Abstract
Idiopathic pulmonary arterial hypertension (IPAH) is a disease of unknown cause that is characterized by elevated pulmonary arterial pressure and pulmonary vascular resistance, and by extensive vascular remodelling. In human IPAH patients, remodelling of the pulmonary vasculature results in the formation of plexiform lesions in the terminal pulmonary arterioles. Various molecules are expressed in the human plexiform lesions, including alpha smooth muscle actin, von Willebrand factor, vascular endothelial growth factor, vascular endothelial growth factor receptor type 2, hypoxia inducible factor-1α, survivin, tenascin, collagen, fibronectin, and various immune/inflammatory cells such as, cytotoxic lymphocytes, B lymphocytes, MHC class II cells, and monocytes/macrophages are also present. Plexiform lesions rarely develop in the lungs of laboratory animals, but plexiform-like complex vascular lesions (CVL) do develop spontaneously in the lungs of broiler chickens from an IPAH-susceptible line. To examine angioproliferative and immune-system-related activities associated with CVL in broiler lungs, paraformaldehyde-fixed, paraffin-embedded lung sections from 8-week-old to 24-week-old broiler chickens were stained immunohistochemically using monoclonal or polyclonal antibodies specific for angioproliferative molecules and immune/inflammatory cells. The CVL in the lungs of broiler chickens exhibited positive staining for both angioproliferative molecules and immune/inflammatory cells. These observations combined with the close histological resemblance of broiler CVL to the plexiform lesions of human IPAH patients further validates chickens from our IPAH-susceptible line as an excellent animal model of spontaneous plexogenic arteriopathy.
Introduction
Idiopathic pulmonary arterial hypertension (IPAH) is a disease of unknown cause that is characterized by elevated pulmonary arterial pressure and pulmonary vascular resistance, and by extensive remodelling of the pulmonary vasculature. In human patients, the vascular remodelling includes intimal hyperplasia along with medial and adventitial hypertrophy and hyperplasia in the large and intermediate-sized pulmonary arteries and arterioles (Tuder et al., Citation2009). Cells such as smooth muscle cells, endothelial cells, and fibroblasts originating within the pulmonary artery/arterioles interact with cells from the circulation to form complex vascular lesions (CVL), known as plexiform lesions (Taraseviciene-Stewart et al., Citation2001; Xu et al., Citation2007; Masri et al., Citation2007). Most plexiform lesions are formed near branch points of muscular arteries (Cool et al., Citation1999) and are composed of a network of vascular channels that penetrate a core of myofibroblastic or less well differentiated cells (Heath et al., Citation1988; Tuder et al., Citation1994).
Various angioproliferative molecules are expressed in plexiform lesions, some of which include von Willebrand factor (vWF), alpha smooth muscle actin (αSMA) (Hirose et al., Citation2000), vascular endothelial growth factor (VEGF), VEGF receptor type 2 (VEGFR-2), hypoxia inducible factor-1α (HIF-1α) (Tuder et al., Citation2001), survivin (Cool et al., Citation2005), collagen (Botney et al., Citation1993; Morrell et al., Citation2009), fibronectin (Mitani et al., Citation2001), and tenascin (Jones & Rabinovitch, Citation1996). Expression of these molecules in plexiform lesions of IPAH patients contributes to our understanding of the pathophysiological events leading to the formation of plexiform lesions. vWF is synthesized mainly in endothelial cells and plays a crucial role in platelet adhesion and aggregation at the site of vascular injury (Veyradier et al., Citation2000). Higher levels of vWF detected in arterial and venous blood from IPAH patients reflect increased endothelial cell proliferation (Collados et al., Citation1999). Expression of αSMA is well known as the earliest indicator of the developing smooth muscle cell phenotype (Owens, Citation1995), and αSMA is expressed abundantly in plexiform lesions (Tuder et al., Citation2007). vWF and αSMA are considered the most common markers to be expressed in plexiform lesions in lungs of IPAH patients, and their overexpression definitively signals the involvement of endothelial cells and smooth muscle cells and their proliferation during the pathogenesis of plexogenic arteriopathy. VEGF is an endothelial cell-specific mitogen and is essential for the survival of the endothelial cells (Hirose et al., Citation2000). VEGF elicits cellular responses by binding with its two receptors known as VEGF receptor type 1 and VEGFR-2, of which VEFGR-2 mediates the majority of the cellular response (Holmes et al., Citation2007). VEGFR-2 also plays a critical role in the survival of endothelial cells (Gerber et al., Citation1998). Expression of both VEGF and VEGFR-2 was detected in plexiform lesions in the lungs of pulmonary arterial hypertension (PAH) patients (Tuder et al., Citation2001). HIF is a heterodimeric transcription factor consisting of an inducible alpha subunit (HIF-1α) and a constitutive beta subunit (HIF-1β) (Wang et al., Citation1995). HIF-1α plays an important role in activating the VEGF gene in response to hypoxia and also is expressed abundantly in human plexiform lesions (Tuder et al., Citation2001). Survivin is an anti-apoptotic molecule and is overexpressed in the pulmonary arterioles from PAH patients when compared with control subjects (McMurtry et al., Citation2005). Survivin promotes endothelial cell and smooth muscle cell proliferation and also decreases vascular cell apoptosis (McMurtry et al., Citation2005; Bonnet et al., Citation2006). Tenascin is an extracellular matrix glycoprotein and induces proliferation and survival of pulmonary arterial smooth muscle cells (PASMC) (Jones & Rabinovitch, Citation1996). Fibronectin is also an extracellular matrix glycoprotein, which is expressed in the pulmonary arterioles of PAH patients and is responsible for smooth muscle cell proliferation (Clausell & Rabinovitch, Citation1993; Jones et al., Citation1997). Collagen is a protein present in the muscle cells and connective tissue, and also is one of the components of extracellular matrix. Collagen is synthesized actively in the pulmonary arterioles of IPAH patients, and thickening of the adventitia of pulmonary arteriole is attributed to the increased synthesis of collagen (Meyrick & Reid, Citation1980; Botney et al., Citation1993). Various components of extracellular matrix, including collagen, tenascin, and fibronectin, were expressed in the plexiform lesions of the lungs of PAH patients, suggesting the active involvement of these extracellular matrix components in the formation of plexiform lesions (Tuder et al., Citation2007).
In addition to the angioproliferative molecules, the plexiform lesions in the lungs from PAH patients have been shown to be infiltrated with various immune and inflammatory cells such as B cells, T cells, and macrophages (Tuder et al., Citation1994). In another study, Cool et al. (Citation1997) also demonstrated that the macrophages and T cells were clustered around the plexiform lesions. The presence of macrophages and perivascular cell infiltrates composed of T lymphocytes and B lymphocytes suggests that the inflammation/immune system may play a role in the pathogenesis of PAH (Dorfmüller et al., Citation2003). Some PAH patients have elevated circulating levels and pulmonary expression of various inflammatory cytokines and chemokines, including interleukin (IL)-1β, IL-6, monocyte chemoattractant protein-1, and RANTES (regulated upon activation, normal T-cell-expressed and secreted) (Humbert et al., Citation1995; Dorfmüller et al., Citation2002; Itoh et al., Citation2006). It has been postulated that the release of these cytokines, chemokines, and growth factors by inflammatory cells may contribute to the proliferation and migration of PASMC (Tajsic & Morrell, Citation2011).
Comprehensive understanding of the pathogenesis of plexiform lesion development in lungs of IPAH patients has been hampered due to the lack of good animal models in which plexiform lesions develop spontaneously (Wideman & Hamal, Citation2011). Chickens bred for rapid growth and meat production (broiler chickens) provide an excellent model of spontaneous IPAH encompassing many of the classical signs and the genetically tractable system for elucidating the underlying causes. Approximately 3% of all broiler chickens develop IPAH when reared under conditions that promote rapid growth. Incidences of IPAH exceeding 20% have been induced in broiler chickens by subjecting them to conditions that further increase the cardiac output or pulmonary vascular resistance, including exposure to sub-thermoneutral temperatures, hypobaric hypoxia, poor air quality, respiratory disease, or partial occlusion of the pulmonary vasculature (Wideman, Citation2000, Citation2001; Wideman et al., Citation2007). Broilers with IPAH consistently exhibit medial hypertrophy within the 25 to 100 µm diameter inter-parabronchial pulmonary arteries that are homologous to the pre-acinar arteries of mammals (Cueva et al., Citation1974; Hernandez, Citation1987; Pan et al., Citation2005; Tan et al., Citation2005a, Citation2005b). Intimal proliferation has been observed within the muscularized pulmonary arteries of broilers developing IPAH (Xiang et al., Citation2002; Tan et al., Citation2005a, Citation2005b). Pulmonary arterial pressure (PAP) is elevated beyond the normal level in broiler chickens with IPAH. PAP ≥25 mmHg coupled with wedge pressures ≤15 mmHg are specifically diagnostic for IPAH attributable to elevated arteriole (precapillary) resistance (Wideman et al., Citation2007). In our previous study, PAP and wedge pressures were recorded from 8-week-old, 12-week-old, 16-week-old, 20-week-old and 24-week-old male and female broilers from a IPAH-susceptible line. The PAP values in these broilers ranged from 12 to 62 mmHg and the wedge pressures averaged 12 mmHg. Broilers with elevated PAP consistently exhibited the haemodynamic characteristics of IPAH, which reaffirms that increased PAP is a reliable diagnostic indicator for IPAH (Wideman et al., Citation2010).
A recent study in our laboratory demonstrated for the first time the presence of the plexiform-like CVL in the lungs of broiler chickens that are susceptible to IPAH. It must be noted that these broilers were reared in normoxic conditions and the plexiform-like CVL developed spontaneously. These CVL in broiler lungs resembled histologically the plexiform lesions in humans suffering from IPAH (Wideman et al., Citation2011). The histology and maturation of plexiform-like CVL in broiler lungs has been discussed in detail previously (Wideman & Hamal, Citation2011; Wideman et al., Citation2011). These studies also showed that plexiform lesions developed in both females and males without gender difference, and lesion incidences (lung sections with lesions/lung sections examined) averaged approximately 40% in 8-week-old to 52-week-old broilers. A typical mature plexiform-like CVL in the lung of a broiler is shown in . Macrophages having a foam-type appearance (arrow) are arrayed adjacent to the remnants of the dilated arterial wall, surrounding a compact, fairly homogeneous core of proliferating intimal cells (arrowhead). Perivascular mononuclear and inflammatory cell infiltrates were also observed in the CVL of the lungs of the broiler chickens (Wideman & Hamal, Citation2011; Wideman et al., Citation2011).
Figure 1. 1A: Mature plexiform-like CVL in the lung of a 24-week-old-broiler from a line selected for susceptibility to IPAH. The CVL occupies the position of an inter-parabronchial arteriole supplying two parabronchi on the ventral surface of the lung (100×). 1B: Foam-type macrophages (arrow) are present around the periphery of a matrix of intimal proliferating cells (arrowhead) at the centre of the CVL (400×).
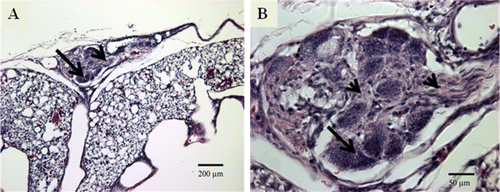
Having demonstrated that the plexiform-like CVL in the lungs of IPAH-susceptible broilers closely mirrors the histological appearance of plexiform lesions in humans, we wanted to examine the expression of key angioproliferative molecules and immune/inflammatory cells that were previously demonstrated to be expressed in human plexiform lesions using cell-specific immunohistochemical markers. The objective of this study was to examine immunohistochemically the expression of various angioproliferative molecules and immune/inflammatory cells in the plexiform-like CVL in the lungs of the broiler chickens from an IPAH-susceptible line.
Materials and Methods
Bird source and management
All procedures involving broiler chickens were approved by the University of Arkansas Institutional Animal Care and Use Committee. Male and female chicks from the IPAH-susceptible broiler line were reared in environmental chambers within the Poultry Environmental Research Laboratory under normoxic and thermoneutral conditions to emphasize the spontaneous onset of PAH and the plexiform-like CVL in the lungs of broiler chickens. The IPAH-susceptible broiler lines were developed through divergent selection by rearing them in a hypobaric chamber. At the ninth generation of selection, when reared under conditions of hypobaric hypoxia, broilers from the IPAH-susceptible line exhibited IPAH-related mortality of 75% compared with the 7.5% for broilers from the IPAH-resistant line. The photoperiod was set for 23 h light:1 h dark for the first 4 days, and 16 h light:8 h dark thereafter. Thermoneutral temperatures were maintained throughout: 32°C for days 1 to 3, 31°C for days 4 to 6, 29°C for days 5 to 10, 26°C for days 11 to 14, and 24°C thereafter. Chicks initially were placed on the floor on wood-shavings litter at a density of 930 cm2/bird, and by 6 weeks of age the bird density had been reduced to >2000 cm2/bird. A corn and soybean meal-based feed formulated to meet or exceed the minimum National Research Council (Citation1994) standards for all ingredients (22.7% crude protein, 3059 kcal metabolizable energy (ME)/kg, 1.5% arginine, and 1.43% lysine) was provided ad libitum. Water was also available ad libitum via nipple watering systems.
Lung tissue collection and fixation
At 8, 12, 16, 20 and 24 weeks of age, at least 12 randomly selected broilers of each gender were anesthetized to a surgical plane with intra-muscular injections of allobarbitol (5,5-diallylbarbituric acid, 3.0 ml, 25 mg/ml) and ketamine HCl (1.0 to 2.5 ml of 100 mg/ml). Heparinized saline (1 ml per bird of 200 units/ml ammonium heparin in 0.9% NaCl) was injected intravenously, after which the broiler chickens were euthanized by exsanguination. Lungs were fixed as described previously (Wideman et al., Citation2011). In brief, the heart was exposed by retracting the sternum, the right atrium was clamped with a haemostat, the left atrium was opened for drainage, and polyethylene tubing (2.5 mm I.D.) inserted via a slit in the right ventricle was used to flush the pulmonary vasculature with 200 ml of 0.9% NaCl. The lungs then were fixed in situ by transcardiac perfusion with 200 to 400 ml freshly prepared 4% phosphate-buffered paraformaldehyde. Carboys containing the 0.9% NaCl and the 4% paraformaldehyde were positioned at an elevation sufficient to obtain a gravimetric perfusion pressure of 46 cmH2O (34 mmHg) at the level of the heart. The thoracic cavity was flooded with 4% paraformaldehyde and the lungs remained fixed in situ for a minimum of 3 h, after which the lungs were removed and cut in the transverse plane at the major rib indentations (costal sulci). These lungs were immersed overnight in 4% paraformaldehyde, after which they were rinsed briefly in tap water, dehydrated in 25% and 50% ethanol for 30 min each, and stored in 70% ethanol. After fixation, the lung tissues were dehydrated in graded series of alcohol, cleared, and then embedded in paraffin blocks. The paraffin blocks were serial sectioned at 5 µm thickness (40 to 50 sections/lung samples). Every third section was stained with haematoxylin and eosin for histological evaluation and the remaining sections were used for immunohistochemistry.
Immunohistochemistry
The haematoxylin-and-eosin-stained lung sections were first examined to ascertain the presence of the CVL, then the unstained sections adjacent to the haematoxylin and eosin sections having CVL were used for immunohistochemistry. A minimum of 12 sections with CVL were used for immunohistochemical staining to identify and localize each angioproliferative molecule and immune/inflammatory cells.
Paraffin-embedded sections were deparaffinized by placing them in two xylene baths for 5 min each and then rehydrating the tissues in a graded series of alcohol (100%, 100%, 95%, 70%, and 50%) for 3 min each. Antigens (targets) were retrieved/exposed by heating these sections in Coplin jars filled with target retrieval solution (DAKO, Carpinteria, California, USA) in a water bath at 95 to 99°C for 30 min. The sections were allowed to cool for 20 min at room temperature and were washed with Tris buffer (washing was done after each incubation hereafter). Endogenous peroxidase activity in the lung sections was blocked by incubating with 150 µl DAKO dual endogenous block enzyme for 10 min. The lung sections were then incubated for 30 min with 150 µl diluted primary antibodies (primary antibodies with their specificity are listed in ).
Table 1. Primary antibodies used for immunohistochemistry.
Following primary antibody incubation, for αSMA and vWF the lung sections were incubated with 150 µl DAKO Envision+ dual-link (anti-mouse/anti-rabbit) horseradish peroxidase (1:50 dilution) for 30 min followed by incubation with 150 µl DAB+ substrate chromogen solution (DAKO) for 10 min. For the rest of the molecules/cells, following primary antibody incubation, the lung sections were incubated with 150 µl diluted biotinylated horse anti-mouse IgG (1:50 dilution; Vector Laboratories Inc., Burlingame, California, USA)—except for chicken Ia (anti-mouse IgM), where biotinylated goat anti-mouse IgM was used as the detection antibody for 30 min. The lung sections were then incubated with 150 µl ABC reagent (Vector Laboratories Inc.) for 30 min followed by incubation with 150 µl DAB substrate (Sigma-Aldrich, St Louis, Missouri, USA) for 10 min. After 10 min, the tissue sections were washed with deionized water, counterstained with haematoxylin (DAKO) for 20 sec, dehydrated in a graded series of alcohol (50%, 70%, 95%, 100%, 100%) for 3 min each, cleared in xylene, and mounted.
Results
By immunohistochemical staining, we were able to demonstrate the expression of various angioproliferative molecules and immune/inflammatory cells in the CVL in the lungs of 8-week-old, 12-week-old, 16-week-old, 20-week-old, and 24-week-old broilers from an IPAH-susceptible line. Brown colour development indicated positive staining. The positive staining was classified qualitatively as strong, moderate or weak depending on the intensity of staining (brown colour).
Expression of angioproliferative molecules
vWF was expressed strongly in both intimal proliferating cells and the foam-type macrophages (A), whereas αSMA was expressed moderately in the intimal proliferating cells and strongly in the foam-type macrophages (B). VEGF (C), VEGFR-2 (D), HIF-1α (E), and survivin (F) showed strong expression in the foam-type macrophages and moderate expression in the intimal proliferating cells. Tenascin (G) had strong expression in the intimal proliferating cells but weak expression in the foam-type macrophages. Fibronectin (H) was expressed strongly in the foam-type macrophages but moderately in the intimal proliferating cells. Collagen type III (I) had strong expression in the intimal proliferating cells but was not expressed in the foam-type macrophages, whereas collagen type IV (J) had strong expression in the foam-type macrophages and moderate expression in the intimal proliferating cells.
Figure 2. Photomicrographs of immunostained lung sections from IPAH-susceptible broilers showing positive staining for angioproliferative molecules that include (2A) vWF, (2B) αSMA, (2C) VEGF, (2D) VEGFR-2, (2E) HIF-1α, (2F) survivin, (2G) tenascin, (2H) fibronectin, (2I) collagen type III, and (2J) collagen type IV. It must be noted that we examined the lung sections from 8-week-old to 24-week-old broilers. The lung sections presented here are from 12-week-old broilers except for VEGFR-2 (20 weeks old). The brown-coloured reaction product indicates positive staining. For each molecule, the foam-type macrophages are indicated by an arrow and the matrix of intimal proliferating cells by an arrowhead. Original magnification ×400, scale bar =50 µm.
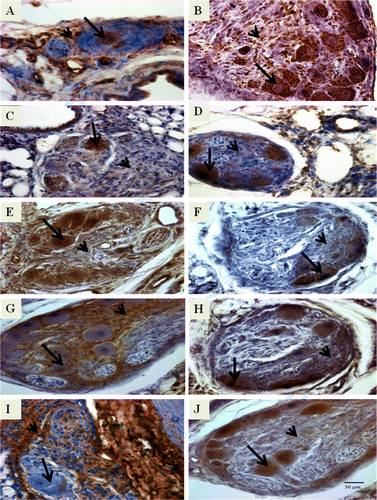
Expression of immune/inflammatory cell markers
Immune/inflammatory cells were detected using mouse monoclonal primary antibodies that were specific for cell surface markers such as chicken KUL01, chicken CD8α, chicken Bu-1, and chicken Ia. Expression of these cell surface markers was used to determine the presence of monocytes/macrophages, cytotoxic lymphocytes, B cells, and MHC class II cells, respectively. Monocytes/macrophages (KUL01) (A), cytotoxic lymphocytes (CD8α) (B), and B cells (Bu-1) (C) were expressed strongly by the foam-type macrophages and moderately by the intimal proliferating cells. MHC class II cells (Ia) (D) were expressed strongly by the intimal proliferating cells but weakly by the foam-type macrophages.
Figure 3. Photomicrographs of immunostained lung sections from IPAH-susceptible broiler chickens showing positive staining for immune/inflammatory cell markers that include (3A) monocytes/macrophages (KUL01), (3B) cytotoxic lymphocytes (CD8), (3C) B cells (Bu-1), and (3D) MHC class II cells (Ia). It must be noted that we examined the lung sections from 8-week-old to 24-week-old broilers. The lung sections presented here are from 12-week-old broilers. The brown-coloured reaction product indicates positive staining. For each immune/inflammatory cell, the foam-type macrophages are indicated by an arrow and matrix of intimal proliferating cells by an arrowhead. Original magnification ×400, scale bar = 50 µm.
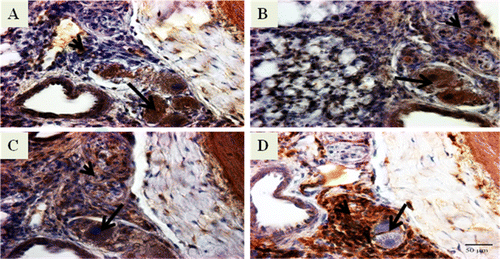
Discussion
The objective of this study was to examine the expression of angioproliferative cell markers and immune/inflammatory cell markers in plexiform-like CVL in the lungs of IPAH-susceptible broilers. Our results demonstrated positive staining (i.e. strong, moderate, weak expression) of these molecules and the presence of marker-defined cells in the plexiform-like CVL in the lungs of 8-week-old to 24-week-old broilers. The histology of the plexiform-like CVL in lungs of IPAH-susceptible broilers has been described in detail in our previous study (Wideman et al., Citation2011), which demonstrated that the broilers had plexiform lesion incidence (calculated as number lung sections with at least one plexiform lesion divided by the number of lung sections examined) of 52%, 50%, 51%, 40%, and 36% at 8, 12, 16, 20, and 24 weeks of age, respectively.
In this study, there was strong expression of vWF and αSMA in the CVL, indicating active proliferation of endothelial and smooth muscle cells, a hallmark of plexiform lesion formation (Hirose et al., Citation2000). As vWF is synthesized mainly in the endothelial cells, higher expression of vWF acts as marker for endothelial cell proliferation (Collados et al., Citation1999), whereas expression of αSMA acts as marker for smooth muscle cell proliferation (Owens, Citation1995). Both vWF and αSMA were expressed abundantly in the plexiform lesions of IPAH patients (Tuder et al., Citation2007). Expression of these two key markers for endothelial cell and smooth muscle in the CVL is therefore a strong indication that the key event in development of CVL in broiler lungs may also involve the proliferation of endothelial cells and smooth muscle cells.
VEGF and VEGFR-2 were expressed at high levels in the CVL in broiler lungs. Both VEGF and VEGFR-2 are very critical in the survival of the newly-forming endothelial cells (Gerber et al., Citation1998; Hirose et al., Citation2000) and VEGF also has mitogenic effects on PASMC (Tuder et al., Citation2001). Strong expression of VEGF and VEGFR-2 therefore suggests that these molecules may contribute to the survival and proliferation of the endothelial cells and PASMC in the CVL of broiler lungs. Elevated expression of HIF-1α in CVL suggests that the increased expression VEGF is due to increased transcription of the VEGF gene because HIF-1α is a main activator of the VEGF gene in response to hypoxia (Tuder et al., Citation2001). Additionally HIF-1α also up-regulates many other genes such as endothelin-1 that are associated with pathogenesis of PAH (Yamashita et al., Citation2001). Higher expression of survivin, an anti-apoptotic molecule, suggests that it may induce proliferation of endothelial cells and smooth muscle cells and also prevent the apoptosis of the vascular cells. Previous in vivo and in vitro studies have shown the ability of endogenous survivin to inhibit PASMC apoptosis. Additionally, gene transfer of wild-type survivin into PASMC resulted in proliferation of PASMC (Hassoun et al., Citation2009). Survivin also induces receptors for platelet-derived growth factor in PASMC (Wang et al., Citation2005). Platelet-derived growth factor has been considered a key mediator in the progression of several fibroproliferative disorders including IPAH because the binding of platelet-derived growth factor to its receptor leads to the proliferation and migration of the PASMC and fibroblasts (Humbert et al., Citation1998).
We also observed strong expression of extracellular matrix components such as collagen (type III and type IV), tenascin, and fibronectin in broiler CVL. The accumulation of the extracellular matrix is increased in the pulmonary vessels of PAH patients (Haworth, Citation1988) and active synthesis and deposition of collagen is believed to contribute to the thickening of the pulmonary arterioles and also to the fibrotic nature of the plexiform lesions (Meyrick & Reid, Citation1980; Botney et al., Citation1993). Increased synthesis and accumulation of tenascin and fibronectin may also contribute to the proliferation of smooth muscle cells (Clausell & Rabinovitch, Citation1993; Jones et al., Citation1997). Increased expression of extracellular matrix components in CVL therefore points to the similarity in the pathophysiological events involved in the formation of CVL in broiler lungs and the formation of plexiform lesions in the lungs of human IPAH patients.
Overall the angioproliferative molecules contribute to the proliferation of either endothelial cells and/or smooth muscle cells (VEGF, VEGFR-2, fibronectin, tenascin), prevent apoptosis of vascular smooth muscle cells (survivin), and contribute to the thickening of the pulmonary arterioles (collagen). The net effect of increased expression of these molecules and the multiple interactions among them may lead to the formation of the plexiform-like CVL in broiler lungs.
In addition to angioproliferative molecules, the immune/inflammatory cell markers, including markers for CD8+ cells (cytotoxic lymphocytes), B cells, MHC class II cells (macrophages, dendritic cells, activated T cells, and B cells), and monocytes/macrophages were expressed strongly in the broiler CVL. The presence of the perivascular cell infiltrates composed of T lymphocytes and B lymphocytes and macrophages suggests that immune cells may play a key role in the development of CVL similar to the development of plexiform lesions in human PAH patients (Tuder et al., Citation1994; Cool et al., Citation1997; Dorfmüller et al., Citation2003). In the lungs of PAH patients, expression of inflammatory cytokines and chemokines such as IL-1β, IL-6, monocyte chemoattractant protein-1, and RANTES was elevated (Humbert et al., Citation1995; Dorfmüller et al., Citation2002; Itoh et al., Citation2006). These chemokines and cytokines play a key role in migration as well as activation of the macrophages, resulting in their increased inflammatory activities. It has been postulated that the release of cytokines, chemokines, and growth factors by inflammatory cells may contribute to the proliferation and migration of PASMC, a key event in the development of plexiform lesion (Tajsic & Morrell, Citation2011). Increased expression of immune/inflammatory cells in CVL therefore hints that these cells may contribute to plexiform lesion development by interacting with angioproliferative molecules and growth factors in broiler lungs. For both angioproliferative molecules and immune/inflammatory cell markers, the foam-type macrophages exhibited strong (more intense) expression of many markers that were not expressed or only weakly expressed by the intimal proliferating cells. It is likely that during the inflammatory process the foam-type macrophages ingest many types of cells and cellular debris, which may contribute to the enhanced expression of various markers by the foam-type macrophages. There were some exceptions to this trend, as some molecules were either expressed weakly (tenascin, MHC class II cells) or not expressed at all (collagen type II) by foam-type macrophages.
In summary, CVL in the lungs of the broiler chickens from an IPAH-susceptible broiler line showed high expression of the angioproliferative molecules (vWF, αSMA, VEFG, VEGFR-2, HIF-1α, survivin, tenascin, fibronectin, collagen type III and collagen type IV) and immune/inflammatory cells (cytotoxic lymphocytes, B cells, MHC class II cells, and monocytes/macrophages), which is very similar to the expression of these cells/molecules in the plexiform lesions in lungs of human IPAH patients. These similarities suggest that the pathophysiological events or pathways involved in the formation of CVL in the lungs of broiler chickens may be similar to the pathophysiological events or pathways involved in the formation of plexiform lesions in the lungs of human patients. One can therefore infer that, in addition to the histological resemblance of avian and human plexiform lesions, the same types of mediators appear to be involved in formation of CVL in both species, further validating the IPAH-susceptible broiler chicken as an excellent animal model of spontaneous plexogenic arteriopathy.
Acknowledgements
Supported by NIH/National Heart Lung Blood Institute Grant 1R15HL092517 01. The authors thank David Cross of the Department of Poultry Science, University of Arkansas for sectioning formalin-fixed, paraffin-embedded lung tissues for immunohistochemistry. They would also like to thank Dr Edward M. Dzialowski, Department of Biological Science, University of North Texas for providing the immunohistochemistry protocol. The monoclonal antibodies—namely, mouse anti-chicken collagen type III developed by Dr Richard Mayne, mouse anti-human collagen type IV developed by Dr Heinz Furthmayr, mouse anti-chicken tenascin developed by Dr Douglas M. Famborough and mouse anti-chicken fibronectin developed by Dr Michael Solursh—were obtained from the Developmental Studies Hybridoma Bank developed under the auspices of the NICHD and maintained by the Department of Biology, The University of Iowa.
References
- Bonnet , S. , Michelakis , E.D. , Porter , C.J. , Andrade-Navarro , M.A. , Thebaud , B. , Bonnet , S. , Haromy , A. , Harry , G. , Moudgil , R. , McMurtry , M.S. , Weir , E.K. and Archer , S. L. 2006 . An abnormal mitochondrial-hypoxia inducible factor-1alpha-Kv channel pathway disrupts oxygen sensing and triggers pulmonary arterial hypertension in fawn hooded rats: similarities to human pulmonary arterial hypertension . Circulation , 113 : 2630 – 2641 .
- Botney , M.D. , Liptay , M.J. , Kaiser , L.R. , Cooper , J.D. , Parks , W.C. and Mecham , R.P. 1993 . Active collagen synthesis by pulmonary arteries in human primary pulmonary hypertension . American Journal of Pathology , 143 : 121 – 129 .
- Clausell , N. and Rabinovitch , M. 1993 . Upregulation of fibronectin synthesis by interleukin-1β in coronary artery smooth muscle cells is associated with the development of the postcardiac transplant arteriopathy in piglets . The Journal of Clinical Investigation , 92 : 1850 – 1858 .
- Collados , M.T. , Sandoval , J. , Lopez , S. , Masso , F.A. , Paez , A. , Borbolla , J.R. and Montano , L. F. 1999 . Characterization of von Willebrand factor in primary pulmonary hypertension . Heart and Vessels , 14 : 246 – 252 .
- Cool , C.D. , Kennedy , D. , Voelkel , N.F. and Tuder , R.M. 1997 . Pathogenesis and evolution of plexiform lesions in pulmonary hypertension associated with scleroderma and human immunodeficiency virus infection . Human Pathology , 28 : 434 – 442 .
- Cool , C.D. , Stewart , J.S. , Gary , P.W. , Miller , J. , Williams , R.L. , Voelkel , N. F. and Tuder , R. M. 1999 . Three-dimensional reconstruction of pulmonary arteries in plexiform pulmonary hypertension using cell-specific markers. Evidence for a dynamic and heterogeneous process of pulmonary endothelial cell growth . The American Journal of Pathoogy , 155 : 411 – 419 .
- Cool , C.D. , Groshong , S.D. , Oakey , J. and Voelkel , N.F. 2005 . Pulmonary hypertension: cellular and molecular mechanisms . Chest , 128 : 565S – 571S .
- Cueva , S. , Sillau , H. , Valenzuela , A. and Ploog , H. 1974 . High altitude induced pulmonary hypertension and right ventricular failure in broiler chickens . Research in Veterinary Science , 16 : 370 – 374 .
- Dorfmüller , P. , Perros , F. , Balabanian , K. and Humbert , M. 2003 . Inflammation in pulmonary arterial hypertension . European Respiratory Journal , 22 : 358 – 363 .
- Dorfmüller , P. , Zarka , V. , Durand-Gasselin , I. , Monti , G. , Balabanian , K. , Garcia , G. , Capron , F. , Coulomb-Lhermine , A. , Marfaing-Koka , A. , Simonneau , G. , Emilie , D. and Humbert , M. 2002 . Chemokine RANTES in severe pulmonary arterial hypertension . American Journal of Respiratory and Critical Care Medicine , 165 : 534 – 539 .
- Gerber , H.P. , McMurtrey , A. , Kowalski , J. , Yan , M. , Keyt , B.A. , Dixit , V. and Ferrara , N. 1998 . Vascular endothelial growth factor regulates endothelial cell survival through the phosphatidylinositol 3′-kinase/Akt signal transduction pathway: requirement for Flr/KDR activation . The Journal of Biological Chemistry , 273 : 30336 – 30343 .
- Hassoun , P.M. , Mouthon , L. , Barbera , J.A. , Eddahibi , S. , Flores , S.C. , Grimminger , F. , Jones , P.L. , Maitland , M.L. , Michelakis , E.D. , Morrell , N.W. , Newman , J.H. , Rabinovitch , M. , Schermuly , R. , Stenmark , K.R. , Voelkel , N.F. , Yuan , J.X. and Humbert , M. 2009 . Inflammation, growth factors, and pulmonary vascular remodeling . Journal of the American College of Cardiology , 54 : S10 – S19 .
- Haworth , S.G. 1988 . Pulmonary vascular remodeling in neonatal pulmonary hypertension . Chest , 93 : 133S – 138S .
- Heath , D. , Smith , P. and Gosney , J. 1988 . Ultrastructure of early plexogenic pulmonary arteriopathy . Histopathology , 12 : 41 – 52 .
- Hernandez , A. 1987 . Hypoxic ascites in broilers: a review of several studies done in Colombia . Avian Diseases , 31 : 171 – 183 .
- Hirose , S. , Hosoda , Y. , Furuya , S. , Otsuki , T. and Ikeda , E. 2000 . Expression of vascular endothelial growth factor and its receptors correlates closely with formation of the plexiform lesion of human pulmonary hypertension . Pathology International , 50 : 472 – 479 .
- Holmes , K. , Roberts , O.L. , Thomas , A.M. and Cross , M.J. 2007 . Vascular endothelial growth factor receptor-2: structure, function, intracellular signalling and therapeutic inhibition . Cellular Signalling , 19 : 2003 – 2012 .
- Humbert , M. , Monti , G. , Brenot , F. , Sitbon , O. , Portier , A. , Grangeot-Keros , L. , Duroux , P. , Galanaud , P. , Simonneau , G. and Emilie , D. 1995 . Increased interleukin-1 and interleukin-6 serum concentrations in severe primary pulmonary hypertension . American Journal of Respiratory and Critical Care Medicine , 151 : 1628 – 1631 .
- Humbert , M. , Monti , G. , Fartoukh , M. , Magnan , A. , Brenot , F. , Rain , B. , Capron , F. , Galanaud , P. , Duroux , P. , Simonneau , G. and Emilie , D. 1998 . Platelet-derived growth factor expression in primary pulmonary hypertension: comparison of HIV seropositive and HIV seronegative patients . European Respiratory Journal , 11 : 554 – 559 .
- Itoh , T. , Nagaya , N. , Ishibashi-Ueda , H. , Kyotani , S. , Oya , H. , Sakamaki , F. , Kimura , H. and Nakanishi , N. 2006 . Increased plasma monocyte chemoattractant protein-1 level in idiopathic pulmonary arterial hypertension . Respirology , 11 : 158 – 163 .
- Jones , P.L. and Rabinovitch , M. 1996 . Tenascin-C is induced with progressive pulmonary vascular disease in rats and is functionally related to increased smooth muscle cell proliferation . Circulation Research , 79 : 1131 – 1141 .
- Jones , P.L. , Cowan , K.N. and Rabinovitch , M. 1997 . Tenascin-C, proliferation and subendothelial fibronectin in progressive pulmonary vascular disease . The American Journal of Pathology , 150 : 1349 – 60 .
- Masri , F.A. , Xu , W. , Comhair , S.A. , Asosingh , K. , Koo , M. , Vasanji , A. , Drazba , J. , Anand-Apte , B. and Erzurum , S.C. 2007 . Hyperproliferative apoptosis-resistant endothelial cells in idiopathic pulmonary arterial hypertension . American Journal of Physiology Lung Cellular Molecular Physiology , 293 : L548 – L554 .
- McMurtry , M.S. , Archer , S.L. , Altieri , D.C. , Bonnet , S. , Haromy , A. , Harry , G. , Bonnet , S. , Puttagunta , L. and Michelakis , E.D. 2005 . Gene therapy targeting survivin selectively induces pulmonary vascular apoptosis and reverses pulmonary arterial hypertension . The Journal of Clinical Investigation , 115 : 1479 – 1491 .
- Meyrick , B. and Reid , L. 1980 . Hypoxia-induced structural changes in the media and adventitia of rat hilar pulmonary artery, and their regression . The American Journal of Pathology , 101 : 151 – 178 .
- Mitani , Y. , Ueda , M. , Komatsu , R. , Maruyama , K. , Nagai , R. , Matsumura , M. and Sakurai , M. 2001 . Vascular smooth muscle cell phenotypes in primary pulmonary hypertension . European Respiratory Journal , 17 : 316 – 320 .
- Morrell , N. , Adnot , S. , Archer , S. , Dupuis , J. , Jones , P. , MacLean , M.R. , McMurtry , I.F. , Stenmark , K.R. , Thistlethwaite , P.A. , Weissmann , N. , Yuan , J.X. and Weir , E.K. 2009 . Cellular and molecular basis of pulmonary arterial hypertension . Journal of the American College of Cardiology , 54 : S20 – S31 .
- National Research Council, (NRC) ., 1994 , Nutrient Requirements of Poultry . 9th rev. ed . The National Academies Press , Washington , DC .
- Owens , G.K. 1995 . Regulation of differentiation of vascular smooth muscle cells . Physiological Reviews , 75 : 487 – 517 .
- Pan , J.Q. , Tan , X. , Li , J.C. , Sun , W.D. and Wang , X.L. 2005 . Effects of early feed restriction and cold temperature on lipid peroxidation, pulmonary vascular remodeling and ascites morbidity in broilers under normal and cold temperatures . British Poultry Science , 46 : 374 – 381 .
- Tajsic , T. and Morrell , N.W. 2011 . Smooth muscle cell hypertrophy, proliferation, migration and apoptosis in pulmonary hypertension . Comprehensive Physiology , 1 : 295 – 317 .
- Tan , X. , Liu , Y.J. , Li , J.C. , Pan , J.Q. , Sun , W.D. and Wang , X.L. 2005a . Activation of PCKα and pulmonary vascular remodeling in broilers . Research in Veterinary Science , 79 : 131 – 137 .
- Tan , X. , Pan , J.Q. , Li , J.C. , Liu , Y.J. , Sun , W.D. and Wang , X.L. 2005b . L-Arginine inhibiting pulmonary vascular remodelling is associated with promotion of apoptosis in pulmonary arterioles smooth muscle cells in broilers . Research in Veterinary Science , 79 : 203 – 209 .
- Taraseviciene-Stewart , L. , Kasahara , Y. , Alger , L. , Hirth , P. , McMahon , G. , Waltenberger , J. , Voelkel , N.F. and Tuder , R.M. 2001 . Inhibition of the VEGF receptor 2 combined with chronic hypoxia causes cell death dependent pulmonary endothelial cell proliferation and severe pulmonary hypertension . Journal of the Federation of American Societies for Experimental Biology , 15 : 427 – 438 .
- Tuder , R.M. , Groves , B. , Badesch , D.B. and Voelkel , N.F. 1994 . Exuberant endothelial cell growth and elements of inflammation are present in plexiform lesions of pulmonary hypertension . The American Journal of Pathology , 144 : 275 – 285 .
- Tuder , R.M. , Chacon , M. , Alger , L. , Wang , J. , Taraseviciene-Stewart , L. , Kasahara , Y. , Cool , C.D. , Bishop , A.E. , Geraci , M. , Semenza , G.L. , Yacoub , M. , Polak , J.M. and Voelkel , N.F. 2001 . Expression of angiogenesis-related molecules in plexiform lesions in severe pulmonary hypertension: Evidence for a process of disordered angiogenesis . The Journal of Pathology , 195 : 367 – 374 .
- Tuder , R.M. , Marecki , J.C. , Richter , A. , Fijalkowska , I. and Flores , S. 2007 . Pathology of pulmonary hypertension . Clinics in Chest Medicine , 28 : 23 – 42 .
- Tuder , R.M. , Abman , S.H. , Braun , T. , Capron , F. , Stevens , T. , Thistlethwaite , P.A. and Haworth , S.G. 2009 . Development and pathology of pulmonary hypertension . Journal of the American College of Cardiology , 54 : S3 – S9 .
- Veyradier , A. , Nishikubo , T. , Humbert , M. , Wolf , M. , Sitbon , O. , Simonneau , G. , Girma , J.P. and Meyer , D. 2000 . Improvement of von Willebrand factor proteolysis after prostacyclin infusion in severe pulmonary arterial hypertension . Circulation , 102 : 2460 – 2462 .
- Wang , G.L. , Jiang , B.H. , Ru , E.A. and Semenza , G.L. 1995 . Hypoxia-inducible factor 1 is a basic helix-loop-helix-PAS heterodimer regulated by cellular O2 tension . Proceedings of the National Academy of Sciences of the United States of America , 92 : 5510 – 5514 .
- Wang , G.J. , Sui , X.X. , Simosa , H.F. , Jain , M.K. , Altieri , D.C. and Conte , M. S. 2005 . Regulation of vein graft hyperplasia by survivin, an inhibitor of apoptosis protein . Arteriosclerosis Thrombosis and Vascular Biology , 25 : 2081 – 2087 .
- Wideman , R.F. 2000 . Cardio-pulmonary hemodynamics and ascites in broiler chickens . Avian and Poultry Biology Reviews , 11 : 21 – 43 .
- Wideman , R.F. 2001 . Pathophysiology of heart/lung disorders: pulmonary hypertension syndrome in broiler chickens . World's Poultry Science Journal , 57 : 289 – 307 .
- Wideman , R.F. , Chapman , M.E. , Hamal , K.R. , Bowen , O.T. , Lorenzoni , A.G. , Erf , G.F. and Anthony , N.B. 2007 . An inadequate pulmonary vascular capacity and susceptibility to pulmonary arterial hypertension in broilers . Poultry Science , 86 : 984 – 998 .
- Wideman , R.F. , Eanes , M.L. , Hamal , K.R. and Anthony , N.B. 2010 . Pulmonary vascular pressure profiles in broilers selected for susceptibility to pulmonary hypertension syndrome: age and gender comparisons . Poultry Science , 89 : 1815 – 1824 .
- Wideman , R.F. and Hamal , K.R. 2011 . Idiopathic pulmonary arterial hypertension: an avian model for plexogenic arteriopathy and serotonergic vasoconstriction . Journal of Pharmacological and Toxicological Methods , 63 : 283 – 295 .
- Wideman , R.F. , Hamal , K.R. , Bayona , M.T. , Lorenzoni , A.G. , Cross , D. , Khajali , F. , Rhoads , D.D. , Erf , G.F. and Anthony , N.B. 2011 . Plexiform lesions in the lungs of domestic fowl selected for susceptibility to pulmonary arterial hypertension: incidence and histology . The Anatomical Record , 294 : 739 – 755 .
- Xiang , R.P. , Sun , W.D. , Wang , J.Y. and Wang , X.L. 2002 . Effect of vitamin C on pulmonary hypertension and muscularization of pulmonary arterioles in broilers . British Poultry Science , 43 : 705 – 712 .
- Xu , W. , Koeck , T. , Lara , A.R. , Neumann , D. , DiFilippo , F.P. , Koo , M. , Janocha , A.J. , Masri , F.A. , Arroliga , A.C. , Jennings , C. , Dweik , R.A. , Tuder , R.M. , Stuehr , D.J. and Erzurum , S. C. 2007 . Alterations of cellular bioenergetics in pulmonary artery endothelial cells . Proceedings of the National Academy of Sciences of the United States of America , 104 : 1342 – 1347 .
- Yamashita , K. , Discher , D.J. , Hu , J. , Bishopric , N.H. and Webster , K.A. 2001 . Molecular regulation of the endothelin-1 gene by hypoxia. Contributions of hypoxia-inducible factor-1, activator protein-1, GATA-2, and p300/CBP . Journal of Biological Chemistry , 276 : 12645 – 12653 .