Abstract
Avian metapneumovirus (aMPV) is an important respiratory pathogen of turkeys with considerable economic impact on poultry production. Although vaccination is widely used for the control of the disease, questions regarding vaccine safety and efficacy remain to be elucidated. This report describes the problems associated with reproducibility of the aMPV-vaccine response, comparing T-lymphocyte-compromised and T-cell-intact turkeys. In three consecutive experiments, turkeys partially depleted of T-lymphocytes by treatment with cyclosporin A as well as untreated turkeys were vaccinated with a commercial live aMPV subtype A (aMPV-A) vaccine at 2 weeks of age. Two weeks later they were challenged with a virulent aMPV-A strain. Despite similar genetic background of the turkeys, comparable housing conditions under isolation and the application of the same aMPV-A vaccine, considerable variation was observed among the experiments regarding replication of the vaccine virus, vaccine-induced clinical signs and protection against challenge infection. The results indicate that differences in the outcome of aMPV-A vaccination may be associated with T-lymphocyte suppression and additionally with an interfering aMPV-B vaccine exposure at the hatchery in two of the experiments. Our study provides possible explanations for the variable protection provided by aMPV vaccines under field conditions.
Introduction
Avian metapneumovirus (aMPV) is an important pathogen of turkeys and the causative agent of an acute disease of the upper respiratory tract, which is referred to as turkey rhinotracheitis (TRT) (Gough, Citation2003). TRT causes considerable losses to the turkey industry, mainly due to secondary bacterial infections (Cook et al., Citation1991; Van de Zande et al., Citation2001; Marien et al., Citation2005; Rubbenstroth et al., Citation2009). Four aMPV subtypes, designated A to D, have been identified based on the sequence of the attachment glycoprotein (G) gene (Juhasz & Easton, Citation1994; Bäyon-Auboyer et al., Citation2000; Toquin et al., Citation2003). aMPV subtypes A (aMPV-A) and B (aMPV-B) are widely distributed in Europe, Asia, Africa and South America (Van de Zande et al., Citation1998; Mase et al., Citation2003; Banet-Noach et al., Citation2005; Chacon et al., Citation2007; Owoade et al., Citation2008). Both subtypes are antigenically related and induce development of cross-neutralizing antibodies and cross-protection against infection and disease in turkeys (Cook et al., Citation1993, Citation1995, Citation1999; Van de Zande et al., Citation2000).
Vaccination is widely used for the control of aMPV in turkey flocks. Although vaccines have been shown to provide good protection under experimental conditions, aMPV field infections may still occur and cause respiratory problems in vaccinated flocks (Jones, Citation1996; Van de Zande et al., Citation1998; Banet-Noach et al., Citation2009). Previous work has shown that humoral immunity does not provide sufficient protection against aMPV (Naylor et al., Citation1997; Rubbenstroth & Rautenschlein, Citation2009). In addition, vaccination of chemically B-lymphocyte-suppressed turkeys resulted in full protection against aMPV-challenge infection, despite the lack of detectable aMPV-specific antibodies (Jones et al., Citation1992). These results indicate that cell-mediated immune mechanisms may provide protection against aMPV. In a recently published study we demonstrated that T-lymphocytes may play an important role in the control and clearance of primary aMPV infection in turkeys (Rubbenstroth et al., Citation2010). However, few experimental data are available on the role of T-lymphocytes in the protection against aMPV infection.
Chemical T-lymphocyte suppression with cyclosporin A (CsA) was shown to be a useful tool to study the role of T-lymphocytes in different avian species, including turkeys (Nowak et al., Citation1982; Fitzgerald et al., Citation1995; Suresh & Sharma, Citation1995; Khehra & Jones, Citation1999; Kim et al., Citation2000). CsA selectively inhibits T-lymphocytes during the early phase of activation and reduces production of interleukin 2 at the transcript level (Schnetzler et al., Citation1983; Schreiber & Crabtree, Citation1992; Zenke et al., Citation1993; Resch & Szamel, Citation1997).
In the present study we evaluated the effect of T-cell suppression on the response of turkeys to vaccination with an attenuated aMPV vaccine and on the development of protection against challenge. In three consecutive experiments, CsA-treated and untreated turkey poults were inoculated with a live aMPV-A vaccine. Two weeks later they were challenged with a virulent aMPV-A strain. Development of clinical signs, histopathological lesions, detection of aMPV by reverse transcription polymerase chain reaction (RT-PCR) and induction of aMPV-specific antibodies were recorded. The immunosuppressive effect of CsA was confirmed by flow-cytometric techniques.
Materials and Methods
Turkeys
For Experiment 1, B.U.T. Big-6 turkey eggs were provided by a commercial hatchery and hatched at the Clinic for Poultry, University of Veterinary Medicine Hannover. For Experiments 2 and 3, 1-day-old female Big-6 turkey poults were obtained from the same commercial hatchery. Both batches of poults, as well as the eggs, originated from parent flocks located in a region in which aMPV is not endemic. No aMPV vaccination was performed in the parent flocks to ensure that the poults were free of maternal antibodies against aMPV. Before the beginning of the experiments, the turkeys were confirmed to be negative for aMPV-specific maternal antibodies by enzyme-linked immunosorbent assay (ELISA) and virus neutralization test (VNT). The absence of aMPV-A was confirmed by RT-PCR. Turkeys used in Experiments 2 and 3 had been exposed to an aMPV-B vaccine in the hatchery, as detected by RT-PCR at 1 or 2 weeks post hatch, respectively.
Poults were housed on wood shaving litter in isolation units of the Clinic for Poultry, following animal welfare guidelines. Water and commercial feed were provided ad libitum.
Treatment, vaccination and challenge infection of turkeys
Turkeys were treated with CsA (Sandimmun 100 mg capsules; Novartis, Nürnberg, Germany) by intramuscular injection of 100 mg/kg bodyweight at intervals of 3 to 4 days throughout the experiments (Suresh & Sharma, Citation1995; Rubbenstroth et al., Citation2010). They were vaccinated with a commercial aMPV-A live vaccine by oculonasal inoculation. All vaccine vials used in the present study originated from the same batch. For challenge infection, the virulent aMPV-A strain BUT 8544 (Wilding et al., Citation1986), kindly provided by R.C. Jones (Liverpool University, UK), was used. The isolate was propagated and titrated in chicken embryo tracheal organ cultures (Cook et al., Citation1976). Median ciliostatic doses (CD50) were calculated according to the method of Reed & Muench (Citation1938). In all three experiments, turkeys were challenged oculonasally with 103 CD50 per bird.
Confirmation of T-lymphocyte suppression after CsA treatment
Relative proportions and absolute numbers of CD4+ and CD8α+ T-lymphocytes in peripheral blood mononuclear cells (PBMC) were determined by flow-cytometric analysis. Relative lymphocyte proportions were determined in Experiments 1 and 3. Briefly, PBMC were purified using sucrose gradient purification. Subsequently lymphocytes were stained with fluorescent antibodies and detected using flow-cytometric analysis (Liman & Rautenschlein, Citation2007; Rubbenstroth et al., Citation2010). In Experiment 2, absolute lymphocyte numbers in peripheral blood were determined. Blood samples were diluted and fixed before staining with lymphocyte-specific fluorescent antibodies. Prior to flow-cytometric analysis, a defined number of fluorescent beads was added to each sample. The total number of lymphocytes was calculated by relating the number of counted lymphocytes to the number of counted beads (Burgess & Davison, Citation1999; Rubbenstroth et al., Citation2010).
Inhibition of T-lymphocyte activity was determined in a flow-cytometric proliferation assay. Purified PBMC were stained with carboxyfluorescein succinimidyl ester (Sigma-Aldrich, Steinheim, Germany) and stimulated ex vivo with the T-cell mitogens Concanavalin A (5 µg/ml; Sigma, Saint Louis, Missouri, USA) and phytohaemagglutinin (5 µg/ml; Sigma) for 2 days, before cells were harvested and stained with lymphocyte-specific antibodies. Lymphocytes with reduced intensity of carboxyfluorescein succinimidyl ester staining were considered proliferated cells (Lyons & Parish, Citation1994; Rubbenstroth et al., Citation2010).
Clinical signs and histopathology
Turkeys were observed daily for clinical signs following vaccination and challenge. Respiratory signs were recorded according to a previously published scoring system with a total daily score of 0 to 9 for each bird (Rubbenstroth & Rautenschlein, Citation2009). For histopathological examination, samples of trachea and nasal turbinates were collected post mortem, fixed in 10% phosphate-buffered formalin and embedded in paraffin. Tissue sections were stained with haematoxylin and eosin. Sections were analysed by light microscopy in a blinded fashion (Rubbenstroth & Rautenschlein, Citation2009).
aMPV detection by nested RT-PCR
A subtype-specific RT-PCR was applied for the detection of aMPV-specific RNA (Cavanagh et al., Citation1999). RNA was extracted from choanal swabs with Trifast GOLD (Peqlab, Erlangen, Germany). The RT reaction was performed using the ImProm-II® RT system (Promega, Madison, Wisconsin, USA). For PCR steps, SAWADY Taq-DNA-Polymerase (Peqlab, Erlangen, Germany) was used. PCR products were separated by agarose gel electrophoresis and visualized by ethidium bromide staining and ultraviolet transillumination. Detailed procedures have been published elsewhere (Rubbenstroth & Rautenschlein, Citation2009).
Detection of aMPV-specific antibodies
aMPV-specific IgG levels in serum samples were measured by the Avian Rhinotracheitis Antibody (ART) ELISA Test Kit (BioChek, Gouda, The Netherlands) following the manufacturer's instructions. Lachrymal fluid was collected with filter paper discs (6 mm; Schleicher and Schüll, Dassel-Einbeck, Germany) carefully placed underneath the eyelid of the turkey. Antibodies were detected using the ART ELISA Kit with six-fold diluted lachrymal fluid. ELISA results are presented as sample to positive control (S/P) ratios (Rubbenstroth & Rautenschlein, Citation2009). Virus neutralizing (VN) antibodies in serum samples were detected by VNT following previously published protocols (Baxter-Jones et al., Citation1989; Rubbenstroth & Rautenschlein, Citation2009).
Experimental Design
Three experiments have been conducted in which CsA-treated and untreated turkeys were vaccinated with a live aMPV subtype A vaccine and subsequently challenged with a virulent aMPV subtype A strain. The experimental design is summarized in . This paper will focus on the results of the CsA-treated and untreated vaccinated groups. Results of the CsA-mediated T-lymphocyte suppression and the primary aMPV infection have been described in detail in a separate publication (Rubbenstroth et al., Citation2010).
Table 1. Experimental design.
Experiment 1
Fifty-four turkey poults were randomly assigned to eight groups, which were housed in the same room. Four groups (Groups TCC-1, TVC-1, TCA-1 and TVA-1) were CsA-treated starting at 6 days of life (dol), while the remaining four groups were not treated (). At 16 dol, Groups CVC-1/CVA-1 and TVC-1/TVA-1 were moved to two separate rooms and vaccinated with a commercial aMPV-A live vaccine by oculonasal inoculation. The dose per bird was approximately 103.5 median cell culture infectious doses (CID50), according to the information given by the manufacturer. At 30 dol (14 days post vaccination [d.p.v.]), each of Groups CCA-1, TCA-1, CVA-1 and TVA-1 was moved to a separate room and inoculated oculonasally with 103 CD50 virulent aMPV-A per bird. The experiment was terminated at 44 dol (14 days post challenge [d.p.c.]) and all turkeys were exsanguinated and examined post mortem.
Blood samples for determination of lymphocyte counts were collected at weekly intervals. Choanal swabs were collected for detection of aMPV by RT-PCR at intervals of 3 to 7 days throughout the experiment. Serum samples were collected weekly for detection of aMPV-specific antibodies. Lachrymal fluids for detection of aMPV-specific ELISA-IgG were collected at weekly intervals, beginning at the day of challenge infection. Clinical signs were recorded daily following vaccination and challenge. Samples of trachea and nasal turbinates were collected for histopathological examination at post mortem.
Experiment 2
A second experiment with 119 turkey poults was conducted with a similar experimental design including minor variations (). CsA treatment was started at 4 dol. Turkeys were vaccinated at 13 dol with a dose >103.2 CID50 per bird and challenged at 27 dol (14 d.p.v.). At 7 d.p.c., five to eight turkeys of each group were humanely killed. The experiment was terminated at 41 dol (14 d.p.c.), when the remaining turkeys were humanely killed and subjected to post-mortem examination.
Experiment 3
In a third experiment, only Groups CCC-3, CVA-3 and TVA-3 were included, with six turkeys per group. CsA treatment was started at 5 dol (). Vaccinated groups received >103.2 CID50 of the vaccine per bird at 14 dol and were challenged at 29 dol (15 d.p.v.). The experiment was terminated at 48 dol (19 d.p.c.). Tissue samples for histopathological examination and lachrymal fluids were not collected in this experiment.
Statistical analysis
Statistical analysis of the ELISA and VNT results was performed by Statistix 9 software (Analytical Software, Tallahassee, Florida, USA), using one-way analysis of variance and comparison of means by the Tukey test. P <0.05 was considered to indicate significant difference.
Results
T-lymphocyte suppression
CsA treatment of turkeys consistently resulted in T-lymphocyte suppression in all three experiments. Numbers of CD4+-positive and CD8α+-positive T-lymphocytes in peripheral blood were significantly reduced (P <0.05) in CsA-treated turkeys, as compared with untreated groups (data not shown). This occurred as early as 7 days after the beginning of CsA treatment and remained at levels of 50 to 80% reduction throughout the experiments (data not shown). Proliferative ex vivo mitogen responses of T-lymphocytes were also reduced in CsA-treated groups. Reduced proportions of proliferated T-cells correlated with the reduced number of T-cells in PBMC (data not shown). Results of CsA-mediated T-cell suppression are described in detail in Rubbenstroth et al. (Citation2010).
Detection of aMPV-B by RT-PCR
In Experiments 2 and 3, 1-day-old turkey poults had been obtained from a commercial hatchery. In both experiments an aMPV-B strain was detected by RT-PCR. In Experiment 2 aMPV-B was present in choanal swabs collected at 7 to 20 dol, whereas in Experiment 3 it was first detected at 14 dol and was found sporadically until the end of the experiment. In both experiments aMPV-B was detected in all groups with no apparent differences regarding detection rates and time (data not shown). No aMPV-B was detected in Experiment 1, for which turkeys were hatched at the Clinic for Poultry.
Detection of aMPV-A by RT-PCR
aMPV-A vaccine virus detection varied considerably between the experiments. In Experiment 1 the aMPV-A vaccine virus was detected by RT-PCR in 50 to 100% of the choanal swabs collected from the vaccinated groups at 4 and 7 d.p.v., and was rapidly cleared thereafter (). In Experiment 2 aMPV-A was detectable at low frequencies of up to 50% until 7 d.p.v. Thereafter detection rates reached peak levels of 63 and 75% positive swabs at 14 and 18 d.p.v. in Groups TVC-2 and CVC-2, respectively. The virus was detectable until 21 d.p.v. in Group TVC-2 and until 25 d.p.v. in Group CVC-2 (). In Experiment 3, the aMPV-A vaccine was barely detectable in either vaccinated group. Only one of six choanal swabs was aMPV-A-positive in the CsA-treated vaccinated Group TVA-3 at 8 d.p.v., whereas Group CVA-3 remained negative ().
Table 2. Detection of aMPV subtype A in choanal swabs collected from CsA-treated and untreated turkeys following aMPV vaccination and challenge infection.
Following challenge infection, aMPV-A was detected in 88 to 100% of the choanal swabs of unvaccinated birds in Experiments 1 and 2 (Groups CCA-1 and CCA-2; Groups TCA-1 and TCA1-2) at 4 and 7 d.p.c. Thereafter the virus was rapidly cleared from the untreated Groups CCA-1 and CCA-2, whereas 38 and 63% of the swabs collected from Groups TCA-1 and TCA-2, respectively, were still aMPV-A-positive at 14 d.p.c. (). In the vaccinated groups a considerable variation in aMPV detection was observed after challenge infection in the three experiments. In Experiment 1, only one out of eight swabs was found to be aMPV-A-positive in the untreated vaccine Group CVA-1 at 4 d.p.c., and no aMPV-A was detected in the CsA-treated vaccine Group TVA-1 after challenge. In contrast, aMPV-A detection rates in the CsA-treated vaccine group (TVA-3) of Experiment 3 were 100 and 83% positive swabs at 4 and 7 d.p.c., respectively. These results resembled those of the unvaccinated challenge groups, such as in Experiments 1 and 2. In the untreated vaccine group of Experiment 3 (Group CVA-3), aMPV-A was only detectable at 4 d.p.c., but not thereafter (). In Experiment 2, aMPV-A was detected in both vaccinated challenge groups (Group CVA-2 and TVA-2) until 11 d.p.c. However, since aMPV-A was also detectable in the unchallenged vaccine groups (Groups CVC-2 and TVC-2) at the same time we cannot determine whether the positive results in Groups CVA-2 and TVA-2 represent the virulent challenge virus or residual vaccine virus (). Sequence analysis of the detected aMPV-A in order to distinguish between vaccine and challenge strain was not performed. No aMPV-A was detected in any of the unvaccinated and unchallenged groups (Groups CCC and TCC) throughout the experiments ().
Clinical signs
Following aMPV vaccination, mild clinical signs such as watery eyes or clear nasal exudate were observed in Experiments 1 and 3 in up to 20% of vaccinated birds at 4 to 8 d.p.v. (data not shown). In contrast, mild to moderate clinical signs were observed between 11 and 18 d.p.v. in about one-half of the turkeys of all vaccinated groups in Experiment 2 (). In each of the CsA-treated, vaccinated groups (Groups TVC-2 and TVA-2), one turkey developed severe clinical signs with prominent periorbital swelling and subcutaneous oedema. Peak levels of clinical scores were recorded at 13 d.p.v. in CsA-treated, vaccinated turkeys (Group TVC-2) and 17 d.p.v. in the untreated vaccine group (Group CVC-2; data not shown).
Table 3. Development of clinical signs and histopathological lesions in CsA-treated and untreated turkeys following vaccination and challenge with virulent aMPV-A.
In unvaccinated groups, aMPV-A challenge induced typical TRT-like signs, such as nasal exudate and swollen infraorbital sinuses. Clinical signs started at 3 to 4 d.p.c. and clinical scores peaked at 6 to 7 d.p.c. In untreated challenge groups (Groups CCA-1 and CCA-2), clinical signs had completely waned by 10 d.p.c. In contrast, recovery of CsA-treated challenge groups (Groups TCA-1 and TCA-2) was delayed by about 2 days (Rubbenstroth et al., Citation2010), resulting in an increased accumulated clinical score as compared with the respective untreated groups (). In Experiment 1, both vaccinated challenge groups (Groups CVA-1 and TVA-1) were protected against clinical disease, displaying no or only very low accumulated clinical scores (). In Experiment 2, determination of challenge-induced signs was difficult due to the presence of vaccine related clinical signs. However, both vaccinated challenge groups (Groups CVA-2 and TVA-2) showed accumulated clinical scores that were comparable with the respective unchallenged vaccinated groups (Groups CVC-2 and TVC-2), indicating that virulent aMPV-A challenge did not induce additionally prominent clinical disease (). In Experiment 3, the untreated vaccinated group (Group CVA-3) did not develop signs following challenge. In contrast, the CsA-treated vaccine group (Group TVA-3) was not protected against clinical disease induced by virulent aMPV-A, and expressed prominent clinical signs and high accumulated clinical scores (). No clinical signs were recorded in the groups receiving neither vaccination nor challenge infection (Groups CCC and TCC) in all three experiments.
Histopathology
Histopathological lesions in the respiratory mucosa of nasal turbinates and trachea were investigated in Experiment 1 at 14 d.p.c. and in Experiment 2 at 7 and 14 d.p.c. Infection of unvaccinated turkeys with virulent aMPV-A induced prominent lesions, such as massive lymphoid infiltration and loss of cilia. All turkeys of CsA-treated challenge groups (Groups TCA-1 and TCA-2) had pronounced mucosal lesions at 7 and 14 d.p.c. In the untreated challenge groups (Groups CCA-1 and CCA-2), lesions were less prominent and the number of affected turkeys was lower, particularly at 14 d.p.c. ().
In Experiment 1, no mucosal lesions were present in the unchallenged vaccinated groups (Groups CVC-1 and TVC-1) at 14 d.p.c. (28 d.p.v.). In the vaccinated and challenged groups (Groups CVA-1 and TVA-1), a small number of turkeys (13 and 38%, respectively) expressed mild lesions. In Experiment 2, mild lesions were still present in the vaccinated groups (Groups CVC-2 and TVC-2) at 7 and 14 d.p.c. (21 and 28 d.p.v.) in up to 60% of the birds. Similar lesions were observed in up to 38% of the birds in the challenged vaccinated groups (Groups CVA-2 and TVA-2) of this experiment ().
No mucosal lesions were detected in the unvaccinated and unchallenged control groups (Groups CCC and TCC).
Antibody response
aMPV-specific antibodies were detected by VNT in serum samples and by IgG-ELISA in sera and lachrymal fluids. In Experiments 1 and 2, vaccination with attenuated aMPV-A induced significantly increased VN antibody titres of up to log2 6.3 and log2 5.2 in the CsA-treated (Group TVA) and untreated groups (Group CVA), respectively (a). In contrast, a significantly increased ELISA-IgG response was only detectable in sera and lachrymal fluids of CsA-treated birds (Group TVA; b, c). Maximal S/P ratios were 0.33 in sera and 0.64 in lachrymal fluids. In Experiment 3, exposure to the aMPV-B vaccine in the hatchery led to low titres of VN antibodies (log2 3.8) and ELISA-IgG S/P ratios (0.44) in sera of unvaccinated controls (Group CCC-3; ). Vaccination with attenuated aMPV-A did not significantly increase these levels (Group CVA-3; ). Surprisingly, CsA-treated turkeys (Group TVA-3) did not develop detectable antibodies following aMPV-A vaccination ().
Figure 1. Presence of aMPV-specific antibodies in turkey sera collected immediately before challenge infection at day 14 post vaccination (Experiments 1 and 2) or day 15 post vaccination (Experiment 3). 1a: VN antibodies, presented as mean VN log2 titres±standard deviation (SD) per group. 1b: Serum ELISA IgG antibodies and (1c) ELISA IgG antibodies in lachrymal fluids, presented as mean S/P ratio±SD per group. Values marked with different uppercase letters indicate significant differences between groups within one experiment (one-way analysis of variance and Tukey's comparison of means, P < 0.05). ND, not done. CCC, T-cell-intact, unvaccinated groups; TCC, CsA-treated, unvaccinated groups; CVC (Experiments 1 and 2) or CVA (Experiment 3), T-cell-intact, aMPV-A vaccinated groups; TVC (Experiments 1 and 2) or TVA (Experiment 3), CsA-treated, aMPV-A vaccinated groups. Experiments 1 and 2, n = 8; Experiment 3, n = 6. Turkeys in Experiments 2 and 3 had been exposed to an aMPV-B vaccine in the hatchery.
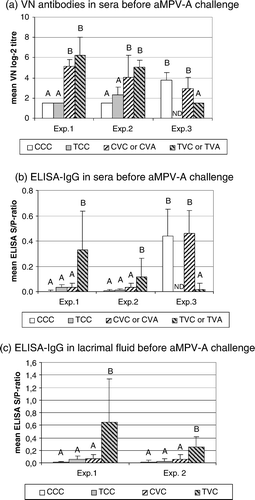
All antibody levels detected prior to challenge were very low, as compared with the response induced by challenge infection. Sera of turkeys infected with virulent aMPV-A reached VN titres of up to log2 8.5 and ELISA-IgG S/P ratios of up to 2.1. Maximal ELISA-IgG S/P ratios in lachrymal fluids were 2.5 (data not shown).
Discussion
The aim of the present study was to investigate the impact of CsA-induced T-lymphocyte suppression on the response of turkeys to attenuated aMPV-A vaccination and the development of protection against virulent aMPV-A challenge. In all three experiments, intramuscular treatment with 100 mg/kg CsA resulted in comparable T-lymphocyte suppression. This was characterized by 50 to 80% reduction of circulating CD4+ and CD8a+ T-cells and by a reduced proliferative response to T-cell mitogens, as described in detail in Rubbenstroth et al. (Citation2010).
Despite the similar genetic background of the birds, comparable housing conditions and aMPV-A vaccine application, we observed considerable variation regarding vaccine response and vaccination efficacy during the three experiments. Vaccination with the attenuated aMPV-A vaccine in Experiment 1 resulted in infrequent, but only short-termed, detection of the vaccine, with no vaccine-induced clinical signs and protection of CsA-treated and untreated turkeys against aMPV-A challenge. In Experiment 2, the aMPV-A vaccine strain was detectable infrequently for up to 25 d.p.v., accompanied by mucosal lesions and mild to moderate clinical signs especially in the CsA-treated groups. In Experiment 3, untreated turkeys developed full protection against challenge, although the aMPV-vaccine strain was barely detectable. In contrast, CsA-treated aMPV-vaccinated turkeys remained fully susceptible in this experiment. These differences may be explained by a variety of factors such as the interfering aMPV-B vaccine in Experiments 2 and 3, as well as the CsA-induced T-cell immunosuppression, which may have affected protection, as indicated in Experiment 3.
In Experiments 2 and 3, 1-day-old turkey poults had been obtained from a commercial hatchery and exposed to an aMPV-B vaccine, which was routinely applied by spray vaccination in the hatchery. We speculate that the presence of aMPV-B at the time of vaccination may have interfered with the aMPV-A vaccine replication, either by competition for possible target cells or indirectly by induction of innate immune mechanisms. Infection of primary chicken embryo cells with aMPV subtype C resulted in a prominent upregulation of several interferon-induced genes (Munir & Kapur, Citation2003). Liman & Rautenschlein (Citation2007) demonstrated that splenic leukocytes isolated from turkeys infected with an attenuated aMPV-B strain were primed to increased production of IFN type I ex vivo. By these means, the presence of the aMPV-B vaccine may contribute to the observed variation in aMPV-A vaccine detection between the three experiments. In Experiment 3 aMPV-A was barely detectable, and in Experiment 2 its replication was delayed as compared with the rapid aMPV-A vaccine detection and clearance in the absence of aMPV-B in Experiment 1. Delayed or reduced replication of aMPV vaccines was reported after co-administration with other respiratory viruses, such as Newcastle disease virus or infectious bronchitis virus (Cook et al., Citation2001; Ganapathy et al., Citation2007; Tarpey et al., Citation2007).
The observed variations in vaccine replication were accompanied by a different clinical response to the vaccine in the three experiments. While no or only very mild clinical signs were observed in a very small number of vaccinated birds in Experiments 1 and 3, aMPV-A-vaccinated birds in Experiment 2 showed more prominent clinical disease and mucosal lesions. Peak clinical scores were recorded at 13 and 17 d.p.v. in CsA-treated (Group TVC-2) and untreated turkeys (Group CVC-2), respectively. These findings correlate well with the peak levels of aMPV-A vaccine detection in the respective groups. Interestingly, the CsA-treated Group TVC-2 had a higher accumulated clinical score and a higher incidence of histopathological lesions at 7 d.p.c. (21 d.p.v.), as compared with the untreated Group CVC-2. Since no other pathogens were detected in Experiment 2 (data not shown), clinical signs and histopathological lesions were likely to be caused by the vaccine virus. Attenuated aMPV live vaccines were shown to possess residual virulence under experimental conditions (Cook et al., Citation1989a; Naylor & Jones, Citation1994; Gulati et al., Citation2001). Also reversion to full virulence was observed in the field (Catelli et al., Citation2006; Ricchizzi et al., Citation2008).
While untreated turkeys in Experiment 3 developed detectable levels of serum antibodies after exposure to the aMPV-B vaccine, the introduction of aMPV-B in Experiment 2 did not result in detectable VN or ELISA antibody levels in sera and lachrymal fluids. Vaccination with the live aMPV-A vaccine in Experiments 1 and 2 resulted in significantly increased VN antibody titres in sera of CsA-treated and untreated turkeys, whereas ELISA IgG antibodies were detectable only in sera and lachrymal fluid of CsA-treated birds. In Experiment 3, live vaccination with aMPV-A did not induce an additional increase of antibody levels, which correlates with the barely detectable replication of the vaccine in this experiment. Previous studies demonstrated that both assays used in the present study detect antibodies directed against aMPV-A and aMPV-B (Liman & Rautenschlein, Citation2007; Aung et al., Citation2008). The low or undetectable levels of ELISA antibodies following live aMPV vaccination are in agreement with previous results (Eterradossi et al., Citation1995; Mekkes & de Wit, Citation1998; Van de Zande et al., Citation2000).
Inoculation of unvaccinated turkeys with a virulent aMPV-A strain caused reproducible clinical disease, histopathological lesions and aMPV-A detection in Experiments 1 and 2. Despite the presence of aMPV-B, unvaccinated turkeys in Experiment 2 were susceptible to challenge with virulent aMPV-A and developed typical TRT signs with high clinical scores. In previous studies, live aMPV-B vaccination of turkeys at 1 or 2 weeks of age was demonstrated to provide sufficient cross-protection against aMPV-A challenge (Eterradossi et al., Citation1995; Van de Zande et al., Citation2000). The younger age of the birds at the time of aMPV-B exposure and the presumably small amount of virus may explain the lack of cross-protection observed in the present study.
Untreated aMPV-A-vaccinated turkeys in all three experiments developed good protection against aMPV-A challenge infection at 2 weeks post vaccination. Protection was characterized as the absence of clinical signs and by no or only low frequencies of aMPV detection and histopathological lesions after challenge infection. In Experiment 2, the estimation of the degree of protection was difficult due to the persistence of vaccine virus and the presence of vaccine-induced clinical signs in the vaccinated groups, even after challenge. However, no differences in clinical signs, microscopic lesions and frequencies of aMPV-A detection were observed between the vaccinated challenge group (Group CVA-2) and the respective unchallenged group (Group CVC-2). These findings indicate that turkeys of Group CVA-2 have been protected against aMPV challenge.
Vaccine-induced protection of CsA-treated groups was variable in the three experiments. Group TVA-1 in Experiment 1 developed nearly full protection against challenge with only mild clinical signs transiently observed in a few birds. In Experiment 2, the CsA-treated vaccine group (Group TVA-2) also appeared to be sufficiently protected, since challenge did not exacerbate clinical disease and mucosal lesions, as compared with the respective unchallenged group (Group TVC-2). In contrast to Experiments 1 and 2, CsA-treated vaccinated birds (Group TVA-3) in Experiment 3 were fully susceptible to virulent challenge, showing the typical course of aMPV infection and disease.
The absence or the detection of only low levels of ELISA antibodies in sera and on mucosal surfaces were not indicative for susceptibility to challenge infection with virulent aMPV-A, since T-cell intact, vaccinated birds developed good protection, despite the absence of detectable ELISA-IgG in Experiments 1 and 2. This has also been observed by others during vaccination studies (Cook et al., Citation1989b; Williams et al., Citation1991; Eterradossi et al., Citation1995). On the other hand, the presence of VN antibodies in sera at the time of challenge correlated with protection in the three experiments. All groups protected against aMPV challenge possessed detectable VN antibody levels at the time of challenge, whereas the unprotected CsA-treated group in Experiment 3 (TVA-3) did not. However, high serum levels of passively transferred aMPV specific VN antibodies have been shown to be insufficient for protection against aMPV challenge infection (Rubbenstroth & Rautenschlein, Citation2009). It may be specu lated that VN serum antibodies did not provide protective effects directly, but may rather have been indicative for a protective immune response mediated by additional mechanisms.
T-lymphocytes were shown to be important for the control and clearance of aMPV infection (Rubbenstroth et al., Citation2010). They had previously been thought to provide major protection against aMPV in turkeys (Jones et al., Citation1992). Depletion of T-lymphocytes was shown to abrogate vaccine-induced protection of mice against upper respiratory tract infection with mammalian pneumoviruses, such as the human respiratory syncytial virus (Plotnicky-Gilquin et al., Citation2000). The degree of T-cell suppression was comparable between our experiments, as confirmed by reduced numbers of circulating T-lymphocytes and reduced ex vivo T-lymphocyte mitogen response in CsA-treated groups. The complete lack of vaccine-induced protection of CsA-treated turkeys in Experiment 3 (Group TVA-3) indicates that depletion of T-lymphocytes may also abrogate protection against aMPV. However, the development of protection by CsA-treated turkeys in Experiments 1 and 2 (Groups TVA-1 and TVA-2) also indicates that T-cell suppression may be overcome or be compensated by other immune mechanisms, such as innate immunity, if these mechanisms are sufficiently stimulated by the vaccine. In Experiments 1 and 2, vaccine-induced protection of CsA-treated turkeys may have been achieved because the aMPV-A vaccine replicated sufficiently for several days, as confirmed by RT-PCR detection, thus providing a continuous stimulus for the immune system. In Experiment 3 the aMPV-A vaccine was barely detectable. The insufficient stimulus of immune mechanisms may have led to vaccination failure in CsA-treated, but not in untreated turkeys.
The present study shows that a variety of factors, such as the presence of additional respiratory viruses or vaccines and T-cell competence, may influence the aMPV-vaccine induced immune response and protection in turkeys. The results emphasize the importance of immunocompetence at the time of aMPV vaccination for a predictable protective response and provide potential explanations for failures of aMPV-vaccination observed in the field. Environmental factors, stress and subclinical infections may affect T-lymphocyte functions and interfere with the aMPV-vaccine response.
Acknowledgements
The authors thank Nicole Buhr, Victoria Lebed and Christine Haase for their valuable technical assistance and Sonja Bernhardt and Martina Koschorrek for the help with the bird experiments. Sandimmun was kindly provided by Johann Gerschitz (Novartis). The project was funded by the German Research Society (DFG, RA 767/3-2).
Additional information
Notes on contributors
Dennis Rubbenstroth
Present address: Department of Virology, University of Freiburg, Hermann-Herder Str. 11, 79104 Freiburg, GermanyReferences
- Aung , Y.H. , Liman , M. , Neumann , U. and Rautenschlein , S. 2008 . Reproducibility of swollen sinuses in broilers by experimental infection with avian metapneumovirus subtypes A and B of turkey origin and their comparative pathogenesis . Avian Pathology , 37 : 65 – 74 .
- Banet-Noach , C. , Simanov , L. and Perk , S. 2005 . Characterization of Israeli avian metapneumovirus strains in turkeys and chickens . Avian Pathology , 34 : 220 – 226 .
- Banet-Noach , C. , Simanov , L. , Laham-Karam , N. , Perk , S. and Bacharach , E. 2009 . Longitudinal survey of avian metapneumoviruses in poultry in Israel: infiltration of field strains into vaccinated flocks . Avian Diseases , 53 : 184 – 189 .
- Baxter-Jones , C. , Grant , M. , Jones , R.C. and Wilding , G.P. 1989 . A comparison of three methods for detecting antibodies to turkey rhinotracheitis virus . Avian Pathology , 18 : 91 – 98 .
- Bäyon-Auboyer , M.H. , Arnauld , C. , Toquin , D. and Eterradossi , N. 2000 . Nucleotide sequences of the F, L and G protein genes of two non-A/non-B avian pneumoviruses (APV) reveal a novel APV subgroup . Journal of General Virology , 81 : 2723 – 2733 .
- Burgess , S.C. and Davison , T.F. 1999 . Counting absolute numbers of specific leukocyte subpopulations in avian whole blood using a single-step flow cytometric technique: comparison of two inbred lines of chickens . Journal of Immunological Methods , 227 : 169 – 176 .
- Catelli , E. , Cecchinato , M. , Savage , C.E. , Jones , R.C. and Naylor , C.J. 2006 . Demonstration of loss of attenuation and extended field persistence of a live avian metapneumovirus vaccine . Vaccine , 24 : 6476 – 6482 .
- Cavanagh , D. , Mawditt , K. , Britton , P. and Naylor , C.J. 1999 . Longitudinal field studies of infectious bronchitis virus and avian pneumovirus in broilers using type-specific polymerase chain reactions . Avian Pathology , 28 : 593 – 605 .
- Chacon , J.L. , Brandao , P.E. , Buim , M. , Villarreal , L. and Ferreira , A.J.P. 2007 . Detection by reverse transcriptase-polymerase chain reaction and molecular characterization of subtype B avian metapneumovirus isolated in Brazil . Avian Pathology , 36 : 383 – 387 .
- Cook , J.K.A. , Darbyshire , J.H. and Peters , R.W. 1976 . Use of chicken tracheal organ-cultures for isolation and assay of avian infectious bronchitis virus . Archives of Virology , 50 : 109 – 118 .
- Cook , J.K.A. , Ellis , M.M. and Huggins , M.B. 1991 . The pathogenesis of turkey rhinotracheitis virus in turkey poults inoculated with the virus alone or together with two strains of bacteria . Avian Pathology , 20 : 155 – 166 .
- Cook , J.K.A. , Ellis , M. M. , Dolby , C.A. , Holmes , H.C. , Finney , P.M. and Huggins , M.B. 1989a . A live attenuated turkey rhinotracheitis virus-vaccine. 1. Stability of the attenuated strain . Avian Pathology , 18 : 511 – 522 .
- Cook , J.K.A. , Holmes , H.C. , Finney , P.M. , Dolby , C.A. , Ellis , M.M. and Huggins , M.B. 1989b . A live attenuated turkey rhinotracheitis virus-vaccine. 2. The use of the attenuated strain as an experimental vaccine . Avian Pathology , 18 : 523 – 534 .
- Cook , J.K.A. , Huggins , M.B. , Orbell , S.J. and Senne , D.A. 1999 . Preliminary antigenic characterization of an avian pneumovirus isolated from commercial turkeys in Colorado, USA . Avian Pathology , 28 : 607 – 617 .
- Cook , J.K.A. , Huggins , M.B. , Orbell , S.J. , Mawditt , K. and Cavanagh , D. 2001 . Infectious bronchitis virus vaccine interferes with the replication of avian pneumovirus vaccine in domestic fowl . Avian Pathology , 30 : 233 – 242 .
- Cook , J.K.A. , Huggins , M.B. , Woods , M.A. , Orbell , S.J. and Mockett , A.P. 1995 . Protection provided by a commercially available vaccine against different strains of turkey rhinotracheitis virus . The Veterinary Record , 136 : 392 – 393 .
- Cook , J.K.A. , Jones , B.V. , Ellis , M.M. , Jing , L. and Cavanagh , D. 1993 . Antigenic differentiation of strains of turkey rhinotracheitis virus using monoclonal antibodies . Avian Pathology , 22 : 257 – 273 .
- Eterradossi , N. , Toquin , D. , Guittet , M. and Bennejean , G. 1995 . Evaluation of different turkey rhinotracheitis viruses used as antigens for serological testing following live vaccination and challenge . Journal of Veterinary Medicine—Series B , 42 : 175 – 186 .
- Fitzgerald , S.D. , Reed , W.M. , Furukawa , A.M. , Zimels , E. and Fung , L. 1995 . Effect of T-lymphocyte depletion on the pathogenesis of marble spleen disease virus-infection in ring-necked pheasants . Avian Diseases , 39 : 68 – 73 .
- Ganapathy , K. , Cox , W.J. , Gough , R.E. , Cargill , P. , Montiel , E. and Jones , R C. 2007 . Protection in specific pathogen free chickens with live avian metapneumovirus and Newcastle disease virus vaccines applied singly or in combination . Avian Pathology , 36 : 313 – 317 .
- Gough , R.E. 2003 . “ Avian pneumoviruses ” . In Diseases of Poultry , 11th edn , Edited by: Saif , Y.M. , Barnes , H. J. , Glisson , J. R. , Fadly , A. M. , McDougald , L. R. and Swayne , D. E. 92 – 99 . Ames : Iowa State Press .
- Gulati , B.R. , Patnayak , D.P. , Sheikh , A.M. , Poss , P.E. and Goyal , S.M. 2001 . Protective efficacy of high-passage avian pneumovirus (APV/MN/turkey/1-a/97) in turkeys . Avian Diseases , 45 : 593 – 597 .
- Jones , R.C. 1996 . Avian pneumovirus infection: questions still unanswered . Avian Pathology , 25 : 639 – 648 .
- Jones , R.C. , Naylor , C.J. , Alafaleq , A. , Worthington , K.J. and Jones , R. 1992 . Effect of cyclophosphamide immunosuppression on the immunity of turkeys to viral rhinotracheitis . Research in Veterinary Science , 53 : 38 – 41 .
- Juhasz , K. and Easton , A.J. 1994 . Extensive sequence variation in the attachment (G)-protein gene of avian pneumovirus—evidence for two distinct subgroups . Journal of General Virology , 75 : 2873 – 2880 .
- Khehra , R.S. and Jones , R.C. 1999 . Investigation into avian pneumovirus persistence in poults and chicks using cyclosporin A immunosuppression . Research in Veterinary Science , 66 : 161 – 163 .
- Kim , I.J. , You , S.K. , Kim , H. , Yeh , H.Y. and Sharma , J.M. 2000 . Characteristics of bursal T-lymphocytes induced by infectious bursal disease virus . Journal of Virology , 74 : 8884 – 8892 .
- Liman , M. and Rautenschlein , S. 2007 . Induction of local and systemic immune reactions following infection of turkeys with avian Metapneumovirus (aMPV) subtypes A and B . Veterinary Immunology and Immunopathology , 115 : 273 – 285 .
- Lyons , A.B. and Parish , C.R. 1994 . Determination of lymphocyte division by flowcytometry . Journal of Immunological Methods , 171 : 131 – 137 .
- Marien , M. , Decostere , A. , Martel , A. , Chiers , K. , Froyman , R. and Nauwynck , H. 2005 . Synergy between avian pneumovirus and Ornithobacterium rhinotracheale in turkeys . Avian Pathology , 34 : 204 – 211 .
- Mase , M. , Yamaguchi , S. , Tsukamoto , K. , Imada , T. , Imai , K. and Nakamura , K. 2003 . Presence of avian pneumovirus subtypes A and B in Japan . Avian Diseases , 47 : 481 – 484 .
- Mekkes , D.R. and de Wit , J.J. 1998 . Comparison of three commercial ELISA kits for the detection of turkey rhinotracheitis virus antibodies . Avian Pathology , 27 : 301 – 305 .
- Munir , S. and Kapur , V. 2003 . Regulation of host cell transcriptional physiology by the avian pneumovirus provides key insights into host–pathogen interactions . Journal of Virology , 77 : 4899 – 4910 .
- Naylor , C.J. and Jones , R.C. 1994 . Demonstration of a virulent subpopulation in a prototype live attenuated turkey rhinotracheitis vaccine . Vaccine , 12 : 1225 – 1230 .
- Naylor , C.J. , Worthington , K.J. and Jones , R.C. 1997 . Failure of maternal antibodies to protect young turkey poults against challenge with turkey rhinotracheitis virus . Avian Diseases , 41 : 968 – 971 .
- Nowak , J.S. , Kai , O. , Peck , R. and Franklin , R.M. 1982 . The effects of cyclosporin A on the chicken immune system . European Journal of Immunology , 12 : 867 – 876 .
- Owoade , A.A. , Ducatez , M.F. , Hubschen , J.M. , Sausy , A. , Chen , H. , Guan , Y. and Muller , C.P. 2008 . Avian metapneumovirus subtype A in China and subtypes A and B in Nigeria . Avian Diseases , 52 : 502 – 506 .
- Plotnicky-Gilquin , H. , Robert , A. , Chevalet , L. , Haeuw , J.F. , Beck , A. Bonnefoy , J.Y. 2000 . CD4(+) T-cell-mediated antiviral protection of the upper respiratory tract in BALB/c mice following parenteral immunization with a recombinant respiratory syncytial virus G protein fragment . Journal of Virology , 74 : 3455 – 3463 .
- Reed , L.J. and Muench , H.J. 1938 . A simple method for estimating fifty percent endpoints . American Journal of Hygiene , 27 : 493 – 497 .
- Resch , K. and Szamel , M. 1997 . Molecular mechanisms of the immunosuppressive action of cyclosporin A . International Journal of Immunopharmacology , 19 : 579 – 585 .
- Ricchizzi , E. , Catelli , E. , Cecchinato , M. , Lupini , C. , Brown , P.A. & Naylor , C.J. 2008 . Disease associated with 7 weeks old isolation of an avian Metapneumovirus vaccine derived virus . In H.M. Hafez . Proceedings of the 7th International Symposium on Turkey Diseases 171 175 . Berlin, Germany
- Rubbenstroth , D. and Rautenschlein , S. 2009 . Investigations on the protective role of passively transferred antibodies against avian metapneumovirus infection in turkeys . Avian Pathology , 38 : 427 – 436 .
- Rubbenstroth , D. , Dalgaard , T.S. , Kothlow , S. , Juul-Madsen , H.R. and Rautenschlein , S. 2010 . Effects of cyclosporin A induced T-lymphocyte depletion on the course of avian Metapneumovirus (aMPV) infection in turkeys . Developmental and Comparative Immunology , 34 : 518 – 529 .
- Rubbenstroth , D. , Ryll , M. , Behr , K.P. and Rautenschlein , S. 2009 . Pathogenesis of Riemerella anatipestifer in turkeys after experimental mono-infection via respiratory routes or dual infection together with the avian metapneumovirus . Avian Pathology , 38 : 497 – 507 .
- Schnetzler , M. , Oommen , A. , Nowak , J.S. and Franklin , R.M. 1983 . Characterization of chicken T-cell growth-factor . European Journal of Immunology , 13 : 560 – 566 .
- Schreiber , S.L. and Crabtree , G.R. 1992 . The mechanism of action of cyclosporin A and FK506 . Immunology Today , 13 : 136 – 142 .
- Suresh , M. and Sharma , J.M. 1995 . Hemorrhagic enteritis virus-induced changes in the lymphocyte subpopulations in turkeys and the effect of experimental immunodeficiency on viral pathogenesis . Veterinary Immunology and Immunopathology , 45 : 139 – 150 .
- Tarpey , I. , Huggins , M.B. and Orbell , S.J. 2007 . The efficacy of an avian metapneumovirus vaccine applied simultaneously with infectious bronchitis and Newcastle disease virus vaccines to specific-pathogen-free chickens . Avian Diseases , 51 : 594 – 596 .
- Toquin , D. , de Boisseson , C. , Beven , V. , Senne , D.A. and Eterradossi , N. 2003 . Subgroup C avian metapneumovirus (MPV) and the recently isolated human MPV exhibit a common organization but have extensive sequence divergence in their putative SH and G genes . Journal of General Virology , 84 : 2169 – 2178 .
- Van de Zande , S. , Nauwynck , H. and Pensaert , M. 2001 . The clinical, pathological and microbiological outcome of an Escherichia coli O2: K1 infection in avian pneumovirus infected turkeys . Veterinary Microbiology , 81 : 353 – 365 .
- Van de Zande , S. , Nauwynck , H. , Cavanagh , D. and Pensaert , M. 1998 . Infections and reinfections with avian pneumovirus subtype A and B on Belgian turkey farms and relation to respiratory problems . Journal of Veterinary Medicine Series B—Infectious Diseases and Veterinary Public Health , 45 : 621 – 626 .
- Van de Zande , S. , Nauwynck , H. , Naylor , C. and Pensaert , M. 2000 . Duration of cross-protection between subtypes A and B avian pneumovirus in turkeys . The Veterinary Record , 147 : 132 – 134 .
- Wilding , G.P. , Baxter-Jones , C. and Grant , M. 1986 . Ciliostatic agent found in rhinotracheitis . The Veterinary Record , 118 : 735
- Williams , R.A. , Savage , C.E. , Worthington , K.J. and Jones , R.C. 1991 . Further studies on the development of a live attenuated vaccine against turkey rhinotracheitis . Avian Pathology , 20 : 585 – 596 .
- Zenke , G. , Baumann , G. , Wenger , R. , Hiestand , P. , Quesniaux , V. , Andersen , E. and Schreier , M.H. 1993 . Molecular mechanisms of immunosuppression by cyclosporins . Annals of the New York Academy of Sciences , 685 : 330 – 335 .