Abstract
The purpose of this study was to investigate the prevalence and characteristics of plasmid-mediated quinolone resistance (PMQR) genes qnr, aac(6′)-Ib-cr, and qepA in a total of 185 non-duplicate Salmonella spp. isolated from hatcheries, poultry farms, and poultry slaughterhouses during the period 2001 to 2010 in Korea. Additionally, mutation analysis of quinolone resistance determining regions (QRDRs), conjugation experiments, and plasmid analysis were performed in the PMQR-positive isolates. Among the 185 isolates, six (3.2%) contained qnr genes (two qnrB4 and four qnrS1) but none carried the aac(6′)-Ib-cr or qepA genes. Among the six PMQR-positive isolates, one showed a single mutation (Ser83-Phe substitution) in the QRDRs of gyrA. Among them, three were non-susceptible (intermediate or resistant) to nalidixic acid (minimum inhibitory concentration [MIC] ≥256 µg/ml), ciprofloxacin (MIC 2 µg/ml), and levofloxacin (MIC 4 µg/ml), but others were susceptible to all of the three fluoroquinolones. They were resistant to six or more antimicrobial agents tested and were able to transfer quinolone resistance to recipient Escherichia coli J53 by conjugation. By performing a hybridization test, plasmids harbouring qnrB4 and qnrS1 genes were less than 8 kb and about 70 kb in size, respectively. The horizontal dissemination of qnrS1 gene was mediated by IncN plasmid. Compared with the recipient strain, MICs of the transconjugants increased two-fold to four-fold for nalidixic acid, and eight-fold to 16-fold for ciprofloxacin and levofloxacin. This report is the first to describe the detection of qnr genes in Salmonella spp. isolated from poultry in Korea. Widespread horizontal transfer of these genes among bacteria may be a serious public health concern because these can rapidly increase fluoroquinolone resistance. To ensure the public health, it is essential to continuously survey and carefully monitor the spread of PMQR genes in Salmonella from poultry.
Introduction
Salmonellosis is an important food-borne infection in both humans and animals. Salmonella species are the leading causes of gastrointestinal infections, and salmonellosis represents a major public health problem worldwide (Marcus, Citation2008). Infections via poultry products, especially meat and eggs, are the primary cause of Salmonella-associated illness, and increased consumption of poultry products elevates the risk of Salmonella infection (CDC, Citation2011).
Fluoroquinolones are synthetic and important antimicrobial agents for treating bacterial infections in both humans and animals (Hopkins et al., Citation2005). Widespread use of these compounds has triggered an increase in bacterial resistance. Resistance to fluoroquinolones can lead to treatment failure and is therefore considered a significant public health concern (Gagliotti et al., Citation2008).
Quinolone resistance is mainly due to chromosomal mutations that alter the drug target enzymes DNA gyrase (gyrA and gyrB) and DNA topoisomerase IV (parC and parE), or activate the efflux systems (Hopkins et al., Citation2005; Jacoby, Citation2005). However, plasmid-mediated quinolone resistance (PMQR) genes have been recently identified in various Enterobacteriaceae, and their prevalence is increasing worldwide (Poirel et al., Citation2012). Since the first PMQR gene, qnrA, was reported in 1998 from a clinical isolate of Klebsiella pneumoniae in the USA (Martínez-Martínez et al., Citation1998), three different PMQR mechanisms have been described. These include: DNA gyrase protection from fluoroquinolone by a pentapeptide repeat family of proteins encoded by qnrA, qnrB, qnrC, qnrD, and qnrS (Rodríguez-Martínez et al., Citation2011); the new variant of aminoglycoside acetyltransferase capable of acetylating a piperazinyl substituent of several fluoroquinolones, aac(6′)-Ib-cr (Robicsek et al., Citation2006a); and the major facilitator superfamily-type quinolone efflux pump decreasing susceptibility to the hydrophilic quinolones, qepA (Yamane et al., Citation2007).
The expression of PMQR genes only confers a low level of quinolone resistance, but it can facilitate selection of additional chromosomal resistance mechanisms, leading to the emergence of high-level quinolone-resistant bacteria (Strahilevitz Citation2009; Tamang et al., Citation2011). Besides, widespread horizontal transfer of these genes among bacteria could contribute to the eventual compromise of the use of fluoroquinolones.
PMQR genes have been detected worldwide in various Enterobacteriaceae (Poirel et al., Citation2012), and have also been found among Salmonella isolated from human and animal sources in many countries (Gay et al., Citation2006; Avsaroglu et al., Citation2007; Cattoir et al., Citation2007; Hopkins et al., Citation2007; Cavaco et al., Citation2009; Cui et al., Citation2009; Asai et al., Citation2010). In Korea, PMQR genes were widely reported in human clinical Enterobacteriaceae isolates (Tamang et al., Citation2008; Kim et al., Citation2010; Jeong et al., Citation2011), but have rarely been identified in Enterobateriaceae isolates from animals (Tamang et al., 2011). Additionally, the presence of PMQR genes in Salmonella spp. isolated from poultry has not yet been reported in Korea. The purpose of this study was to investigate the prevalence of PMQR genes among various serotypes of Salmonella isolated from poultry in Korea and to examine the characteristics of the PMQR-positive isolates.
Materials and Methods
Bacterial isolates
From 2001 to 2010 a total of 185 non-duplicate Salmonella spp. were isolated from poultry in Korea. The bacterial isolates were collected randomly from 22 hatcheries (n = 54), 86 poultry farms (n = 110), and five slaughterhouses (n = 21) located in different regions (). Hatchery samples were obtained from the chick fluff in the hatcher room, chick counting room, paper pads of chick boxes, outdoor ventilating outlets of hatcher rooms, and hatchery waste storehouses. Samples from the poultry farm were obtained from cloacal swabs, caecal droppings, litter, nest boxes, egg-collecting belts, faecal-collecting belts, egg sorting tables, and dust on the wall of sheds. In the poultry slaughterhouse, samples were acquired from the chilling water, faeces from mooring areas (the place where poultry are kept before slaughtering), and carcasses. All isolates were identified using the VITEK system (BioMérieux, Inc., Durham, North Carolina, USA) and serotyping was performed using standard agglutination tests with O and H antisera (Difco Laboratories, Detroit, Michigan, USA) according to a protocol from Difco Laboratories (Citation1977).
Table 1. Distribution of serotypes among the 185 Salmonella isolates from hatcheries, slaughterhouses, and poultry farms in Korea.
Additional strains used were Escherichia coli ATCC 25922 as the quality control strain for antimicrobial susceptibility testing; E. coli J53 (resistant to sodium azide) as a recipient for conjugation; and clinical isolates that had been previously confirmed to carry qnrB and qnrS genes (Tamang et al., Citation2008) as a positive control for detection and hybridization of qnrB and qnrS genes.
Detection of PMQR genes
PMQR genes (qnrA, qnrB, qnrS, qnrC, qnrD, qepA, and aac(6′)-Ib-cr) were detected with a polymerase chain reaction (PCR) assay using previously described primers and protocol (Park et al., Citation2006; Robicsek et al., Citation2006b; Yamane et al., Citation2008; Cavaco et al., Citation2009; Wang et al., Citation2009). To prepare the DNA template, a single colony was suspended in a volume of 100 µl distilled water and boiled followed by a centrifugation step of the cell suspension. PCR was performed in a final volume of 20 µl using AccuPower ® HotStart PCR PreMix (Bioneer Corporation, Daejeon, Korea) according to the manufacturer's protocol in a PCR Thermocycler Dice (Takara, Shiga, Japan). All positive PCR products were purified with a QIAquick gel extraction kit (Qiagen GmbH, Hilden, Germany) and submitted to Bioneer Corporation for sequencing. After both strands were aligned, sequence analysis and comparison with relevant sequences were carried out using the BLAST program (National Center for Biotechnology Information, Bethesda, Maryland, USA).
Detection of mutations in the DNA gyrase and topoisomerase IV genes of the PMQR-positive isolates
DNA was extracted from PMQR-positive isolates by boiling as described above. PCR was carried out to amplify quinolone resistance determining regions (QRDRs) of the target genes (gyrA, gyrB, parC, and parE) to identify mutations in PMQR-positive isolates using primers and protocols described previously (Everett et al., Citation1996). Both strands of the PCR products were sequenced (Bioneer Corporation) and analysed as described above.
Antimicrobial susceptibility test in PMQR-positive isolates
Antimicrobial susceptibility tests for PMQR-positive isolates were performed on Mueller-Hinton agar (Difco Laboratories, Detroit, Michigan, USA) using the agar diffusion method with antimicrobial discs (Becton Dickinson, Franklin Lakes, New Jersey, USA) according to the standards of the Clinical and Laboratory Standards Institutes (CLSI, Citation2007). The 17 antimicrobial agents for this test belonged to the following drug classes: β-lactams (ampicillin [AM, 10 µg], cefotaxime [CTX, 30 µg], ceftazidime [CAZ, 30 µg], aztreonam [ATM, 30 µg], cephalothin [CF, 30 µg], cefazolin [CZ, 30 µg], cefoxitin [FOX, 30 µg], cefepime [FEP, 30 µg] and amoxicillin-clavulanic acid [AmC, 20 and 10 µg, respectively]), aminoglycosides (amikacin [AN, 30 µg], kanamycin [K, 30 µg], gentamicin [GEM, 10 µg], and streptomycin [S, 10 µg]), phenicols (chloramphenicol [C, 30 µg]), sulfonamides (sulfisoxazole [G, 250 µg]), tetracyclines (tetracycline [Te, 30 µg]), and dihydrofolate reductase inhibitors (trimethoprim [TMP, 5 µg]). Resistance breakpoints used for this test were those defined by the CLSI (2007). To facilitate data analysis, isolates classified as intermediate were considered susceptible. E. coli ATCC 25922 was used as the quality control strain.
Minimum inhibitory concentrations (MICs) of nalidixic acid (Sigma Chemical Co., St Louis, Missouri, USA), ciprofloxacin (Sigma Chemical Co.), and levofloxacin (Bayer, Wuppertal, Germany) were determined by the agar dilution method according to the CLSI (Citation2006) standards. Interpretive criteria for evaluating susceptibility used in this study were those established by the CLSI (Citation2007). MIC breakpoints used to determine susceptibility (≤) and resistance (≥) to nalidixic acid, ciprofloxacin, and levofloxacin were 16 and 32 µg/ml, 1 and 4 µg/ml, and 2 and 8 µg/ml, respectively. E. coli ATCC 25922 was used as the quality control strain.
Detection of antimicrobial resistance genes in PMQR-positive isolates
Screening for various antimicrobial resistance genes in the PMQR-positive isolates was carried out with a PCR assay. DNA was extracted by boiling as described above. Genes encoding β-lactam resistance (bla TEM, bla CTX-M, bla SHV, bla OXA, and bla CMY) were detected using previously described primers and protocol (Dale et al., Citation1985; Perez-perez & Hanson Citation2002; Monstein et al., Citation2007). Genes conferring resistance to chloramphenicol (cat, cmlA, and floR; Guerra et al., Citation2004; Saenz et al., Citation2004), tetracycline (tetA, tetB, tetC, tetD, tetE, and tetG; Zhao & Aoki, Citation1992; Guardabassi et al., Citation2000; Guillaume et al., Citation2000), aminoglycoside (strA-B and aadA; Sunde & Norström, Citation2005), and sulfonamide (sul1 and sul2; Maynard et al., Citation2003) were also detected as previously described. Both strands of the PCR products were sequenced (Bioneer Corporation) and analysed as described above.
Transfer of PMQR genes
Transferability of the PMQR genes was examined by performing conjugation experiments using the liquid mating method. Cultures of donor (PMQR-positive isolates) and recipient (sodium azide-resistant E. coli J53) cells were incubated with shaking at 37°C in logarithmic phase, and then 0.5 ml of both cultures was added to 4 ml fresh Tryptic soy broth (Difco Laboratories) and incubated overnight without shaking at 37°C. The transconjugants were selected on Trypticase soy agar (Difco Laboratories) plates with sodium azide (100 µg/ml; Sigma Chemical Co.) to inhibit donor, and ampicillin (10 µg/ml; Sigma Chemical Co.) and streptomycin (10 µg/ml; Sigma Chemical Co.) to inhibit recipient. Antimicrobial susceptibility and MICs of the transconjugants were evaluated, and all transconjugants were screened for PMQR genes and antimicrobial resistance genes as described above.
PCR-based replicon typing of PMQR-positive isolates
To identify the incompatibility group of plasmids in PMQR-positive isolates, a PCR-based Inc/rep typing was performed using primers and protocols described by Carattoli et al. (Citation2005). Eighteen primer pairs were used to perform five multiplex and three simplex PCRs, which recognized FIA, FIB, FIC, HI1, HI2, I1-Iγ, L/M, N, P, W, T, A/C, K, B/O, X, Y, F, and FIIA. Both strands of the PCR products were sequenced (Bioneer Corporation) and analysed as described above.
Plasmid preparation and Southern hybridization of PMQR-positive isolates
Plasmid DNA was extracted from each PMQR-positive isolate and their corresponding transconjugants using the method previously described by Kado & Liu (Citation1981) with small modifications. The DNA was separated by electrophoresis in 0.7% agarose gels, and the approximate size of each plasmid with PMQR genes was determined according to the BAC-Tracker supercoiled DNA ladder (Epicentre Biotechnologies, Madison, Wisconsin, USA). For the hybridization assays, the extracted plasmids were transferred to positively charged nylon membranes (Roche Diagnostics GmbH, Mannheim, Germany) by capillary transfer. Probes specific for detection of qnrB4 and qnrS1 genes were prepared with a digoxigenin (DIG) labelling kit (Roche Diagnostics GmbH) according to the manufacturer's instructions. After hybridization with the qnrB4 and qnrS1 probes, hybridized DNA was detected using a DIG DNA labelling and detection kit (Roche Diagnostics GmbH). The restriction pattern of plasmid DNAs was evaluated by restricting with HindIII restriction enzyme (Bioneer Corporation).
Pulsed-field gel electrophoresis analysis of PMQR-positive isolates.
To investigate the clonal relationships among Salmonella isolates harbouring qnr genes, pulsed-field gel electrophoresis (PFGE) analysis was carried out as previously described by Ribot et al. (Citation2006). Briefly, after growth on Trypticase soy agar plates at 37°C for 18 to 20 h, genomic DNAs were digested with 50 u XbaI (Promega, Madison, Wisconsin, USA) for 2 h and separated on a 1.0% agarose gel using a CHEF-Mapper (Bio-Rad laboratories, Hercules, California, USA) in 0.5× TBE buffer (0.1 M Tris, 1 M boric acid, and 0.2 mM ethylenediamine tetraacetic acid) with recirculation at 14°C. Pulse times for the 18-h run at 6.0 V/cm included an initial switch time of 2.2 sec and a final switch time of 63.8 sec. Images of the gels were analysed using Bionumerics software (Applied Maths, Sint-Martens-Latem, Belgium), and a dendrogram of the PFGE patterns was generated based on Dice coefficients of similarity using the unweighted-pair group method with average linkages.
Results
Detection of PMQR genes
Detection of the PMQR genes in 185 Salmonella spp. isolated from poultry are shown in . The PMQR genes were detected in six (3.2%) out of 185 Salmonella isolates. Among the six PMQR-positive isolates, two Salmonella Senftenberg (SS84 and SS87) obtained from different chicken hatcheries (SK and NS companies) in 2002 carried the qnrB4 gene. One Salmonella Enteritidis (SE20) isolate collected from a broiler farm (BS company) in 2003 as well as two Salmonella Virchow (SV97 and SV99) and one Salmonella Heidelberg (SH52) isolate collected from the same poultry slaughterhouse (HL company) in 2002 carried the qnrS1 gene. All of the isolates tested were negative for the qnrA, qnrC, qnrD, qepA or aac(6′)-Ib-cr gene.
Table 2. PMQR genes, QRDR mutations, fluoroquinolone MICs, and plasmid analysis for six PMQR-positive Salmonella isolates, their transconjugants, and the recipient strain.
Mutations in the DNA gyrase and topoisomerase IV genes of the six PMQR-positive isolates
Mutations associated with fluoroquinolone resistance in the DNA gyrase and topoisomerase IV genes from the six PMQR-positive isolates are presented in . One isolate (SV97) possessed a mutation in gyrA at codon 83 that substituted phenylalanine for serine. None of the isolates had any mutations in the gyrB, parC, or parE genes.
MICs of the fluoroquinolones, and patterns of antimicrobial resistance and resistance genes among the six PMQR-positive isolates
MICs of the three fluoroquinolones for the six PMQR-positive isolates are shown in . The two qnrB4-positive isolates were susceptible to all of the three fluoroquinolones. Among the four qnrS1-positive isolates, three were non-susceptible (intermediate or resistant) to nalidixic acid (MIC ≥256 µg/ml), ciprofloxacin (MIC 2 µg/ml), and levofloxacin (MIC 4 µg/ml). Patterns of antimicrobial resistance and the corresponding genes that confer resistance against 17 additional non-quinolone antimicrobial agents are presented in . All PMQR-positive isolates were resistant to six or more antimicrobial agents (ampicillin, streptomycin, sulfisoxazole, trimethoprim, and tetracycline), but susceptible to cefotaxime, aztreonam, cefepime, and amikacin. Presence of resistance genes corresponding to the phenotypes was also detected in all isolates.
Transferability of quinolone resistance and plasmid analysis of the six PMQR-positive isolates
Results of the conjugation experiments and plasmid analysis of the six PMQR-positive isolates are presented in and along with and . All six PMQR-positive isolates transferred genes conferring quinolone resistance to sodium azide-resistant E. coli J53. Two isolates harbouring the qnrB4 gene possessed four small plasmids less than 8 kb in size. Four isolates containing the qnrS1 gene also possessed large plasmids with sizes ranging from 38 to 130 kb. Hybridization assays revealed that qnrB4 and qnrS1 genes were located on plasmids less than 8 kb and approximately 70 kb in size, respectively. As the results of plasmid fingerprinting performed with the HindIII restriction enzyme for transconjugants, three (TSE20, TSV97 and TSV99) of four qnrS1-positive transconjugants had an identical pattern, whereas one had a unique restriction pattern. Two transconjugants carrying the qnrB4 gene (TSS84 and TSS87) also had an identical pattern. PCR-based replicon typing of the plasmid incompatibility groups showed that the plasmids carrying qnrS1 belonged to the IncN. However, none of the replicon types of plasmid incompatibility group was detected from the plasmids carrying the qnrB4 gene. MICs of nalidixic acid of 8 to 16 µg/ml for the transconjugants increased two-fold to four-fold compared with those for recipient strain. MICs of ciprofloxacin and levofloxacin of 0.06 to 0.125 µg/ml and 0.25 to 0.5 µg/ml, respectively, for the transconjugants were eight-fold to 16-fold higher than those for the recipient strain. Resistance to other antimicrobial agents such as ampicillin, streptomycin, sulfisoxazole, trimethoprim, and tetracycline were co-transferred along with the associated resistance genes to the recipient strain.
Figure 1. Plasmids DNAs of qnrB-positive Salmonella isolates, their transconjugants, and positive control strain (1a) and southern hybridization of plasmid DNAs with qnrB-specific probe (1b). Lanes 1 and 7, BAC-Tracker Supercoiled DNA ladder (Epicentre Biotechnologies, Madison, Wisconsin, USA) used as a negative control and a reference for estimation of plasmid size; lane 2, E. coli, as a qnrB-positive control; lane 3, S. Senftenberg (SS84); lane 4, TSS84 (transconjugant); lane 5, S. Senftenberg (SS87); lane 6, TSS87.
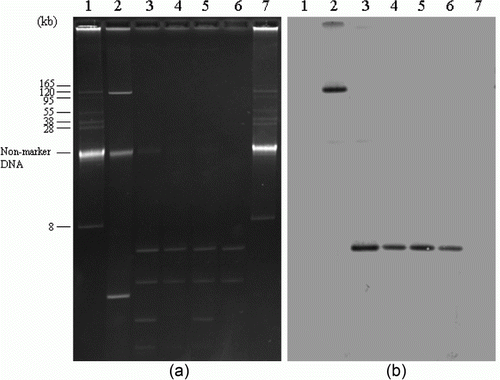
Figure 2. Plasmids DNAs of qnrS-positive Salmonella isolates, their transconjugants, and positive control strain (2a) and Southern hybridization of plasmid DNAs with qnrS-specific probe (2b). Lanes 1 and 11, BAC-Tracker Supercoiled DNA ladder (Epicentre Biotechnologies, Madison, Wisconsin, USA) used as a negative control and a reference for estimation of plasmid size; lane 2, E. coli, as qnrS-positive control; lane 3, S. Enteritidis (SE20); lane 4, TSE20 (transconjugant); lane 5, S. Heidelberg (SH52); lane 6, TSH52; lane 7, S. Virchow (SV97); lane 8, TSV97; lane 9, S. Virchow (SV99); lane 10, TSV99.
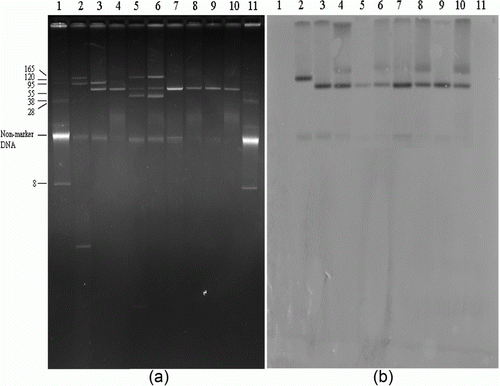
Table 3. Patterns of antimicrobial resistance and resistance genes in the six PMQR-positive Salmonella isolates and their transconjugants.
PFGE analysis of the six PMQR-positive isolates
Clonal relatedness of the six PMQR-positive isolates was determined by PFGE (). A similarity threshold >85% defined clusters. Profiles of the two S. Senftenberg isolates carrying the qnrB4 gene (SS84 and SS87) obtained from different hatcheries had an 89% similarity. Likewise, the qnrS1-positive S. Virchow isolates (SV97 and SV99) had PFGE profiles that were 98% similar.
Discussion
In the present study, six of tested 185 Salmonella isolated from poultry were positive for either qnrB4 or qnrS1. Salmonella serotypes detected in the qnr gene were S. Enteritidis, S. Heidelberg, S. Senftenberg, and S. Virchow. In Salmonella, the qnr gene was initially detected by Hopkins et al. (Citation2007) in six (two Salmonella Stanley, one Salmonella Typhimurium, two S. Virchow, and one Salmonella Virginia) out of 118 Salmonella isolates collected from human clinical specimens between 1993 and 2005 in the UK. Subsequently, various types of PMQR genes have been detected in clinical Salmonella isolates from humans around the world including France, the USA, and China (Cattoir et al., Citation2007; Cavaco et al., Citation2009; Cui et al., Citation2009). Additionally, qnr genes have been found in Salmonella recovered from animals including two S. Typhimurium with qnrS1 in Japan (Asai et al., Citation2010) as well as one Salmonella Infantis and two Salmonella Uganda with qnrB19 in Colombia (Karczmarczyk et al., Citation2010). In Korea, Jeong et al. (Citation2011) initially found the qnr gene in six (2.1%) out of 284 Salmonella isolates collected from human clinical specimens in 2008. Although Tamang et al. (2011) detected the aac(6′)-Ib-cr gene in four (0.6%) out of 692 Salmonella isolates of animal origin, they failed to find the qnr gene. This is the first report of the qnr genes detected in Salmonella isolates from poultry in Korea. Furthermore, our data demonstrated how the qnr gene is distributed along the poultry production chain in Korea given that we examined Salmonella spp. isolated from 22 hatcheries, 86 poultry farms, and five poultry slaughterhouses located throughout the country.
Many investigators have reported that the prevalence of the PMQR genes varies from less than 1% to more than 50% all over the world depending on resistance mechanisms of the organism (Rodríguez-Martínez et al., Citation2011; Poirel et al., Citation2012). In the present study, qnrB4 and qnrS1 genes were identified in 1.1% (two isolates) and 2.2% (four isolates) of the 185 Salmonella isolates from poultry, respectively. Similar to the present study, Cerquetti et al. (Citation2009) identified only one qnrS1-positive isolate (1.4%) out of the 73 E. coli isolates from chickens in Italy. In contrast, Huang et al. (Citation2009) reported that a high prevalence (9.8%) of qnr was observed in chicken E. coli isolates in China. In Korea, Kim et al. (Citation2010) found the qnrB4 gene in four (5.8%) out of 69 extended-spectrum β-lactamase (ESBL)-producing E. coli isolates from human clinical specimens and the qnr gene in 17 (40.5%) out of 42 ESBL-producing K. pneumoniae isolates.
In the present study, we evaluated mutations associated with fluoroquinolone resistance in the QRDRs. No mutations in gyrB, parC, or parE were detected in any of the isolates. A mutation in gyrA that changed the 83rd amino acid from a serine to phenylalanine was identified in the SV97 isolate (S. Virchow) obtained from chilling water in the poultry slaughterhouse. However, this mutation was not found in the SV99 isolate that had patterns of antimicrobial resistance, PFGE results, and a plasmid restriction pattern similar to or identical to those of SV97 that was isolated from carcass in the same poultry slaughterhouse. According to some reports (Asai et al., Citation2010; Jeong et al., Citation2011; Tamang et al., Citation2011), this amino acid substitution suppresses susceptibility to fluoroquinolones, but the chromosomal mutation of gyrA found among the QRDRs in the present study did not reduce fluoroquinolone susceptibility. It is considered necessary to analyse by further investigations the cause of this non-role in quinolone resistance despite having a mutation in gyrA.
In the present study, we confirmed that the presence of qnr genes increased fluoroquinolone MICs. In general, the expression of qnr genes increases the MICs of nalidixic acid and ciprofloxacin by two-fold to eight-fold and eight-fold to 32-fold, respectively (Strahilevit et al., Citation2009). According to the reports by Martínez-Martínez et al. (Citation1998), Gay et al. (Citation2006), and Cattoir et al. (Citation2007), MICs of nalidixic acid increase eight-fold in transconjugants compared with recipient strain, which is a slightly higher rate compared with the one from our study. Martínez-Martínez et al. (Citation1998) and Cattoir et al. (Citation2007) also reported an eight-fold or 64-fold increase in ciprofloxacin MICs, similar to or higher than our figures. We discovered that transconjugants receiving the qnrS1 gene in our study had slightly higher or similar levels of quinolone resistance compared with those receiving the qnrB4 gene, which was similar to the results of Ma et al. (Citation2009). Bacteria with PMQR genes are not always resistant to fluoroquinolones due to the acquisition of low-level quinolone resistance. However, it is thought that when plasmids harbouring qnr genes are transferred to various bacterial species, resistance to fluoroquinolone rapidly increases.
Resistance plasmids with various Inc groups have been reported to be involved in the transmission of the qnrS1 gene among Enterobacteriaceae (Carattoli, Citation2009). Among them, qnrS1-positive IncN plasmids were identified in S. Virchow from human and animal sources in the UK in 2004 and 2005, causing an outbreak associated with imported cooked meat from Thailand (Hopkins et al., Citation2007), and were detected in Salmonella Kentucky and Salmonella Saintpaul from human sources in the Netherlands (Garcia-Fernandez et al., Citation2009). IncN plasmids are common in enterobacterial pathogens from animals, but are rarer in bacteria from humans (Johnson et al., Citation2007). It could be hypothesized that IncN plasmids could have acquired the qnrS1 gene in animals.
In general, isolates with qnr genes express β-lactamases such as ESBLs (Rodríguez-Martínez et al., Citation2011). Avsaroglu et al. (Citation2007) detected qnr genes and the bla TEM-1 gene together in Salmonella isolated from food of avian origin in Turkey. In the present study, bla TEM-1 genes were detected in all the qnr-positive isolates. Although other researchers in Korea have reported that qnr-positive isolates possess genes encoding ESBLs such as bla CTX-M, bla SHV, and bla DHA (Pai et al., Citation2007; Tamang et al., Citation2008), these genes were not detected in our study. This result can be explained by the fact that resistance to these antimicrobial agents had not developed because third-generation and fourth-generation cephalosporins, which affect microorganisms expressing ESBL genes, are not used for poultry.
Plasmids with PMQR genes have various sizes ranging from 2.7 to 320 kb. In addition, it is known that most plasmids containing the qnrS gene are relatively smaller and non-conjugative compared with ones containing qnrA and qnrB genes, and are more likely to be found in Salmonella (Kehrenberg et al., Citation2007; Garcia-Fernandez et al., Citation2009). In our study, however, plasmids with qnrS1 gene (about 70 kb in size) were relatively larger than ones with the qnrB4 gene (less than 8 kb). Differences between the findings from previous studies and our study may relate to the smaller size of plasmids harbouring the qnrB gene that were detected in the present study, their different origin, or their different genetic environments. Rodríguez-Martínez et al. (Citation2011) reported that qnrA-positive and qnrB-positive plasmids often harbour genes that confer resistance to β-lactams, aminoglycosides, chloramphenicol, tetracycline, sulfonamides, trimethoprim, and rifampin whereas qnrS-positive plasmids do not. In contrast, we thought that the qnrS1-positive plasmids examined in this investigation contained other antibiotic resistance genes whereas the qnrB4-positive plasmids did not. Further study will be needed to analyse the different genetic environments of plasmids harbouring the qnrB or qnrS gene.
Epidemiological relationships among the qnr-positive isolates were examined by plasmid and PFGE analysis in the present study. Two S. Senftenberg isolates with qnrB4 gene obtained from different hatcheries and two S. Virchow isolates with qnrS1 gene acquired from the same poultry slaughterhouse had identical plasmid profiles or restriction patterns for strains of the same serotype. In addition, the PFGE patterns for strains of the same serotype were similar. It is believed that these bacteria are mutually transmitted via various vectors. On the other hand, the plasmid carrying the qnrS1 gene in S. Virchow was the same plasmid restriction pattern and Inc group as the one carrying the qnrS1 gene in S. Enteritidis isolated from the broiler farm. This may have resulted from plasmid transfer between Salmonella of different serotypes. Our finding underscores the fact that qnr genes are a significant public health concern because they are transferred via plasmids among various bacterial species.
In conclusion, we have reported the first incidence of qnr genes in Salmonella spp. isolated from the poultry production chain in Korea. The existence of qnr genes among Salmonella isolates recovered from poultry may be a serious public health concern because these can be transmitted to humans via poultry products or by contact with poultry, and can rapidly increase fluoroquinolone resistance in various bacterial species through the transfer of plasmids harbouring qnr genes. To protect the public health, continuous monitoring and surveillance systems are necessary to prevent the spread of PMQR genes among Salmonella spp. that can be transmitted from poultry to humans.
Acknowledgements
This research was supported by Kyungpook National University Research Fund, 2012.
References
- Asai , T. , Sato , C. , Masani , K. , Usui , M. , Ozawa , M. , Ogino , T. , Aoki , H. , Sawada , T. , Izumiya , H. and Watanabe , H. 2010 . Epidemiology of plasmid-mediated quinolone resistance in Salmonella enterica serovar Typhimurium isolates from food-producing animals in Japan . Gut Pathology , 2 : 17 doi: 10.1186/1757-4749-2-17
- Avsaroglu , M.D. , Helmuth , R. , Junker , E. , Hertwig , S. , Schroeter , A. , Akcelik , M. , Bozoglu , F. and Guerra , B. 2007 . Plasmid-mediated quinolone resistance conferred by qnrS1 in Salmonella enterica serovar Virchow isolated from Turkish food of avian origin . Journal of Antimicrobial Chemotherapy , 60 : 1146 – 1150 . doi: 10.1093/jac/dkm352
- Carattoli , A. 2009 . Resistance plasmid families in Enterobacteriaceae . Antimicrobial Agents and Chemotherapy , 53 : 2227 – 2238 . doi: 10.1128/AAC.01707-08
- Carattoli , A. , Bertini , A. , Villa , L. , Falbo , V. , Hopkins , K.L. and Threlfall , E.J. 2005 . Identification of plasmids by PCR-based replicon typing . Journal of Microbiological Methods , 63 : 219 – 228 . doi: 10.1016/j.mimet.2005.03.018
- Cattoir , V. , Weill , F.X. , Poirel , L. , Fabre , L. , Soussy , C.J. and Nordmann , P. 2007 . Prevalence of qnr genes in Salmonella in France . Journal of Antimicrobial Chemotherapy , 59 : 751 – 754 . doi: 10.1093/jac/dkl547
- Cavaco , L.M. , Hasman , H. , Xia , S. and Aarestrup , F.M. 2009 . qnrD, a novel gene conferring transferable quinolone resistance in Salmonella enterica serovar Kentucky and Bovismorbificans strains of human origin . Antimicrobial Agents and Chemotherapy , 53 : 603 – 608 . doi: 10.1128/AAC.00997-08
- Centers for Disease Control and Prevention (CDC) . 2011 . Surveillance for foodborne disease outbreaks-United States, 2008 . Morbidity and Mortality Weekly Report , 60 , 1197 – 1202 .
- Cerquetti , M. , Garcia-Fernandez , A. , Giufre , M. , Fortini , D. , Accogli , M. , Graziani , C. , Luzzi , I. , Caprioli , A. and Carattoli , A. 2009 . First report of plasmid-mediated quinolone resistance determinant qnrS1 in an Escherichia coli strain of animal origin in Italy . Antimicrobial Agents and Chemotherapy , 53 : 3112 – 3114 . doi: 10.1128/AAC.00239-09
- CLSI . 2006 . Methods for Dilution Antimicrobial Susceptibility Tests for Bacteria that Grow Aerobically 7th edn . Approved standard M07-A7 . Wayne , PA : Clinical and Laboratory Standards Institute .
- CLSI . 2007 . Performance Standards for Antimicrobial Susceptibility Testing; 17th Informational Supplement . M100-S17 . Wayne , PA : Clinical and Laboratory Standards Institute .
- Cui , S. , Li , J. , Sun , Z. , Hu , C. , Jin , S. , Li , F. , Guo , Y. , Ran , L. and Ma , Y. 2009 . Characterization of Salmonella enterica isolates from infants and toddlers in Wuhan, China . Journal of Antimicrobial Chemotherapy , 63 : 87 – 94 . doi: 10.1093/jac/dkn452
- Dale , J.W. , Godwin , D. , Mossakowska , D. , Stephenson , P. and Wall , S. 1985 . Sequence of the OXA-2 β-lactamase: comparison with other penicillin-reactive enzymes . FEBS Letters , 191 : 39 – 44 . doi: 10.1016/0014-5793(85)80989-3
- Difco Laboratories . 1977 . Serological Identification of Salmonella . Detroit , MI : Difco Laboratories .
- Everett , M.J. , Jin , Y.F. , Ricci , V. and Piddock , L.J. 1996 . Contribution of individual mechanisms to fluoroquinolone resistance in 36 Escherichia coli strains isolated from humans and animals . Antimicrobial Agents and Chemotherapy , 40 : 2380 – 2386 .
- Gagliotti , C. , Buttazzi , R. , Sforza , S. and Moro , M.L. 2008 . Resistance to fluoroquinolones and treatment failure/short-term relapse of community-acquired urinary tract infections caused by Escherichia coli . Journal of Infection , 57 : 179 – 184 . doi: 10.1016/j.jinf.2008.07.004
- Garcia-Fernandez , A. , Fortini , D. , Veldman , K. , Mevius , D. and Carattoli , A. 2009 . Characterization of plasmids harbouring qnrS1, qnrB2 and qnrB19 genes in Salmonella . Journal of Antimicrobial Chemotherapy , 63 : 274 – 281 . doi: 10.1093/jac/dkn470
- Gay , K. , Robicsek , A. , Strahilevitz , J. , Park , C.H. , Jacoby , G. , Barrett , T.J. , Medalla , F. , Chiller , T.M. and Hooper , D.C. 2006 . Plasmid-mediated quinolone resistance in non-Typhi serotypes of Salmonella enterica . Clinical Infectious Diseases , 43 : 297 – 304 . doi: 10.1086/505397
- Guardabassi , L. , Dijkshoorn , L. , Collard , J.M. , Olsen , J.E. and Dalsgaard , A. 2000 . Distribution and in-vitro transfer of tetracycline resistance determinants in clinical and aquatic Acinetobacter strains . Journal of Medical Microbiology , 49 : 29 – 36 .
- Guerra , B. , Junker , E. , Miko , A. , Helmuth , R. and Mendoza , M.C. 2004 . Characterization and localization of drug resistance determinants in multi-resistant, integron carrying Salmonella enterica serotype Typhimurium strains . Microbial Drug Resistance , 10 : 83 – 91 . doi: 10.1089/1076629041310136
- Guillaume , G. , Verbrugge , D. , Chasseur-Libotte , M. , Moens , W. and Collard , J. 2000 . PCR typing of tetracycline resistance determinants (Tet A-E) in Salmonella enterica serotype Hadar and in the microbial community of activated sludges from hospital and urban wastewater treatment facilities in Belgium . FEMS Microbiology Ecology , 32 : 77 – 85 .
- Hopkins , K.L. , Davies , R.H. and Threlfall , E.J. 2005 . Mechanisms of quinolone resistance in Escherichia coli and Salmonella: recent developments . International Journal of Antimicrobial Agents , 25 : 358 – 373 . doi: 10.1016/j.ijantimicag.2005.02.006
- Hopkins , K.L. , Wootton , L. , Day , M.R. and Threlfall , E.J. 2007 . Plasmid-mediated quinolone resistance determinant qnrS1 found in Salmonella enterica strains isolated in the UK . Journal of Antimicrobial Chemotherapy , 59 : 1071 – 1075 . doi: 10.1093/jac/dkm081
- Huang , S.Y. , Dai , L. , Xia , L.N. , Du , X.D. , Qi , Y.H. , Liu , H.B. , Wu , C.M. and Shen , J.Z. 2009 . Increased prevalence of plasmid-mediated quinolone resistance determinants in chicken Escherichia coli isolates from 2001 to 2007 . Foodborne Pathogens and Disease , 6 : 1203 – 1209 . doi: 10.1089/fpd.2009.0348
- Jacoby , G.A. 2005 . Mechanism of resistance to quinolones . Clinical Infectious Diseases , 41 : S120 – S126 . doi: 10.1086/428052
- Jeong , H.S. , Kim , J.A. , Shin , J.H. , Chang , C.L. , Jeong , J. , Cho , J.-H. , Kim , M.-N. , Kim , S.J. , Kim , Y.R. , Lee , C.H. , Lee , K.W. , Lee , M.A. , Lee , W.G. , Shin , J.H. and Lee , J.N. 2011 . Prevalence of plasmid-mediated quinolone resistance and mutations in the gyrase and topoisomerase IV genes in Salmonella isolated from 12 tertiary-care hospitals in Korea . Microbial Drug Resistance , 17 : 551 – 557 . doi: 10.1089/mdr.2011.0095
- Johnson , T.J. , Wannemuehler , Y.M. , Johnson , S.J. , Logue , C.M. , White , D.G. , Doetkott , C. and Nolan , L.K. 2007 . Plasmid replicon typing of commensal and pathogenic Escherichia coli isolates . Applied and Environmental Microbiology , 73 : 1976 – 1983 . doi: 10.1128/AEM.02171-06
- Kado , C.I. and Liu , S.T. 1981 . Rapid procedure for detection and isolation of large and small plasmids . Journal of Bacteriology , 145 : 1365 – 1373 .
- Karczmarczyk , M. , Martins , M. , McCusker , M. , Mattar , S. , Amaral , L. , Leonard , N. , Aarestrup , F.M. and Fanning , S. 2010 . Characterization of antimicrobial resistance in Salmonella enterica food and animal isolates from Colombia: identification of a qnrB19-mediated quinolone resistance marker in two novel serovars . FEMS Microbiology Letters , 313 : 10 – 19 . doi: 10.1111/j.1574-6968.2010.02119.x
- Kehrenberg , C. , Hopkins , K.L. , Threlfall , E.J. and Schwarz , S. 2007 . Complete nucleotide sequence of a small qnrS1-carrying plasmid from Salmonella enterica subsp. enterica Typhimurium DT 193 . Journal of Antimicrobial Chemotherapy , 60 : 903 – 905 . doi: 10.1093/jac/dkm283
- Kim , M.H. , Lee , H.J. , Park , K.S. and Suh , J.T. 2010 . Molecular characteristics of extended spectrum beta-lactamases in Escherichia coli and Klebsiella pneumoniae and the prevalence of qnr in extended spectrum beta-lactamase isolates in a tertiary care hospital in Korea . Yonsei Medical Journal , 51 : 768 – 774 . doi: 10.3349/ymj.2010.51.5.768
- Ma , J. , Zeng , Z. , Chen , Z. , Xu , X. , Wang , X. , Deng , Y. , Lü , D. , Huang , L. , Zhang , Y. , Liu , J. and Wang , M. 2009 . High prevalence of plasmid-mediated quinolone resistance determinants qnr, aac(6′)-Ib-cr, and qepA among ceftiofur-resistant Enterobacteriaceae isolates from companion and food-producing animals . Antimicrobial Agents and Chemotherapy , 53 : 519 – 524 . doi: 10.1128/AAC.00886-08
- Marcus , R. 2008 . New information about pediatric foodborne infections: the view from FoodNet . Current Opinion in Pediatrics , 20 : 79 – 84 . doi: 10.1097/MOP.0b013e3282f43067
- Martínez-Martínez , L. , Pascual , A. and Jacoby , G.A. 1998 . Quinolone resistance from a transferable plasmid . Lancet , 351 : 797 – 799 . doi: 10.1016/S0140-6736(97)07322-4
- Maynard , C. , Fairbrother , J.M. , Bekal , S. , Sanschagrin , F. , Levesque , R.C. , Brousseau , R. , Masson , L. , Lariviere , S. and Harel , J. 2003 . Antimicrobial resistance genes in enterotoxigenic Escherichia coli O149:K91 isolates obtained over a 23-year period from pigs . Antimicrobial Agents and Chemotherapy , 47 : 3214 – 3221 . doi: 10.1128/AAC.47.10.3214-3221.2003
- Monstein , H.-J. , Östholm-Balkhed , Å. , Nilsson , M.V. , Nilsson , M. , Dornbusch , K. and Nilsson , L.E. 2007 . Multiplex PCR amplification assay for the detection of blaSHV, blaTEM and blaCTX-M genes in Enterobacteriaceae . APMIS , 115 : 1400 – 1408 . doi: 10.1111/j.1600-0463.2007.00722.x
- Pai , H. , Seo , M.-R. and Choi , T.Y. 2007 . Association of QnrB determinants and production of extended-spectrum β-lactamase or plasmid-mediated AmpC β-lactamase in clinical isolates of Klebsiella pneumoniae . Antimicrobial Agents and Chemotherapy , 51 : 366 – 368 . doi: 10.1128/AAC.00841-06
- Park , C.H. , Robicsek , A. , Jacoby , G.A. , Sahm , D. and Hooper , D.C. 2006 . Prevalence in the United States of aac(6′)-Ib-cr encoding a ciprofloxacin-modifying enzyme . Antimicrobial Agents and Chemotherapy , 50 : 3953 – 3955 . doi: 10.1128/AAC.00915-06
- Perez-Perez , F.J. and Hanson , N.D. 2002 . Detection of plasmid-mediated AmpC β-lactamase genes in clinical isolates by using multiplex PCR . Journal of Clinical Microbiology , 40 : 2153 – 2162 . doi: 10.1128/JCM.40.6.2153-2162.2002
- Poirel , L. , Cattoir , V. and Nordmann , P. 2012 . Plasmid-mediated quinolone resistance; interactions between human, animal, and environmental ecologies . Frontiers in Microbiology , 3 : 24 doi: 10.3389/fmicb.2012.00024
- Ribot , E.M. , Fair , M.A. , Gautom , R. , Cameron , D.N. , Hunter , S.B. , Swaminathan , B. and Barrett , T.J. 2006 . Standardization of pulsed-field gel electrophoresis protocols for the subtypong of Escherichia coli O157:H7, Salmonella, and Shigella of PulseNet . Foodborne Pathogens and Disease , 3 : 59 – 67 . doi: 10.1089/fpd.2006.3.59
- Robicsek , A. , Strahilevitz , J. , Jacoby , G.A. , Macielag , M. , Abbanat , D. , Park , C.H. , Bush , K. and Hooper , D.C. 2006a . Fluoroquinolone-modifying enzyme: a new adaptation of a common aminoglycoside acetyltransferase . Nature Medicine , 12 : 83 – 88 . doi: 10.1038/nm1347
- Robicsek , A. , Strahilevitz , J. , Sahm , D.F. , Jacoby , G.A. and Hooper , D.C. 2006b . qnr prevalence in ceftazidime-resistant Enterobacteriaceae isolates from the United States . Antimicrobial Agents and Chemotherapy , 50 : 2872 – 2874 . doi: 10.1128/AAC.01647-05
- Rodríguez-Martínez , J.M. , Cano , M.E. , Velasco , C. , Martínez-Martínez , L. and Pascual , Á. 2011 . Plasmid-mediated quinolone resistance: an update . Journal of Infection and Chemotherapy , 17 : 149 – 182 . doi: 10.1007/s10156-010-0120-2
- Saenz , Y. , Brinas , L. , Dominguez , E. , Ruiz , J. , Zarazaga , M. , Vila , J. and Torres , C. 2004 . Mechanisms of resistance in multiple-antibiotic-resistant Escherichia coli strains of human, animal, and food origins . Antimicrobial Agents and Chemotherapy , 48 : 3996 – 4001 . doi: 10.1128/AAC.48.10.3996-4001.2004
- Strahilevitz , J. , Jacoby , G.A. , Hooper , D.C. and Robicsek , A. 2009 . Plasmid-mediated quinolone resistance: a multifaceted threat . Clinical Microbiology Reviews , 22 : 664 – 689 . doi: 10.1128/CMR.00016-09
- Sunde , M. and Norström , M. 2005 . The genetic background for streptomycin resistance in Escherichia coli influences the distribution of MICs . Journal of Antimicrobial Chemotherapy , 56 : 87 – 90 . doi: 10.1093/jac/dki150
- Tamang , M.D. , Nam , H.M. , Kim , A.R. , Lee , H.S. , Kim , T.S. , Kim , M.J. , Jang , G.C. , Jung , S.C. and Lim , S.K. 2011 . Prevalence and mechanisms of quinolone resistance among selected nontyphoid Salmonella isolated from food animals and humans in Korea . Foodborne Pathogens and Disease , 8 : 1199 – 1206 . doi: 10.1089/fpd.2011.0899
- Tamang , M.D. , Seol , S.Y. , Oh , J.-Y. , Kang , H.Y. , Lee , J.C. , Lee , Y.C. , Cho , D.T. and Kim , J. 2008 . Plasmid-mediated quinolone resistance determinants qnrA, qnrB, and qnrS among clinical isolates of Enterobacteriaceae in a Korean hospital . Antimicrobial Agents and Chemotherapy , 52 : 4159 – 4162 . doi: 10.1128/AAC.01633-07
- Wang , M. , Guo , Q. , Xu , X. , Wang , X. , Ye , X. , Wu , S. , Hooper , D.C. and Wang , M. 2009 . New plasmid-mediated quinolone resistance gene, qnrC, found in a clinical isolate of Proteus mirabilis . Antimicrobial Agents and Chemotherapy , 53 : 1892 – 1897 . doi: 10.1128/AAC.01400-08
- Yamane , K. , Wachino , J. , Suzuki , S. and Arakawa , Y. 2008 . Plasmid-mediated qepA gene among Escherichia coli clinical isolates from Japan . Antimicrobial Agents and Chemotherapy , 52 : 1564 – 1566 . doi: 10.1128/AAC.01137-07
- Yamane , K. , Wachino , J. , Suzuki , S. , Kimura , K. , Shibata , N. , Kato , H. , Shibayama , K. , Konda , T. and Arakawa , Y. 2007 . New plasmid-mediated fluoroquinolone efflux pump, QepA, found in an Escherichia coli clinical isolate . Antimicrobial Agents and Chemotherapy , 51 : 3354 – 3360 . doi: 10.1128/AAC.00339-07
- Zhao , J. and Aoki , T. 1992 . Nucleotide sequence analysis of the class G tetracycline resistance determinant from Vibrio anguillarum . Microbiology and Immunology , 36 : 1051 – 1060 .