Abstract
The present study determined the effect of Clostridium perfringens isolates taken from necrotic enteritis (NE) outbreaks on organic farms in a NE virulence testing model. Thirteen strains were isolated in the course of the study. Six C. perfringens field isolates were taken from a naturally occurring NE outbreak on an organic farm. Polymerase chain reaction toxinotyping was used to establish C. perfringens strains, as well as to create a toxin profile. All field isolates were found to be type A and positive for alpha, beta-2 and netB toxin genes. During the NE virulence model, digesta samples were collected before oral inoculation to define the C. perfringens found as part of the natural flora. Three of the five natural flora isolates were found to be C. perfringens type E while the other two isolates were type A; only four of five isolates were positive for either netB or beta-2 toxin genes. Two isolates collected after inoculation were C. perfringens type A positive for cpb2 and netB. All isolates were tested positive for the quorum-sensing-related gene luxS, regardless of the strain source. The presence of luxS, alpha, netB and beta-2 toxin genes seems not to be a determinant of the disease as they were present in isolates from both outbreak birds as well as healthy and pre-inoculated birds. The C. perfringens field isolates induced mild NE lesions in one-half of the birds during the challenge study. Other mechanisms must play a role in the development of the disease beyond toxinotype, potentially including intestinal ecology and health, which would account for acute disease as seen in the field outbreak.
Introduction
Environmental and dietary effects are contributing factors to Clostridium perfringens growth (Kocher, Citation2003; Williams, Citation2005). In chickens, the presence of intestinal damage produced by coccidia and using high amounts of wheat in the diet are considered predisposing factors that increase C. perfringens proliferation and the incidence of necrotic enteritis (NE), through the production of extracellular toxins that degrade host tissue (Al-Sheikhly & Al-Saieg, Citation1980; Annett et al., Citation2002). The addition of antimicrobial growth promoters (AGP) in low doses to chicken feed has been shown to increase weight gain and feed efficiency (Castanon, Citation2007). However, the use of AGP is thought to contribute to the selection of antibiotic-resistant bacteria and antibiotic residues that could contaminate the environment (Courvalin, Citation1994; Teuber, Citation1999; Witte, Citation2001). As a consequence, the European Union and other countries have prohibited the use of antibiotics as AGP in complete feeds (Grave et al., Citation2004). Nevertheless, the absence of AGP in the diets of chickens has significantly increased the incidence of NE (a re-emerging disease), has impaired feed efficiency and has doubled the use of therapeutic antibiotics causing important economic loses to farmers (Casewell et al., Citation2003; Van Immerseel et al., Citation2004; Grave et al., Citation2006). In addition, there is no information available regarding the strain characterization and their toxinotype in these new outbreaks of NE in organic production systems in order to better understand the potential mechanisms associated with the disease.
C. perfringens types A, B, C, D and E are currently recognized as the major pathogenic contributors and are associated with the production of one or more of six different major lethal toxins (Granum, Citation1990; Songer, Citation1996). Types A and C are the only types linked to the disease observed in avian species (Tschirdewahn et al., Citation1991; Cooper & Songer, Citation2009). The C. perfringens strains that infect avian species produce many extracellular toxins; however, toxins alpha (encoded by cpa), beta (cpb), beta-2 (cpb2), enterotoxin (cpe) and netB are the most significant (Engström et al., Citation2003; Martin & Smyth, Citation2009). The phospholipase C enzyme, encoded by cpa, was originally thought to be the main factor associated with pathogenesis of NE; however, recent work has indicated that cpa toxin is not an essential virulence factor in NE in chickens (Keyburn et al., Citation2006). The beta toxin is present in C. perfringens type C found in poultry (Smedly et al., Citation2004). Toxins beta, beta-2 and netB are thought to be pore-forming in nature, causing increased permeability (Shatursky et al., Citation2000; Keyburn et al., Citation2008). Toxins beta-2 and netB may be found in several bacterial isolates and have been indicated as possible factors of pathogenesis, although incidence of these genes varies (Bueschel et al., Citation2003; Keyburn et al., Citation2006). Enterotoxin is linked to certain diarrhoeas, as the major factor in food-borne contamination, and causes cell death by changing membrane permeability (Cornillot et al., Citation1995; McClane et al., Citation2000; Brynestad & Granum, Citation2002). Studies of chickens from conventional systems found that type A and type C isolates are the most common in sick birds, many of which were also found to have the cpb2 gene, although toxin expression was detected in only one-half of the birds (Crespo et al., Citation2007). Additionally, C. perfringens was also found in 10 to 15% of healthy birds (Shane et al., Citation1984; Gholamiandekhordi, et al., Citation2006). However, the expression and production of C. perfringens toxins can be associated with the quorum-sensing-related gene luxS, which can synthesize AI-2 protein. This protein facilitates pathogenic bacteria signal interactions during C. perfringens infections and induces toxin production including alpha toxin (Ohtani et al., Citation2002; Vandeville et al., Citation2005; De Keersmaecker et al., Citation2006).
To understand the pathogenesis of NE in organic systems, it is important to develop an in vivo passage model that allows consistent production of strain behaviour, clinical signs and lesions of NE. To date, consistent reproduction of the disease by oral inoculation with C. perfringens has not been successfully achieved despite the ability of bacteriocins from NE-producing strains of C. perfringens that displace non-NE strains from the gut of chicks (Olkowski et al., Citation2006; Barbara et al., Citation2008). Nevertheless, new experiments have been carried out to reproduce the infection using outbreak strains focusing more on virulence factors such as netB-producing strains (Cooper & Songer, Citation2009). The present study tested whether challenge with C. perfringens isolates from outbreaks on organic farms would reproduce the illness and examines the interaction between toxinotype and the pattern of illness observed.
Materials and Methods
Bird sample collection and culture of field isolates
Intestinal samples from 25 chickens of 25 to 32 days of age were collected from flocks experiencing NE outbreaks on two commercial farms using antibiotic-free certified feeds provided by the Organic Producers Association of Manitoba, Winnipeg, Canada. Necropsy was conducted on 25 animals that showed signs of disease including depression, ruffled feathers, and low weight gain. Two centimetres of intestinal sections from the duodenum (distal), jejunum (proximal to Meckel's diverticulum) and ileum (proximal to ileo-caecal junction) were collected for histology and fixed in 10% phosphate-buffered formalin for at least 24 h, after which they were transferred to 50% ethanol solution for 15 min followed by 70% ethanol until they were embedded in paraffin. Sections of 5 µm were cut and stained with haematoxylin and eosin. The samples were analysed by standard procedures with a light microscope emphasizing gut architecture and tissue damage. Bacteriological samples from the duodenum, jejunum and ileum were cultured on triptose soya agar with 5% sheep blood agar (Becton Dickinson, Mississauga, Canada) for 24 hours in an anaerobic environment at 35°C. Primary isolates were identified by characterizing colony morphology, Gram staining, and reverse CAMP (Hansen & Elliot, Citation1980). Isolates were preserved in preservation media (Brucella Broth; BBL, Mississauga, Canada) and 50% buffered glycerol (1 M MgSO4, 1 M Tris pH 8 and H2O), and were kept at−80°C until use.
Toxinotyping by polymerase chain reaction
Nucleic acid extraction of the intestinal lumen content was performed using an RNeasy mini kit (Qiagen, Mississauga, Canada). DNA extraction procedures were carried out according to the manufacturer guidelines. DNA aliquots were diluted 10 times to minimize polymerase chain reaction (PCR) inhibition (Vilei et al., Citation2005). All primers were obtained from the University of Calgary, Alberta, Canada (). The genotyping for C. perfringens was based on a procedure described by Garmory et al. (2000) and Keyburn et al. (Citation2008), with some modifications. Briefly, two multiplex—M1 (cpa, cpb) and M2 (etx, cpIo, cpe)—and one single PCR reaction for each netB and Cpb2 reaction were prepared using equal concentrations of primers . Each 25 µl PCR reaction contained 12.5 µl PCR Master Mix (100 µM Tris-HCl, pH 8.3 at 25°C; 500 µM KCl; 15 µM MgCl2; 0.01% (w/v) gelatine), and 5 units of Taq DNA polymerase, 4 µM dNTPs (Promega, Madison, Wisconsin, USA), 0.30 µM each cpe, cpb, cpb2, etx, cpa and cpIo oligos, and 50µM for netB oligo primers. Then 2 to 5 µl DNA template were added to each reaction. The PCRs were performed in a model 2720 Thermal Cycler (A&B Biosystem, Foster City, California, USA) using the following thermocycling programme: 95°C for 1 min, 39 cycles consisting of 95°C for 45 sec, 53°C for 3 sec, and 72°C for 1 min, and one final extension step at 72°C for 10 min. Electrophoresis was performed on a 1.5% agarose gel with ethidium bromide using standard procedures.
Table 1. PCR toxin primer sequences and products.
Capillary 16S ribosomal DNA gene sequencing comparison and phylogenetic tree
The region of 16S ribosomal DNA was amplified using standard PCR conditions. The following primers were used to run the PCR assay using the methodology described by Weisburg et al. (Citation1991): 27f 5′-AGAGTTTGATCMTGGCTCAG-3′ and 1100 r 5′-GGGTTGCGCTCGTTG-3′. Forward primers were fluorescently labelled (WellRED D4dye; Sigma-Proligo, St Louis, Missouri, USA) to allow detection of the fragments by capillary electrophoresis. The PCR reaction contained 12.5 µl PCR Master Mix (Promega), 5 µM each primer and DNA template (0.2 µg) for a total volume of 20 µl. Samples were run in a preheated thermal cycler for 35 cycles with: 30 sec at 94oC, 30 sec at 54oC, 30 sec at 72oC. Then 10 µl DNA amplicons were analysed on a 1.5% agarose gel. PCR band profiles were verified by 16S rDNA gene sequence determination and phenotypic identification. The 16S rDNA sequencing of C. perfringens was performed at the University of Calgary, University Core DNA Services using Applied Biosystems 3730 and 3730xl DNA analysers. The isolates collected in this experiment were compared with the whole C. perfringens genome sequences found in the NCBI database.
Sequence alignment was performed using a BioEdit Sequence Alignment Editor (Hall, Citation1999) and tree visualization was generated by UPGMA algorithm using MEGA version 4 (Tamura et al., Citation2007). The three isolate sources included NE-affected birds from field outbreaks (wild type), healthy birds prior to NE virulence testing model inoculation of C. perfringens (natural flora) and chickens displaying mild or no signs of disease after inoculation of C. perfringens (post challenge).
C. perfringens isolation from NE virulence testing model
The ability of the C. perfringens field outbreak isolates to induce NE was evaluated by an NE virulence testing model using chickens fed organic feed. Two hundred and fifty Ross broilers were raised experimentally to mimic the conditions of the natural outbreak. The birds were floor-raised in groups of 25 per pen and fed an antibiotic-free certified organic starter diet containing 23% crude protein and 50% wheat. At day 14, birds were fed a certified organic grower diet containing 20% crude protein and 60% wheat. Both diets were purchased from the manufacturer who supplied feed during the field outbreak. On day 1 all the animals were vaccinated against coccidia, using an oral coccidiosis vaccine (Immucox®, Guelph, Canada). The vaccine pucks were evenly split at 1 puck for every 100 birds. Two representative field outbreak isolates of C. perfringens were initially grown in 5 ml Schaedler's anaerobic broth (Oxoid®, Missisauga, Canada) at 37°C in anaerobic conditions for use in the challenge study. The amount of media was increased over the course of 8 days and cultures were split to allow for growth until sufficient amounts of bacteria were obtained (Olkowski et al., Citation2006). Using an oesophageal cannula, birds were orally challenged on day 14 with 1 ml C. perfringens culture at 3x1010 colony-forming units/bird. Five birds from each pen were euthanized at day 11 (pre challenge) to determine normal flora; the remaining birds were euthanized at day 22 (post challenge) in accordance with the Animal Ethics Use Protocols of the University of Manitoba approved by the Animal Ethics Committee. Approximately 10 g ileal and caecal digesta were collected and mixed, and the isolation of C. perfringens was carried out as described previously.
Results
Post-mortem, histology and sample culture analysis
Outbreaks. To determine the characteristic lesions of natural cases of NE, pathology was performed on animals that, at the time of necropsy, displayed depression, ruffled feathers, inappetence and dark diarrhoea. Macroscopically, necropsy of the affected birds revealed dehydration, small intestine thickened and ballooned with gas, intestinal mucosa with diphtheritic membrane, and necrotic areas in the distal intestine with thickened intestinal walls associated with the “Turkish towel” effect typical of NE (a). Histopathology of the duodenal and jejunal sections showed necrosis of villous tips or entire villi, down to the crypts, with many large bacilli in this necrotic and cellular debris (b). Also, there were various coccidial stages including oocysts throughout the cellular debris and enterocytes of the viable tissue. Parasitological and bacteriological findings indicated high levels of coccidia and C. perfringens that correlated with the histological analysis, which led to a final diagnosis of coccidiosis and NE.
Figure 1. Macroscopic and microscopic lesions of natural cases of NE. 1a: Small intestine thickened and ballooned with gas, intestinal mucosa with diphtheritic membrane, and necrotic areas in the distal Intestine with thickened intestinal walls associated with the “Turkish towel” effect typical of NE. 1b: Intestinal section showing villi necrosis, with many large bacilli lining the necrotic area and cellular debris.
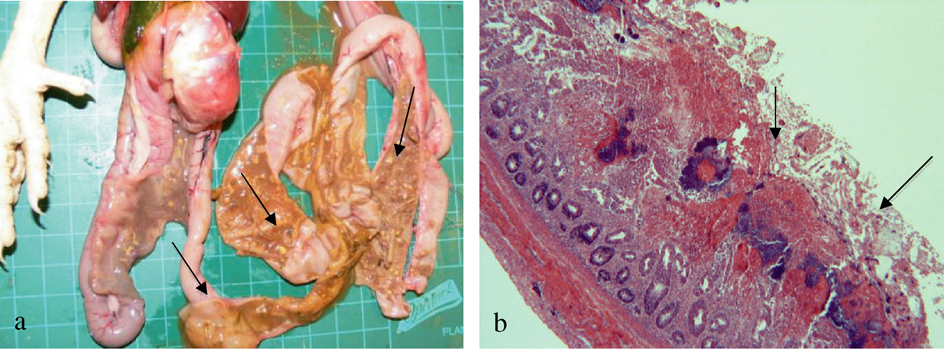
Necrotic enteritis virulence testing model. Clinical signs were mild in all groups and were characterized by depression, ruffled feathers, and inappetence. Necropsy revealed distended intestine, oedema and enteritis areas in the middle and distal small intestine; there was no evidence of macroscopic changes associated with thickness or intestinal tissue damage. Histopathological analysis showed extensive oedema, lymphocytic infiltration, mild villi necrosis and various coccidia stages in the enterocytes in all intestinal sections.
Genotype, toxinotype and phylogenetic tree
Phylogenetic analysis of cultures. The majority of the 13 isolates showed a high level (97 to 100%) of similarity with two of the three whole-genome sequences of C. perfringens found in the gene bank (GenBank/DDBJ/EMBL): strains SM101 and American Type Culture Collection ATCC 13124 (accession numbers NC 008262 and NC 008216, respectively) () . In order to obtain highly reliable results in the phylogenetic analysis, the sequences from the whole-genome project were also added in the analysis, including strains ATCC 3626, JGS1495, JGS1721, JGS1987, National Collection of Type Cultures (NCTC) 8239 and strain F4969 (incomplete sequence data).
Figure 2. Phylogenetic analysis of C. perfringens isolates of 16S rDNA sequences. Level of similarity relation between C. Perfringens isolates from outbreaks, normal flora and post challenge, and three sequences of C. perfringens deposited in the gene bank are shown. FI, field isolate; NF, normal flora; PC, post challenge; all others refer to strains from the NCBI database.
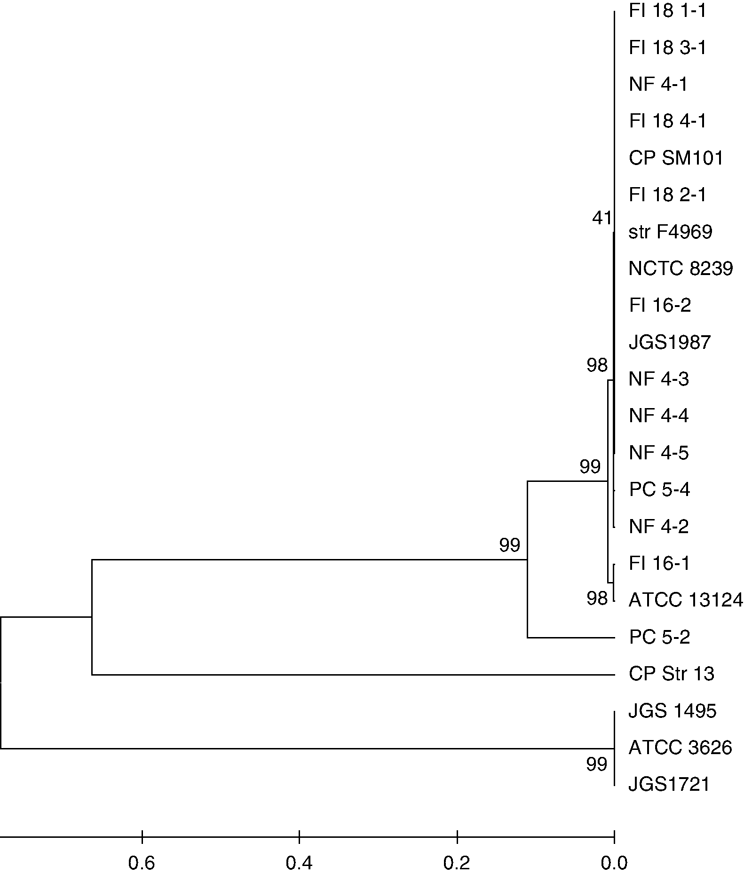
Whole-genome sequence SM101 was highly related to all natural flora isolates, five of six field isolates and one of two post-challenge isolates. As well, unfinished whole-genome sequences of strain F4969, NCTC 8239 and JGS1987 were highly related. The data were supported by bootstrap analysis showing values higher than 60 (). Field Isolate 16-1 was highly related to ATCC 13124 (bootstrap value of 98). It was the only isolate found within this group. Post-challenge isolate 5-2 stood alone as it was not as highly related to any of the other trial isolates, although its lineage relates to strain 13.
C. perfringens toxinotyping. All field isolates were positive for cpa, cpb2, netB and luxS (). Normal flora isolates (collected before inoculation in the NE virulence testing model) were all positive for cpa and showed varying degrees of positive reaction for cpb2, cpIo and netB. Over one-half of the normal flora isolates were C. perfringens type A; three isolates found to contain cpIo belonged to C. perfringens type E. Isolates collected after the NE virulence testing model were all positive for cpa, although only some showed positive results for cpb2 and netB. The luxS gene was positive for all the strains regardless of their source.
Table 2. Summary of the C. perfringens PCR toxinotyping profile according different sources and correlation with clinical status.
Discussion
In the present study, the genotype of NE-producing strains of C. perfringens from an organic production system was determined. Also, the capacity of these strains to induce and reproduce NE was examined using a virulence test model.
C. perfringens SM101, strain F4969 and NCTC 8239 are all type A bacteria known to carry the cpe gene. Type E JGS1987 is also related to this large group. Isolates NF4-3 and 4-4 are type E based on toxinotype and were found to have a similar lineage as JGS1987, although type A isolate FI16-2 was found to be more directly related. SM101 was the only completed whole genome in this group, a type A originating from a human food-poisoning isolate (Myers et al., Citation2006). SM101 did not consistently cause disease under experimental conditions, which could explain its relation to all five natural flora isolates that did not have disease-causing effects (Myers et al., Citation2006). Five of six field isolates were also found in this group, all of which are type A, like SM101. None of the experimental isolates in this group were positive for cpe, which may be found in SM101 strains associated with food poisoning (Myers et al., Citation2006).
Field isolate 16-1 belongs to whole-genome sequence ATCC 13124, taken from human gas gangrene and having great disease-causing potential (Myers et al., Citation2006). Both described are type A C. perfringens and neither were shown to carry cpe genes. Despite similarities in genotype, FI16-1 was divergent from related field isolates found in the SM101 groups. It seems that this infection was produced by a bacterial population unique in comparison with others collected.
Isolates NF 4-4 and PC 5-4 were taken from the same pen and were highly related. Isolate PC 5-2 does not have a corresponding NF isolate as no C. perfringens could be cultured. It could be that challenge boosted C. perfringens levels to where they were detectable; oddly, the isolate was not highly related to that which was used to inoculate the birds but appears to be an original strain most highly related to strain 13, a soil-borne bacterium with disease-causing potential. This strain was most probably already present at the time of challenge or was introduced soon after (Shimizu et al., Citation2002). JGS 1496, ATCC 3626 and JGS 1721 strains are all whole genome sequences currently available and are the divergent group, a logical outcome as they are C. perfringens types not found in chickens or within the isolates studied in this research.
C. perfringens type A commonly occurs in North America and is one of two types associated with NE (Songer, Citation2006). Alpha toxin was found in all bacterial cultures from the field isolates, normal flora and post-challenge isolates. In the present study, alpha toxin was recurrently found to be the only major toxin pointing to C. perfringens type A as the main type noted, apart from a few exceptions that are described later in this manuscript.
An interesting finding was that cpIo was detected at low levels in normal flora isolates. To our knowledge, this is the first report indicating the presence of C. perfringens type E in poultry. The finding of type E in the presumed normal flora could explain the lack of disease found in these three groups pre-challenge. It should also be noted that the room used for experimentation was previously used to house bovine species that could carry type E C. perfringens (Itodo et al., Citation1986). Toxin gene cpIo was not found in the field or post-challenge isolates.
Toxin genes for cpb, cpe, and etx were absent in all field strains, normal flora and post-challenge isolates. Toxins cpb or etx are associated with C. perfringens types other than type A and would have changed the toxinotype noted. Toxin gene cpe is known to cause human food poisoning and may be found in type A and type C C. perfringens but is not commonly seen in poultry (Tschirdewahn et al., Citation1991; Songer, Citation1996; Gholamiandekhordi et al., 2006).
It has been suggested that potentially virulent toxins cpb2 and netB could contribute to disease effects in field isolates (Bueschel et al., Citation2003; Keyburn et al., Citation2008). In the present work, these genes were also found in varying degrees in normal flora and post-inoculation isolates. However, no obvious NE was noted in the birds used for normal flora samples despite the presence of at least one of these toxin genes in all of the isolates. Only one of two birds in the post-inoculation group showed signs of enteritis, one of them having cpb2 and low levels of netB expression. The evidence of the level of virulence of these toxin genes remains inconclusive. Our results agree with those of previous studies, which suggested that cpb2 and netB did not necessarily have a direct correlation with the incidence of NE (Gholamiandekhordi et al., Citation2006; Martin & Smyth, Citation2009). Positive PCR results for both netB and cpb2 toxin genes in the field isolates could play a role in pathogenesis and may have enhanced necrosis in the intestine. However, some studies have shown that beta-2 toxin may not be significantly associated with NE (Bueschel et al., Citation2003; Gholamiandekhordi et al., Citation2006; Keyburn et al., Citation2008). It should be noted that the occurrence of a gene does not necessarily determine phenotype of toxin production, which may be a stronger determinant of virulence (Abildgaard et al., Citation2010).
Positive findings for luxS were expected based on the level of conservation of this gene between bacterial types (Brassler, Citation1999). As a quorum-sense-related gene, luxS is reliant on predisposing factors to increase colonization by causing a shift in the gut ecology and bacterial numbers before allowing toxin production. In the case of alpha toxin it was found that luxS had only a slight stimulatory effect and was not completely dependent on luxS for toxin production (Ohtani et al., Citation2002). However, in our results using NE-producing strains there was no difference between luxS findings in normal flora versus NE-producing strains.
In the field cases, classic signs of NE typically seen upon necropsy included the well-characterized “Turkish towel” effect and focal necrosis in the middle and distal small intestine (Broussard et al., Citation1986; Kaldhusdal & Hofshagen, Citation1992). The isolation of both coccidia and C. perfringens confirmed a simultaneous infection that implies coccidiosis is a common precursor to NE, suggesting that similar origins are seen in organic and conventional systems (Al-Sheikhly & Al-Saieg, Citation1980).
Experimental reproduction of acute NE has proven difficult in many cases (Olkowski et al., Citation2006; Thompson et al., Citation2006; Gholamiandehkordi et al., Citation2007). It has been shown that in vitro passage can reduce virulence of a strain due to genetic modification (Chalmers et al., Citation2007). Predisposing factors associated with NE have been used in challenges to increase the potential for disease replication with varying results (Olkowski et al., Citation2006; Chalmers et al., Citation2007; Gholamiandehkordi et al., Citation2007). For example, mucosal damage, caused by the coccidia vaccine, could affect intestinal microbiotic features triggering endogenous virulence factors that facilitate NE (Gholamiandehkordi et al., Citation2007). The outcome of the NE virulence testing model in this experiment had similar outcomes to that seen by Thompson et al. (Citation2006), where the inoculation isolate was taken recently from an outbreak and went through minimal in vitro passage yet did not accomplish an acute case of NE. This study differed from others where NE was achieved in that the birds were given liquid inoculations rather than cultures mixed with feed (Chalmers et al., Citation2007; Cooper & Songer, Citation2010).
The presence of large numbers of bacteria in the necrotic debris of the mucosa, which is a characteristic of NE, was similar to what has been shown before (Olkowski et al., Citation2006). Therefore, our model should be considered successful.
In conclusion, the C. perfringens strains isolated from organic farm outbreaks, normal flora and birds challenged with NE-producing strains were found to be similar to those found in conventional systems based on toxinotype characterization and phylogeny analysis. Based on these findings it seems that the effect of a NE outbreak is equally as challenging in organic as in conventional systems with an added risk due to the absence of prophylactic measures. Therefore, more research into non-antibiotic prophylactic strategies may be beneficial to reduce the potential for NE outbreaks. In addition, further analysis is needed to establish a much better correlation between gene expression of toxin and the pathogenic capacity of C. perfringens strains from organic systems.
Acknowledgements
The excellent technical assistance of Dr Tom Hutchison and Chris Link-Muirhead from Manitoba Agriculture, Food and Rural Initiatives, Veterinary Diagnostic Services (MAFRI) is greatly appreciated. The authors would like to thank Andre Hamel (MAFRI) for providing the control PCR C. perfringens strains, Angela Kroeker for incredible technical support, and Omar Abu-Dahab for providing the certified organic feed. The authors would like to express appreciation to the Agricultural and Rural Development Initiative (ARDI) for the ?nancial support from a scholarship for the ?rst author. Financial support from the Manitoba Egg Farmers for poultry infrastructure resources is gratefully appreciated.
References
- Abildgaard , L. , Sondergaard , T.E. , Engberg , R.M. , Schramm , A. and Højberg , O. 2010 . In vitro production of necrotic enteritis toxin B, NetB, by netB-positive and netB-negative Clostridium perfringens originating from healthy and diseased broiler chickens . Veterinary Microbiology , 144 : 231 – 235 .
- Al-Sheikhly , F. and Al-Saieg , A. 1980 . Role of coccidia in the occurrence of necrotic enteritis of chickens . Avian Diseases , 24 : 324 – 333 .
- Annett , C. , Viste , J. , Chrinino-Trejo , M. , Classen , H. , Middleton , D. and Simko , E. 2002 . Necrotic enteritis: effect of barley, wheat and corn diets on proliferation of Clostridium perfringens type A . Avian Pathology , 31 : 598 – 601 .
- Barbara , A.J. , Trinh , H.T. , Glock , R.D. and Songer , J.G. 2008 . Necrotic enteritis-producing strains of Clostridium perfringens displace non-necrotic enteritis strains from the gut of chicks . Veterinary Microbiology , 126 : 377 – 382 .
- Brassler , B.L. 1999 . How bacteria talk to each other: regulation of gene expression by quorum sensing . Current Opinion in Microbiology , 2 : 582 – 587 .
- Broussard , C.T. , Hofacre , C.L. , Page , R.K. and Fletcher , O.J. 1986 . Necrotic enteritis in cage-reared commercial layer pullets . Avian Diseases , 30 : 617 – 619 .
- Brynestad , S. and Granum , P.E. 2002 . Clostridium perfringens and foodborne infections . International Journal of Food Microbiology , 74 : 195 – 202 .
- Bueschel , D.M. , Jost , B.H. , Billington , S.J. , Trinh , H.T. and Songer , J.G. 2003 . Prevalence of cpb2, encoding β2 toxin, in Clostridium perfringens field isolates correlation of genotype with phenotype . Veterinary Microbiology , 94 : 121 – 129 .
- Casewell , M. , Friis , C. , Marco , E. , McMullin , P. and Phillips , I. 2003 . The European ban on growth-promoting antibiotics and emerging consequences for human and animal health . Journal of Antimicrobial Chemotherapy , 52 : 159 – 161 .
- Castanon , J.I.R. 2007 . History of the use of antibiotic as growth promoters in European poultry feeds . Poultry Science , 86 : 2466 – 2471 .
- Chalmers , G. , Bruce , H.L. , Toole , D.L. , Barnum , D. and Boerlin , P. 2007 . Necrotic enteritis potential in a model system using Clostridium perfringens isolated from field outbreaks . Avian Diseases , 51 : 834 – 839 .
- Cornillot , E. , Saint-Joanis , B. , Daube , G. , Katayama , S. , Granum , P.E. , Canard , B. and Cole , S.T. 1995 . The enterotoxin gene (cpe) of Clostridium perfringens can be chromosomal or plasmid-borne . Molecular Microbiology , 15 : 639 – 647 .
- Cooper , K.K. and Songer , J.G. 2009 . Necrotic enteritis in chickens: a paradigm of enteric infection by Clostridium perfringens type A . Anaerobe , 15 : 55 – 60 .
- Cooper , K.K. and Songer , J.G. 2010 . Virulence of Clostridium perfringens in an experimental model of poultry necrotic enteritis . Veterinary Microbiology , 142 : 323 – 328 .
- Courvalin , P. 1994 . Transfer of antibiotic resistance genes between Gram-positive and Gram-negative bacteria . Antimicrobial Agents and Chemotherapy , 38 : 1447 – 1451 .
- Crespo , R. , Fisher , D.J. , Shivaprasad , H.L. , Fernandez-Miyakawa , M.E. and Uzal , F.A. 2007 . Toxinotypes of Clostridium perfringens isolated from sick and healthy avian species . Journal of Veterinary Diagnostic Investigation , 19 : 329 – 333 .
- De Keersmaecker , S.C. , Sonck , K. and Vanderleyden , J. 2006 . Let LuxS speak up in AI-2 signaling . Trends in Microbiology , 14 : 114 – 119 .
- Engström , B.E. , Fermér , C. , Lindberg , A. , Saarinen , E. , Bayerud , A. and Gunnarsson , A. 2003 . Molecular typing of isolates of Clostridium perfringens from healthy and diseased poultry . Veterinary Microbiology , 94 : 225 – 235 .
- Garmony , H.S. , Chanter , N. , French , N.P. , Bueschel , D. , Songer , J.G. and Titball , R.W. 2000 . Occurrence of Clostridium perfringens β2-toxin amongst animals determined using genotyping and subtyping PCR assays . Epidemiology and Infection , 124 : 61 – 67 .
- Gholamiandekhordi , A.R. , Ducatelle , R. , Heyndrickx , M. , Haesebrouck , F. and Van Immerseel , F. 2006 . Molecular and phenotypical characterization of Clostridium perfringens isolates from poultry flocks with different disease status . Veterinary Microbiology , 113 : 143 – 152 .
- Gholamiandehkordi , A.R. , Timbermont , L. , Lanckriet , A. , Van Den Broeck , W. , Pedersen , K. Dewulf , J. 2007 . Quantification of gut lesions in a subclinical necrotic enteritis model . Avian Pathology , 36 : 375 – 382 .
- Granum , P.E. 1990 . Clostridium perfringens toxins involved in food poisoning . International Journal of Food Microbiology , 10 : 101 – 112 .
- Grave , K. , Jensen , V.F. , Odensvik , K. , Wierup , M. and Bangen , M. 2006 . Use of veterinary therapeutic antimicrobials in Denmark, Norway and Sweden following termination of antimicrobial growth promoter use . Preventive Veterinary Medicine , 75 : 123 – 132 .
- Grave , K. , Kaldhusdal , M. , Kruse , H. and Flatlandsmo , K. 2004 . What has happened in Norway after the ban of avoparcin? Consumption of antimicrobials by poultry . Preventive Veterinary Medicine , 62 : 59 – 72 .
- Hall T.A. 1999 BioEdit: a user-friendly biological sequence alignment editor and analysis program for Windows 95/98/NT Nucleic Acids Symposium Series 41 95 98
- Hansen , M.V. and Elliott , L.P. 1980 . New presumptive identification test for Clostridium perfringens: reverse CAMP test . Journal of Clinical Microbiology , 12 : 617 – 619 .
- Itodo , A.E. , Adesiyun , A.A. , Adekeye , J.O. and Umoh , J.U. 1986 . Toxin-types of Clostridium perfringens strains isolated from sheep, cattle and paddock soils in Nigeria . Veterinary Microbiology , 12 : 93 – 96 .
- Kaldhusdal M. Hofshagen M. 1992 Barley inclusion and avoparcin supplementation in broiler diets. 2. Clinical, pathological, and bacteriological findings in a mild form of necrotic enteritis Poultry Science 71 1145 1153
- Keyburn A.L. Boyce J.D. Vaz P. Bannam T. Ford M. Parker D. et al. 2008 NetB, a new toxin that is associated with avian necrotic enteritis caused by Clostridium perfringens PLoS Pathogens 4 e26
- Keyburn , A.L. , Sheedy , S.A. , Ford , M.E. , Williamson , M.M. , Awad , M.M. , Rood , J.I. and Moore , R.J. 2006 . The alpha-toxin of Clostridium perfringens is not an essential virulence factor in necrotic enteritis in chickens . Infection and Immunity , 74 : 6496 – 6500 .
- Kocher , A. 2003 . Nutritional manipulation of necrotic enteritis outbreak in broilers . Recent Advances in Animal Nutrition in Australia , 14 : 111 – 116 .
- Martin , T.G. and Smyth , J.A. 2009 . Prevalence of netB among some clinical isolates of Clostridium perfringens from animals in the United States . Veterinary Microbiology , 136 : 202 – 205 .
- McClane , B.A. , Lyerly , D. , Scott Moncrief , J.J. and Wilkins , T.D. 2000 . “ Enterotoxic Clostridia: Clostridium perfringens Type A and Clostridum difficile ” . In Gram-Positive Pathogens , 1st edn , Edited by: Fischetti , V.A. , Novick , R. , Ferretti , J.J. , Portnoy , D.A. and Rood , J.I. 551 – 562 . Washington , DC : ASM Press .
- Myers , G.S. , Rasko , D.A. , Cheung , J.K. , Ravel , J. , Seshadri , R. DeBoy , R.T. 2006 . Skewed genomic variability in strains of the toxigenic bacterial pathogen, Clostridium perfringens . Genome Research , 16 : 1031 – 1040 .
- Ohtani , K. , Hayashi , H. and Shimizu , T. 2002 . The luxS gene is involved in cell-cell signalling for toxin production in Clostridium perfringens . Molecular Microbiology , 44 : 171 – 179 .
- Olkowski , A.A. , Wojnarowicz , C. , Chirino-Trejo , M. and Drew , M.D. 2006 . Responses of broiler chickens orally challenged with Clostridium perfringens isolated from field cases of necrotic enteritis . Research in Veterinary Science , 81 : 99 – 108 .
- Shane , S.M. , Koetting , D.G. and Harrington , K.S. 1984 . The occurrence of Clostridium perfringens in the intestine of chicks . Avian Diseases , 28 : 1120 – 1224 .
- Shatursky O. Bayles R. Rogers, M Jost B.H. JG Songer J.G. Tweten R.K. 2000 Clostridium perfringens beta-toxin forms potential-dependant, cation-selective channels in lipid bilayers Infection and Immunity 68 5546 5551
- Shimizu , T. , Ohtani , K. , Hirakawa , H. , Ohshima , K. , Yamashita , A. Shiba , T. 2002 . Complete genome sequence of Clostridium perfringens, an anaerobic flesh-eater . Proceeding of the National Academy of Sciences USA , 99 : 996 – 1001 .
- Smedley III J.G. Fisher D.J. Sayeed S. Chakrabarti G. McClane B.A. 2004 The enteric toxins of Clostridium perfringens. Reviews of Physiology, Biochemistry and Pharmacology 152 182 204
- Songer , J.G. 1996 . Clostridial enteric diseases of domestic animals . Clinical Microbiological Reviews , 9 : 216 – 234 .
- Songer , J.G. 2006 . “ Clostridial abomasitis ” . In Genus Clostridium. Clostridia in Medical Veterinary and Food Microbiology. Diagnosis and Typing , Edited by: Mainil , J. , Duchesnes , C. , Granum , P.E. , Menozzi , M.G. , Peck , M. , Pelkonen , S. , Popoff , M. , Stackebrandt , E. and Titball , R. 44 – 50 . Brussels : European Concerted Action QLK2-CT2001- 01267 .
- Tamura , K. , Dudley , J. , Nei , M. and Kumar , S. 2007 . MEGA4: molecular evolutionary genetics analysis (MEGA) software version 4.0 . Molecular Biology and Evolution , 24 : 1596 – 1599 .
- Teuber , M. 1999 . Spread of antibiotic resistance with food-borne pathogens . Cellular and Molecular Life Sciences , 56 : 755 – 763 .
- Thompson , D.R. , Parreira , V.R. , Kulkarni , R.R. and Prescott , J.F. 2006 . Live attenuated vaccine-based control of necrotic enteritis of broiler chickens . Veterinary Microbiology , 113 : 25 – 34 .
- Tschirdewahn , B. , Notermans , S. , Wernars , K. and Untermann , F. 1991 . The presence of enterotoxigenic Clostridium perfringens in faeces of various animals . International Journal of Food Microbiology , 14 : 175 – 178 .
- Vendeville A. Winzer K. Heurlier K. Tang C.M. Hardie K.R. 2005 Making “sense” of metabolism: autoinducer-2, Luxs and pathogenic bacteria Nature Reviews Microbiology 3 383 396
- Van Immerseel , F.V. , De Buck , J. , Pasmans , F. , Huyghebaert , G. , Hasesebrouck , F. and Ducatelle , R. 2004 . Clostridium perfringens in poultry: an emerging threat for animal and public health . Avian Pathology , 33 : 537 – 549 .
- Vilei , E.M. , Schlatter , Y. , Perreten , V. , Straub , R. , Popoff , M.R. Gibert , M. 2005 . Antibiotic-induced expression of a cryptic cpb2 gene in equine beta2-toxigenic Clostridium perfringens . Molecular Microbiology , 57 : 1570 – 1581 .
- Weisburg , W.G. , Barns , S.M. , Pelletier , D.A. and Lane , D.J. 1991 . 16S ribosomal DNA amplification for phylogenetic study . Journal of Bacteriology , 173 : 697 – 703 .
- Williams , R.B. 2005 . Intercurrent coccidiosis and necrotic enteritis of chickens: rational, integrated disease management by maintenance of gut integrity . Avian Pathology , 34 : 159 – 180 .
- Witte , W. 2001 . Selective pressure by antibiotics in livestock . International Journal of Antimicrobial Agent , 16 : 19 – 24 .