ames : Iowa State University Press .
- Crasta , K.C. , Chua , K.L. , Subramaniam , S. , Frey , J. , Loh , H. and Tan , H.M. 2002 . Identification and characterization of CAMP cohemolysin as a potential virulence factor of Riemerella anatipestifer . Journal of Bacteriology , 184 : 1932 – 1939 .
- Dougherty , E. , Saunders , L.E. and Parson , E.H. 1955 . The pathology of infectious serositis of ducks . American Journal of Pathology , 31 : 475 – 487 .
- Gilsson , J.R. , Hofacre , C.L. and Christensen , J.P. 2003 . “ Riemerella anatipestifer infection ” . In Diseases of Poultry , 11th edn , Edited by: Saif , Y.M. , Barner , H.J. , Glisson , J.R. , Fadly , A.M. , McDougald , L.R. and Swayne , D.E. 658 – 675 . Ames : Iowa State University Press .
- Graham , R. , Brandly , C.A. and Dunlap , G.L. 1938 . Studies on duck septicemia . Cornell Veterinarian , 28 : 1 – 8 .
- Harry , E.G. 1969 . Pasteurella anatipestifer serotypes isolated from cases of anatipestifer septicemia in duck . Veterinary Research , 84 : 673
- Hendrickson , J.M. and Hilbert , K.F. 1932 . A new and serious septicemia disease of young ducks with a description of the new causative organism. Pfeifferella anatipestifer . Cornell Veterinarian , 22 : 239 – 252 .
- Higgins , D.A. , Henry , R.R. and Kounev , Z.V. 2000 . Duck immune responses to Riemerella anatipestifer vaccines . Development and Comparative Immunology , 24 : 153 – 167 .
- Huang , B. , Subramaniam , S. , Chua , K.L. , Kwang , J.L. , Frey , H. and Tan , H.M. 1999 . Molecular fingerprinting of Riemerella anatipestife by repetitive sequence PCR . Veterinary Microbiology , 67 : 213 – 219 .
- Huang , B. , Subramaniam , S. , Frey , J. , Loh , H. , Tan , H.M. , Fernandez , C.J. , Kwang , J. and Chua , K.L. 2002 . Vaccination of ducks with recombinant outer membrane protein (OmpA) and a 41 kDa partial protein (P45N') of Riemerella anatipestifer . Veterinary Microbiology , 84 : 219 – 230 .
- Kado , C.I. and Liu , S.T. 1981 . Rapid procedure for detection and isolation of large and small plasmids . Journal of Bacteriology , 145 : 1365 – 1363 .
- Loh , H. , Teo , T.P. and Tan , H.C. 1992 . Serotype of “Pastaurella” anatipestifer isolate from ducks in Singapore: a proposal of new serotype . Avian Pathology , 21 : 453 – 459 .
- NCCLS 2003 . Performance Standards for Antimicrobial Disk Susceptibility Tests . Approved standard M2-A8, 8th edn . Wayne , PA : NCCLS .
- Pathanasophon , P. , Phuektes , P. , Tanticharoenyos , T. , Narongsak , W. and Sawada , T. 2002 . A potential new serotype of Riemerella anatipestifer isolated from ducks in Thailand . Avian Pathology , 31 : 267 – 270 .
- Sandhu , T.S. 2003 . “ Riemerella anatipestifer infection ” . In Diseases of Poultry , 11th edn , Edited by: Saif , Y.M. , Barner , H.J. , Glisson , J.R. , Fadly , A.M. , McDougald , L.R. and Swayne , D.E. 676 – 682 . Ames : Iowa State University Press .
- Sandhu , T.S. and Harry , E.G. 1981 . Serotypes of Pasteurella anatipestifer isolated from commercial white Pekin ducks in the United States . Avian Disease , 25 : 497 – 502 .
- Subramaniam , S.K.L. , Chua , H.M. , Tan , H. , Loh , H. , Kuhnert , P. and Frey , J. 1997 . Phylogenetic position of Riemerella anatipestifer based on 16S rRNA gene sequences . International Journal of Systematic Bacteriology , 47 : 562 – 565 .
- Subramaniam , S. , Huang , B. , Loh , H. , Kwang , J. , Tan , H.M , Chua , K.L. and Frey , J. 2000 . Characterization of a predominant immunogenic outer membrane protein of Riemerella anatipestifer . Clinical and Diagnostic Laboratory Immunology , 7 : 168 – 174 .
- Teo , T.P. , Tan , H.C. and Loh , H. 1992 . Protective efficacy of bivalent Pasteurella anatipestifer broth-grown bacteria in ducklings . Singapore Journal of Primary Industries , 20 : 53 – 60 .
- Tsai , H.J. , Liu , Y.T. , Tseng , C.S. and Pan , M.J. 2005 . Genetic variation of the ompA and 16S rRNA genes of Riemerella anatipestifer . Avian Pathology , 34 : 55 – 64 .
- Weng , S. , Lin , W. , Chang , Y. and Chang , C. 1999 . Identification of a virulence-associated protein homolog gene and ISRa1 in a plasmid of Riemerella anatipestifer . FEMS Microbiology Letters , 179 : 11 – 19 .