Abstract
Tibial dyschondroplasia (TD) is characterized by expansion of the proximal growth plates of the tibiotarsus that fail to form bone, lack blood vessels, and contain non-viable cells. Thiram (a carbamate pesticide), when fed to young broiler chicks, induces TD with high regularity and precision. We used this experimental model to understand the cause of the defects associated with TD by selecting and identifying the genes differentially expressed in the TD growth plate of broiler chickens. Broiler chicks at 7 days of age were randomly divided into two groups. After fasting overnight, they were fed with regular diet (control) or the same diet containing 100 mg/kg thiram for 96 h to induce TD (thiram-fed). mRNA was purified from the growth plates of control and thiram-fed broilers. Forward and reverse-subtracted cDNA libraries were generated by suppression subtractive hybridization technology. Ten selected genes from cDNA libraries were identified by real-time quantitative polymerase chain reaction. All were differentially expressed in TD growth plates (P<0.05 or P<0.01). The levels of collagen type X (Col X), pro-alpha-1 collagen type I (Col I α1), collagen type IX (Col IX), NADH dehydrogenase (NADH DH), cytochrome C oxidase subunit III (COX III), enolase 1, alpha (ENO1), carbonic anhydrase II (CA2) and heat shock protein 90 (Hsp90) mRNA transcripts were up-regulated, while the expression levels of Matrilin 3 (MATN3) and chondromodulin-I (ChM-I) were down-regulated. Col I and Hsp90 were detected by immunohistochemistry at different stages. Given that these genes are involved in matrix formation, endochondral ossification, developmental regulation, electron transport in the mitochondrial respiratory chain and vascularization, our findings may provide new insights into understanding the pathogenesis of TD.
Introduction
Tibial dyschondroplasia (TD) is the most prevalent skeletal abnormality in broiler chickens and turkeys. TD disorder is characterized by the formation of non-calcified, nonvascularized cartilage that can extend from the epiphyseal growth plate into the metaphysis (Whitehead, Citation1997). The economic cost of TD, as manifested in the high rates of morbidity, mortality, and condemnations at the processing plants, is enormous, as up to 30% of the flock can be affected. Various dietary, environmental, nutritional and genetic factors are involved in the aetiology of TD. The mycotoxin fusachromanone and the dithiocarbamates thiram and disulfuram can experimentally induce severe TD lesions (Rath et al., Citation2005).
Previous studies have reported abnormal gene expression in lesioned growth plate cartilage from late stages of TD, based on immunohistochemistry, semi-quantitative real-time reverse transcriptase – polymerase chain reaction (PCR) and in situ hybridization (Praul et al., Citation2000; Shirley et al., Citation2003; Webster et al., Citation2003). Genes encoding extracellular matrix molecules such as aggrecan, type II collagen, type X collagen, osteopontin and osteonectin, growth factors and hormones such as transforming growth factor-beta, PTH-related peptide and Indian Hedgehog genes, and enzymes such as carbonic anhydrase II (CA2) and matrix metalloproteinase were among those abnormally expressed (Chen et al., Citation1993; Knopov et al., Citation1995; Twal et al., Citation1996; Wu et al., Citation1996; Rath et al., Citation1997; Pines et al., Citation1998; Ben-Bassat et al., Citation1999; Webster et al., Citation2003). However, little is known about changes in expression of other genes in the early stages of TD. Identifying early alterations in gene expression may be more significant in understanding the pathogenesis of TD.
To explore the potential genes involved early in the development of TD, two cDNA libraries from control and thiram-induced early TD growth plates of broilers on the fourth day were established with suppression subtractive hybridization (SSH).
Materials and Methods
Induction of TD and tissue collection
AV broilers at 7 days of age were divided randomly into two groups. After fasting overnight, they were fed with a regular diet (control group) or a diet containing 100 mg/kg tetramethylthiuram disulphide (thiram) for 96 h (thiram-fed group) to induce TD (). After 96 h of tetramethylthiuram disulfide administration, eight birds from each group were sacrificed by cervical dislocation. The cartilage growth plates from individual birds were dissected out, immediately frozen in liquid N2, and then stored at –70°C.
RNA extraction and mRNA isolation
Individual cartilage growth plates collected from each group of birds were homogenized in TRIzol reagent (Invitrogen, Carlsbad, California, USA) to extract total RNA, according to the manufacturer's instructions. Poly(A) RNA was purified from the total RNA using the Oligotex mRNA Mini Kit (Qiagen, Germany) and the manufacturer's protocol. Concentrations were determined based on absorbance at 260 nm wavelengths.
Construction of cDNA libraries by SSH
The growth plate cartilage mRNA (2 µg) pooled from control or thiram-fed group of chickens (n=8 per group) was reverse-transcribed into cDNA. Two subtractive cDNA libraries were constructed by SSH using the PCR-Select cDNA Subtraction Kit (Clontech, Palo Alto, California, USA), according to the manufacturer's instructions. The forward library was screened for the up-regulated genes while the reverse library was examined for down-regulated genes. Secondary (nested) PCR products obtained from the forward and reverse subtractions were separately cloned into the pMD18-T vector (TaKaRa Biotech, Dalian, China), and were transformed into Escherichia coli DH5α to establish different cDNA libraries. Individual recombinant clones were cultured in 96-well plates containing 200 µl Luria–Bertani medium with ampicillin and 15% glycerol, and were then stored at –70°C.
Screening of cDNA libraries by dot blot assay
Subtracted clones were subjected to screening for the differential expression of the unique genes (Zhang et al., Citation2008). Individual PCR products were firstly denatured with 0.3 M NaOH, spotted onto nylon membranes (Hybond) in duplicate and fixed by baking in an oven at 80°C for 2 h. DIG-labelled cDNA probes from either the forward or reverse subtractions were prepared, using the DIG High Prime DNA Labeling and Detection Starter Kit I (Roche Applied Science, Mannheim, Germany), according to the manufacturer's protocols. The duplicate membranes were hybridized with two probes generated from the forward or reverse subtractions, at 65°C for 16 h. The clones that hybridized to the tester probe alone or had five-fold greater signal intensity over the control as determined by densitometry were considered positive, and were then selected for further analysis. The images were quantified by densitometry scanning, followed by analysis with GeneSnap (Syngene, USA).
DNA sequencing and gene identification
Plasmids from positive clones were extracted and their inserts were sequenced using the primer M13-47 (5′-CGCCAGGGTTTTCCCAGTCACGAC-3′) in an ABI 3700 automatic sequencer (Jenery Biotechnology, Shanghai, China). The cDNA sequences obtained were analysed by comparing them with the GenBank database and the chicken genome database using the BLASTn and BlastX programs.
Analysis of mRNA transcripts by real-time PCR
Differential expression of 10 genes was verified using quantitative one-step RT-PCR. Total RNA, isolated from three individual chick tibial growth plate samples on the fourth day, served as a template for amplification using an ABI PRISM 7900 Sequence Detection System (Applied Biosystems). RT-PCR transcripts from each chick were amplified in triplicate and detected using the QuantiTect SYBR Green RT-PCR kit (Qiagen). All primers used () were synthesized by Genery Biotechnology (Shanghai, China). The thermal cycling parameters were as follows: 50°C for 30 min, 95°C for 1 min; 40 cycles at 95°C for 15 sec; 56°C to 60°C for 15 sec (the annealing temperature varied depending on the gene specific primers); and 72°C for 45 sec. At the 72°C step, fluorescent data acquisition was performed. Following PCR cycling, disassociation curve analysis was performed at 95°C for 15 sec, 60°C for 15 sec and 95°C for 15 sec; data acquisition was performed at the final 60°C to 95°C ramp and at the final 95°C step. Analysis of the disassociation curves (as well as agarose gel electrophoresis) confirmed that a fluorescent signal was generated only from specific cDNA transcripts. The housekeeping gene β-actin was used as the endogenous assay control. Relative quantification of gene amplification by real-time PCR was performed using the cycle threshold (Ct) values. The fold change in expression of the target gene is presented as log2 of the difference between averaged Ct values for the control and thiram-fed chicks.
Table 1. Primers used in real-time PCR analysis
Statistical analysis
Data from the real-time PCR (Ct values) were analysed by Student t test using SPSS12.0. P<0.05 was considered significant, and P<0.01 was considered very significant.
Identification of differential expression genes by immunohistochemistry
Tibiae were removed immediately from chicks sacrificed by cervical dislocation on days 4, 9, 16 and 23, and were fixed overnight in 4% paraformaldehyde in phosphate-buffered saline at 4°C. Serial histological sections were prepared after the samples had been decalcified fully in 10% ethylenediamine tetraacetic acid decalcifying fluid and dehydrated in graded ethanol solutions, cleared in xylene and embedded in paraffin wax. The sections were deparaffinized in xylene, rehydrated through series of graded ethanol solutions, rinsed for 5 min in distilled water, and incubated in 3% H2O2 at room temperature for 10 min. Collagen type I (Col I) and heat shock protein 90 (Hsp90) were detected by immunohistochemistry with Col I and Hsp90 antibodies at a 1:100 dilution using the Enhanced Sensitive IH Detection Kit I (BBCL, WuHan, China) according to the manufacturer's protocol. Rabbit Anti-Col I and Rabbit Anti-Hsp90α were obtained from Boster Biotechnology Co. Ltd (Wuhan, China). As a negative control, non-immune rabbit IgG was substituted for the primary antibody.
Results
Screening of the SSH cDNA libraries
The library containing up-regulated genes was named Broiler TD Forward (BTDF), and the library that included down-regulated genes was termed Broiler Control Reverse (BCR). Approximately 400 clones in each library were generated. Of these, 40 positive clones from the libraries were identified using a dot blot assay.
Ten homologous gene sequences shared more than 99% identity with genes known in chicken (Gallus Gallus). Of the 10 genes, the expression of collagen type X (Col X), NADH dehydrogenase 1 beta subcomplex 5 (NADH DH), pro-alpha-1 (I) collagen (Col I), cytochrome C oxidase subunit III (COX III), CA2, alpha (IX) collagen-proteoglycan (Col IX) and enolase 1, alpha (ENO1) and Hsp90 genes were up-regulated, while matrilin 3 (MATN3), leukocyte cell-derived chemotaxin 1 (LECT1)—which also named chondromodulin-I (ChM-I)—were down-regulated at the early stage of the pathogenic process of TD in broilers ().
Table 2. Up-regulated genes and down-regulated genes isolated by SSH and dot blot analysisa
Confirmation of differential expression genes by real-time-PCR
The relative levels of 10 gene transcripts were normalized to β-actin in control or thiram-fed groups, were analysed and are presented in . The levels of expression of Col X, NADH DH, Col I, COX III, CA2, Col IX, ENO1 and Hsp90 gene mRNA transcripts were significantly higher in the thiram-fed chickens, as compared with that in control chickens, but the levels of the expression of MATN3 and ChM-I were significantly decreased in the thiram-fed chickens.
Figure 2. Analysis of the genes differentially expressed in growth plates. The levels of mRNA transcripts for 10 genes were determined by real-time PCR. Relative quantification of gene amplification was performed using the Ct values. Statistical differences were analysed by Student t test. *P<0.05, significant; **P<0.01, very significant.
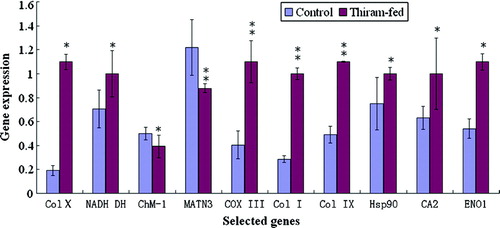
Identification of differential expression genes by immunohistochemistry
Col I and Hsp90 protein synthesis were localized in the prehypertrophic and hypertrophic chondrocyte of control or TD growth plates by immunohistochemistry ( and ). Noticeably increased Col I and Hsp90 were detected in TD growth plates on days 4, 9, 16 and 23 after feeding the thiram diet, which mirrored with their mRNA differential expression, and increased Hsp90 staining is clearly seen at days 16 and 23 especially (, T and , T). At days 16 and 23, the synthesis of Col I and Hsp90 was identified in hypertrophic chondrocytes around TD lesion ( and , day 16 and 23 T_H).
Figure 3. Expression changes of Col I in control and thiram-fed chicken growth plates at different stages. (Bar=100 µm.) Col I mainly lies in the prehypertrophic and hypertrophic zones with brown colour, but increased Col I was detected in the thiram-fed chicken growth plate on days 4, 9, 16 and 23 or around the TD lesion at the 16th and 23rd days. C, control; T, thiram-fed; PH, prehypertrophic zone; H, hypertrophic zone.
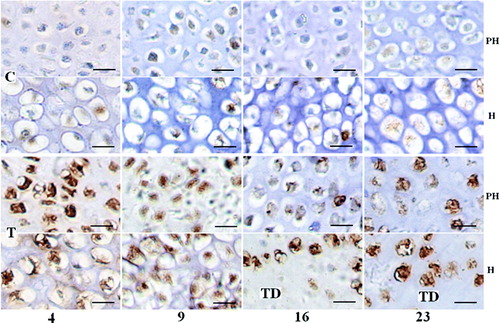
Figure 4. Expression changes of Hsp90 in control and thiram-fed chicken growth plates at different stages. (Bar=100 µm.) Hsp90 mainly lies in the prehypertrophic and hypertrophic zones with brown colour, but increased Hsp90 was detected in the thiram-fed chicken growth plate on days 4 and 9, and especially at days 16 and 23 or around the TD lesion at the 16th and 23rd days. C, control; T, thiram fed; PH, prehypertrophic zone; H, hypertrophic zone.
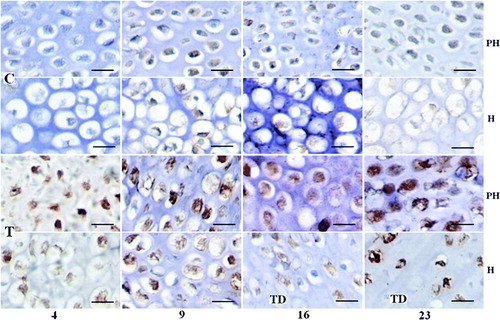
Discussion
Since the initial description of TD by Leach & Nesheim (Citation1965), many factors have been thought to contribute to TD development. However, little is known about the mechanism(s) underlying the pathogenesis of TD due to diverse causative factors and different experimental models. Thiram is a fungicide and has been demonstrated to induce TD with high regularity and precision (Rath et al., Citation2005). We used this experimental model to understand the cause of the defects associated with TD by selecting and identifying the genes differentially expressed in the TD growth plate of broiler chickens. Two cDNA libraries were generated from control or thiram-fed TD chickens by SSH, a technology that has been demonstrated to effectively identify differentially expressed genes. Following repeated screening, a total of 10 genes were found to be differentially expressed in TD chickens as compared with that in controls. Real time-PCR analysis of these gene transcripts confirmed that the levels of Col X, NADH DH, Col I, COX III, CA2, Col IX, ENO1 and Hsp90 transcripts were significantly up-regulated in TD chickens as compared with that in controls, while the levels of expression of MATN3 and ChM-I were significantly down-regulated in the thiram-fed chickens. Both Col I and Hsp90 results also corresponded to the results obtained by immunohistochemistry. Their functions were predicted to be in the formation of extracellular matrix, endochondral ossification, developmental regulation, electron transport in mitochondria and vascularization. Conceivably, these differentially expressed genes at the early stage of TD may contribute to the pathogenesis of TD.
Type X collagen, which is a recognized marker of chondrocyte hypertrophy, is exclusively expressed by hypertrophic chondrocytes and is an integral extracellular matrix macromolecule to the process of mineralization (Schmid & Linsenmeyer, Citation1987; Shen, Citation2005). Interestingly, we found that the levels of type X collagen gene transcripts in TD growth plates were up-regulated. Our data also agree with previous findings (Wardale & Duance, Citation1996), and suggest that the expression of type X collagen gene is up-regulated at the early stage of the pathogenic process of TD. Reginato et al. (Citation1998) also tested whether the accumulation of type X collagen in TD cartilage results from its increased biosynthesis, which is due largely to increased expression of the gene for this collagen; and up-regulated expression of type X collagen gene has been observed in cultured TD chondrocytes by Reginato et al. But up-regulated expression of type X collagen gene does not agree with findings of other who reported that chondrocytes of the TD lesion show reduced type X collagen, no change in type X collagen or type X collagen only expressed around the TD lesion (Chen et al., Citation1993; Pines et al., Citation1998; Webster et al., Citation2003; Rath et al., 2005). These inconsistencies may be attributed to samples collected from varying stages of the TD lesion. Furthermore, it has been demonstrated that extracellular proteins such as type X collagen accumulating intracellularly is characteristic of endoplasmic reticulum stress, a cellular survival technique (Tsang et al., Citation2007). However, the altered differentiation of chondrocytes disrupts the highly coordinated events of endochondral ossification culminating in chondrodysplasia. We deduced that type X collagen gene expression could be variable at different stages in TD.
Type I collagen is the most abundant collagen, present in many connective tissues and in precartilaginous tissues, and also expressed by hypertrophic chondrocytes. Decreased production of collagen I usually causes mild osteogenesis imperfecta (Byers & Cole, Citation2002). Furthermore, the intracellular post-translational modifications of type I procollagen are critical for bone formation (Barnes et al., Citation2006). Our data indicated that the expression of Col Iα1 gene was up-regulated significantly in TD chickens, which may reflect the response of TD growth plates to lower levels of Col I in the extracellular matrix.
Matrilin-3 is mainly expressed in the proliferation zone of a growth plate and also in the maturation zone. Through its coiled coil domain, matrilin-3 forms disulphide-linked oligomers of homotetramers, heterotrimers and tetramers, with matrilin-1, contributing to the matrix maturation process during endochondral ossification (Zhang & Chen, Citation2000). Collagen IX stabilizes the individual homogeneously occurring fibrils (Eikenberry & Bruckner, Citation1999) and is thought to direct the organization at the tissue level of the fibrillar network in cartilage. Interestingly, matrilin-3 can integrate into cartilage fibrils by a direct interaction with collagen IX and, as an adapter, indirectly with the cartilage oligomeric matrix protein (Budde et al., Citation2005). Therefore, matrilin-3 is considered an interface component, capable of interconnecting macromolecular networks and mediating interactions between cartilage fibrils and the extra fibrillar matrix (Wagener et al., Citation2005. Our data showed lower levels of matrilin-3 and higher levels of collagen IX mRNA transcripts in TD lesions, suggesting that their abnormal expression resulted in the pathogenic process of TD in chickens. However, whether the down-regulation of matrilin-3 and up-regulation of collagen IX gene expression is associated with change in the levels of the mature collagen cross-linked hydroxylysyl-pyridinoline remain to be investigated as it was significantly higher in dyschondroplastic lesions (Wardale & Duance, Citation1996).
LECT1, also named ChM-I, is an angiogenesis inhibitor (Hiraki et al., Citation1991, Citation1997). In chicken, ChM-I is expressed in precartilaginous tissues, the heart and eyes during early development, which is down-regulated by retinoic acid, indicating that ChM-I expression is chondrocyte-specific at the late stages (day 17) of embryonic development (Dietz et al., Citation1999). Lower levels of blood vessels or non-vascularized cartilage in TD birds could result in down-regulated expression of the ChM-I gene.
CA2 is extensively distributed in multiple tissues and involved in many kinds of physiological and pathological processes. CA2 in osteoclast plays an important role in bone resorption. Deficiency of CA2 can cause inherited infantile osteopetrosis with distal renal tubular acidosis in human (Borthwick et al., Citation2003). Gay et al. (Citation1985) found that activity of CA2 was diminished in the proximal part of the lesion and was zero in the distal lesion. The enzymes are believed to be diminished by necrotic and autolytic processes, although it is not involved in initiating the formation of the lesion. The up-regulated expression of CA2 gene at the early stage of TD chicken may involve in cartilage calcification by promoting bone resorption.
NADH DH, cytochrome C oxidase (COX) and ENO1 are important enzymes for transmitting electrons in the mitochondrial respiratory chain and in glucose metabolism. Thiram may oxidize cellular thiol-group-containing compounds, resulting in cellular injury and death by interfering with the –SH group(s) of numerous critical proteins and enzymes (Catherine et al., Citation2001). Thiram can inhibit mitochondrial succinate dehydrogenase and cause irreversible mitochondrial injury by inducing the mitochondrial permeability transition (Balakirev & Zimmer, Citation2001). Our study found that the expression of NADH DH, COX and ENO1 genes were up-regulated in TD growth plates of broiler. Our findings support the notion that thiram creates a metabolic dysfunction, leading to the development of TD in broiler by irreversible mitochondrial injury.
Heat shock proteins are stress-responsive proteins, and Hsp90 has various cellular functions, including conformational regulation of a variety of proteins that participate in signalling (Buchner, Citation1999). In embryogenesis, Hsp90 plays an important role in neural, retina, bone, and muscle development (Csermely et al., Citation1998). A large body of work spanning the past decade has identified the molecular chaperone Hsp90 as a critical modulator of an extensive network of cellular signalling pathways, including cell cycle control, gene transcription, and apoptotic signalling (Cullinan & Whitesell, Citation2006). In a recent study, Genin et al. (Citation2008) demonstrated that the TD growth plate is hypoxic, probably because the lack of vascularization and hypoxia leads to increases in the levels of Hsp90 and Hsp70. In the present study, we found that the expression o f Hsp90 gene was up-regulated at the early stage of TD chickens. These data suggested Hsp90 may regulate the cartilage development by various of regulate pathways.
Our previous studies have shown that thiram could promote chicken growth plate chondrocyte proliferation, and disturb the regulation of endochondral calcification and the development of normal cartilage, resulting in prehypertrophic cell accumulation, angionecrosis, abnormal extracellular matrix synthesis, defer endochondral calcification and bone resorption (unpublished observations). Data from the present study would support our hypothesis that thiram induces angionecrosis in growth plates, which down-regulates the chondromodulin-I expression, promoting vascularization of growth plates with TD lesion. Subsequently, these pathogenic processes may disturb the synthesis of extracellular matrix by up-regulating the expression of type IX collagen and adaptor protein gene matrilin-3, type X collagen, type I collagen, and CA2, and in turn delay the endochondral calcification. Therefore, the identification of these differentially expressed genes that are involved in the perturbation of chondrocyte differentiation in TD may provide new insights into understanding the molecular mechanism(s) of TD. Our findings may aid in design of new therapies for intervention of TD in chickens.
Acknowledgements
This study was supported by the China National Foundation of Science (30571370). The authors thank Dr Barbara Vertel (Chicago Medical School, USA), Dr E. Wayne Johnson (USA) and Dr Qing Yan Qiao (USA) for their helpful discussions and advice.
References
- Balakirev , M.Y. and Zimmer , G. 2001 . Mitochondrial injury by disulfiram: two different mechanisms of the mitochondrial permeability transition . Chemico-Biological Interactions , 138 : 299 – 311 .
- Barnes , A.M. , Chang , W. , Morello , R. , Cabral , W.A. , Weis , M. , Eyre , D.R. Leikin , S. 2006 . Deficiency of cartilage-associated protein in recessive lethal osteogenesis imperfecta . The New England Journal of Medicine , 355 : 2757 – 2764 .
- Ben-Bassat , S. , Genina , O. , Lavelin , I. , Leach , R.M. and Pines , M. 1999 . Parathyroid receptor gene expression by epiphyseal growth plates in rickets and tibial dyschondroplasia . Molecular and Cellular Endocrinology , 149 ( 1–2 ) : 185 – 195 .
- Borthwick , K.J. , Kandemir , N. , Topaloglu , R. and Karet , F.E. 2003 . A phenocopy of CAII deficiency: a novel genetic explanation for inherited infantile osteopetrosis with distal renal tubular acidosis . Journal of Medical Genetics , 40 : 115 – 121 .
- Buchner , J. 1999 . Hsp90 & Co.: a holding for folding . Trends Biochemical Science , 24 : 136 – 141 .
- Budde , B. , Blumbach , K. , Ylöstalo , J. and Grässel , S. 2005 . Altered integration of matrilin-3 into cartilage extracellular matrix in the absence of collagen IX . Molecular and Cellular Biology , 25 : 10465 – 10478 .
- Byers , P.H. and Cole , W.G. 2002 . “ Osteogenesis imperfecta ” . In Connective Tissue and its Heritable Disorders: Molecular, Genetic, and Medical Aspects , 2nd edn , Edited by: Royce , P.M. and Steinmann , B. 385 – 430 . New York : Wiley-Liss Inc .
- Catherine , C. , Sophie , B. , Parviz , P. and André , R. 2001 . Thiram-induced cytotoxicity is accompanied by a rapid and drastic oxidation of reduced glutathione with consecutive lipid peroxidation and cell death . Toxicology , 163 : 153 – 162 .
- Chen , Q. , Gibney , E.P. , Leach , R.M. and Linsenmayer , T.F. 1993 . Chicken tibial dyschondroplasia: a limb mutant with two growth plates and possible defects of collagen crosslinking . Developmental Dynamics , 196 : 54 – 61 .
- Csermely , P. , Schnaider , T. , Soti , C. , Prohaszka , Z. and Nardai , G. 1998 . The 90-kDa molecular chaperone family: structure, function, and clinical applications. A comprehensive review . Pharmacology & Therapeutics , 79 : 129 – 168 .
- Cullinan , S.B. and Whitesell , L. 2006 . Heat shock protein 90: a unique chemotherapeutic target . Seminars in Oncology , 33 : 457 – 565 .
- Dietz , U.H. , Ziegelmeier , G. , Bittner , K. and Balling , R. 1999 . Spatio-temporal distribution of chondromodulin-I mRNA in the chicken embryo: expression during cartilage development and formation of the heart and eye . Developmental Dynamics , 216 : 233 – 243 .
- Eikenberry , E.F. and Bruckner , P. 1999 . “ Supramolecular structure of cartilage matrix ” . In Bone and Cartilage Metabolism , Edited by: Seibel , M.J. , Robins , S.P. and Bilezikian , J.P. 289 – 300 . San Diego , CA : Academic Press .
- Gay , C.V. , Anderson , R.E. and Leach , R.M. 1985 . Activities and distribution of alkaline phosphatase and carbonic anhydrase in the tibial dyschondroplastic lesion and associated growth plate of chicks . Avian Disease , 29 : 812 – 882 .
- Genin , O. , Hasdai , A. , Shinder , D. and Pines , M. 2008 . Hypoxia, hypoxia-inducible factor-1{alpha} (HIF-1α, and heat-shock proteins in tibial dyschondroplasia . Poultry Science , 87 ( 8 ) : 1556 – 1564 .
- Hiraki , Y. , Tanaka , H. , Inoue , H. and Suzuki , F. 1991 . Molecular cloning of a new class of cartilage-specific matrix, chondromodulin-I, which stimulates growth of cultured chondrocytes . Biochemical Biophysiology Research Communications , 175 : 971 – 977 .
- Hiraki , Y. , Inoue , H. and Iyama , K. 1997 . Identification of chondromodulin I as a novel endothelial cell growth inhibitor: purification and its localization in the avascular zone of epiphyseal cartilage . The Journal of Biological Chemistry , 272 : 32419 – 32426 .
- Knopov , V. , Leach , R.M. , Barak-Shalom , T. , Hurwitz , S. and Pines , M. 1995 . Osteopontin gene expression and alkaline phosphatase activity in avian tibial dyschondroplasia . Bone , 16 : 329S – 334S .
- Leach , R.M. and Nesheim , M.C. 1965 . Nutritional, genetic and morphological studies of an abnormal cartilage formation in young chicks . The Journal of Nutrition , 86 : 236 – 244 .
- Pines , M. , Knopov , V. , Genina , O. , Hurwitz , S. , Faerman , A. Gerstenfeld , L.C. 1998 . Development of avian tibial dyschondroplasia: gene expression and protein synthesis . Calcified tissue international , 63 : 521 – 527 .
- Praul , C.A. , Ford , B.C. , Gay , C.V. , Pines , M. and Leach , R.M. 2000 . Gene expression and tibial dyschondroplasia . Poultry Science , 79 : 1009 – 1013 .
- Rath , N.C. , Huff , W.E. , Balog , J.M. , Bayyari , G.R. and Reddy , R.P. 1997 . Matrix metalloproteinase activities in avian tibial dyschondroplasia . Poultry Science , 76 : 501 – 505 .
- Rath , N.C. , Richards , M.P. and Huff , W.E. 2005 . Changes in the tibial growth plates of chickens with thiram-induced dyschondroplasia . Journal of Comparative Pathology , 33 : 41 – 52 .
- Reginato , A.M. , Bashey , R.I. , Rosselot , G. , Leach , R.M. , Gay , C.V. and Jimenez , S.A. 1998 . Type X collagen biosynthesis and expression in avian tibial dyschondroplasia . Osteoarthritis Cartilage , 6 : 125 – 136 .
- Schmid , T.M. and Linsenmeyer , T.F. 1987 . “ Type X collagen ” . In Structure and Function of Collagen Types , Edited by: Mayne , R. and Burgeson , R.E. 223 – 259 . London : Academic Press .
- Shen , G. 2005 . The role of type X collagen in facilitating and regulating endochondral ossification of articular cartilage . Orthod Craniofacial Research , 8 : 11 – 17 .
- Shirley , R.B. , Davis , A.J. , Compton , M.M. and Berry , W.D. 2003 . The expression of calbindin in chicks that are divergently selected for low or high incidence of tibial dyschondroplasia . Poultry Science , 82 : 1965 – 1973 .
- Tsang , K.Y. , Chan , D. , Cheslett , D. , Chan , W.C. , So , C.L. Melhado , I.G. 2007 . Surviving endoplasmic reticulum stress is coupled to altered chondrocyte differentiation and function . PLoS Biology , 5 ( 3 ) : 568 – 585 .
- Twal , W.O. , Wu , J. , Gay , C.V. and Leach , R.M. Jr . 1996 . Immunolocalization of basic fibroblastic growth factor in avian tibial dyschondroplastic cartilage . Poultry Science , 75 : 130 – 134 .
- Wagener , R. , Ehlen , H.W. , Ko , Y.P. , Kobbe , B. , Mann , H.H. , Sengle , G. and Paulsson , M. 2005 . The matrilins—adaptor proteins in the extracellular matrix . FEBS Letters , 579 : 3323 – 3329 .
- Wardale , R.J. and Duance , V.C. 1996 . Collagen expression in chicken tibial dyschondroplasia . Journal of Cell Science , 109 : 1119 – 1131 .
- Webster , S.V. , Farquharson , C. , Jefferies , D. and Kwan , A.P. 2003 . Expression of type X collagen, Indian hedgehog and parathyroid hormone related-protein in normal and tibial dyschondroplastic chick growth plates . Avian Pathology , 32 : 69 – 80 .
- Whitehead , B.C. 1997 . Dyschondroplasia in poultry . Proceedings of the Nutrition Society , 56 : 957 – 966 .
- Wu , J. , Pines , M. , Gay , C.V. , Hurwitz , S. and Leach , R.M. Jr . 1996 . Immunolocalization of osteonectin in avian tibial dyschondroplastic cartilage . Developmental Dynamics , 207 : 69 – 74 .
- Zhang , W.P. , Li , H.Y. , Cheng , G.F. and Hu , S. 2008 . Avian influenza virus infection induces differential expression of genes in chicken kidney . Research in Veterinary Science , 84 ( 3 ) : 374 – 381 .
- Zhang , Y. and Chen , Q. 2000 . Changes of matrilin forms during endochondral ossification . The Journal of Biological Chemistry , 275 : 32628 – 32634 .