Abstract
In recent years, special attention has been paid to real-time polymerase chain reaction (PCR) for avian metapneumovirus (AMPV) diagnosis, due to its numerous advantages over classical PCR. A new multiplex quantitative real-time reverse transcription-PCR (qRT-PCR) with molecular beacon probe assay, designed to target the SH gene, was developed. The test was evaluated in terms of specificity, sensitivity and repeatability, and compared with conventional RT nested-PCR based on the G gene. All of the AMPV subtype A and B strains tested were amplified and specifically detected while no amplification occurred with other non-target bird respiratory pathogens. The detection limit of the assay was 10−0.41 median infectious dose/ml and 101.15 median infectious dose/ml when the AMPV-B strain IT/Ty/B/Vr240/87 and the AMPV-A strain IT/Ty/A/259-01/03 were used, respectively, as templates. In all cases, the amplification efficiency was approximately 2 and the error values were <0.2. Standard curves, generated either using the serial dilution of an RNA suspension or RNA extracted from the serial dilution of titrated viral suspensions as templates, exhibited good linearity (R 2>0.9375) between crossing point values and virus quantities, making the assay herein designed reliable for quantification. When the newly developed qRT-PCR was compared with a conventional RT nested-PCR, it showed greater sensitivity with RNA extracted from both positive controls and from experimentally infected birds. This assay can be effectively used for the detection, identification, differentiation and quantitation of AMPV subtype A or subtype B to assist in disease diagnosis and to carry out rapid surveillance with high levels of sensitivity and specificity.
Introduction
The avian metapneumovirus (AMPV) is responsible for an acute and very contagious infection of the upper respiratory tract of turkeys and chickens (Gough & Jones, Citation2008). In both turkeys and chickens, a drop in egg production and impaired egg shell quality follow AMPV infection (Naylor & Jones, Citation1993; Cecchinato et al., Citation2012).
The AMPV can affect other avian species as well, such as guinea fowls (Kles et al., Citation1987), pheasants (Catelli et al., Citation2001) and ducks (Toquin et al., Citation1999).
The AMPV is a virus belonging to the Paramyxoviridae family; the genome is a non-segmented, negative-stranded RNA containing eight genes (3′-N-P-M-F-M2-SH-G-L-5′) (Easton et al., Citation2004). Four different subtypes are known: A, B, C and D (Gough & Jones, Citation2008). Subtypes A and B have an almost worldwide distribution while subtype C, after its appearance in the USA (Senne et al., Citation1997), has been sporadically reported in France (Toquin et al., Citation2006) and Korea (Lee et al., Citation2007). Subtype D was isolated once in France in the mid-1980s (Bayon-Auboyer et al., Citation2000) and has never been reported subsequently.
The diagnosis of this disease poses a challenge due to the mild or non-specific signs observed after infection in some avian species, and low AMPV multiplication rates in the host organism, thus making sampling for viral isolation difficult and a clinical diagnosis impossible. Moreover, viral isolation can be time consuming and requires additional investigation. Serology offers a faster solution, but it is not always trustworthy because AMPV genetic differences can hinder its sensitivity, and it gives retrospective information (Cook & Cavanagh, Citation2002). Molecular assays represent a fast, practical, specific and sensitive diagnostic technique.
Sequence analysis of AMPV subtype A, B, C and D genomes has enabled numerous general or subtype-specific reverse transcriptase-polymerase chain reaction (RT-PCR)-based diagnostic protocols to be designed. Primers directed to conserved regions of the N gene have been shown to successfully detect isolates from the A, B and C subtypes (Toquin et al., Citation1999) and subtype D (Bayon-Auboyer et al., Citation1999). Tests for subtypes A and B specifically based on the G gene have been shown to be sensitive and, due to sequence differences between subtypes, can readily differentiate between them (Naylor et al., Citation1997; Bayon-Auboyer et al., Citation1999; Hess et al., Citation2004). Other tests are based on F gene (Jing et al., Citation1993; Mase et al., Citation1996; Cecchinato et al., Citation2004a) or M gene sequences, the latter of which was used to develop RT-PCRs for AMPV subtype C-specific detection (Ali & Reynolds, Citation1999; Pedersen et al., Citation2000, Citation2001; Shin et al., Citation2000). However, for subtype A detection, higher sensitivity was seen in RT-PCRs designed on the F gene (Cecchinato et al., Citation2004a).
In recent years, special attention has been paid to real-time PCR, due to its numerous advantages over classical PCR. First of all, the sample analysis throughput is greater, it is faster, is less prone to contamination, makes quantification possible, and different studies have demonstrated its greater sensitivity in diagnosis when compared with conventional PCR assays. Real-time RT-PCR protocols for AMPV have been developed to detect the AMPV of subtype A (Ferreira et al., Citation2009) and subtype C (Velayudhan et al., Citation2005; Abbassi et al., Citation2007), and to detect and differentiate between subtypes A and B (Kwon et al., Citation2010) or any of the four AMPV subtypes (Guionie et al., Citation2007).
To improve AMPV molecular diagnosis, a new multiplex quantitative real-time RT-PCR (qRT-PCR) with a molecular beacon probe assay was developed in this study. The protocol, which allows the identification, differentiation and quantitation of AMPV subtypes A and B, was designed to target the SH gene, evaluated in terms of specificity, sensitivity and repeatability, and was compared with a conventional RT nested-PCR based on the G gene (Naylor et al., Citation1997).
Materials and Methods
Primer and probe design
Beacon Designer 7.0 software (PREMIER Biosoft International, Palo Alto, CA, USA) was used to design a set of primers (forward and reverse) based on a conservative region of the SH gene of AMPV subtypes A and B (). The sequences necessary for the primer and the molecular beacon probe design were obtained from GenBank or were previously reported (Catelli et al., Citation2010; Cecchinato et al., Citation2010; Lupini et al., Citation2011). A 200-nucleotide maximum distance was allowed between the primer pairs. Each primer was evaluated for GC content, secondary structures, primer dimers and 3′ end stability. Probe sequences were designed to target conserved genome regions within subtype A or subtype B. The regions targeted differed in AMPV-A and AMPV-B.
Table 1. Sequences of primers and probes used for reverse transcription copying and real-time PCR amplification.
Molecular beacons are single-stranded oligonucleotides that possess a stem-and-loop structure. The loop portion of the molecular beacons reports the presence of a specific complementary nucleic acid. The stem has five to seven base pairs, which are complementary. A fluorophore and a quencher are linked to the two ends of the stem. The stem keeps these two moieties in close proximity, causing the fluorescence of the fluorophore to be quenched by energy transfer. When the probe encounters a target DNA molecule, the molecular beacon undergoes a spontaneous conformational reorganization that forces the stem apart, leading to the restoration of fluorescence. The conformational state of a molecular beacon is thus directly reported by its fluorescence: in the closed state, the molecular beacon is not fluorescent; in the open state, when the fluorophore and the quencher molecules are apart, it emits intense fluorescence (Tan et al., Citation2000).
The probes were labelled with FAM or HEX dyes (), which can be differentiated by their maximum emission wavelength, allowing their use in duplex assays. A BLAST search of the NCBI nr database was carried out for each sequence in order to check the specificity. All probes and primers were synthesized by Integrated DNA Technologies® Inc. (Coralville, Iowa, USA).
Assay optimization
All reactions were carried out on LightCycler 480® (Roche Diagnostic, Marnes La Coquette, France) using a Superscript® III Platinum® One-Step Quantitative RT-PCR kit (Life Technologies, Carlsbad, California, USA). The primer and probe concentrations, annealing temperature and the length of the reverse transcriptase step were evaluated to determine the best efficiency (η) values using 10-fold serial dilutions of RNA extracted from one AMPV-A strain (IT/Ty/A/259-01/03) and two AMPV-B strains (IT/Ty/B/Vr240/87; IT/Ty/B/129-18/04) as templates.
Specificity
To determine the specificity of the assay, 20 AMPV strains, two of which belonged to subtype A, 17 to subtype B and one to subtype C (), along with other well-known pathogens causing respiratory infection in poultry, including Newcastle disease virus, infectious bronchitis virus, infectious laringotracheitis virus (two strains), Mycoplasma gallisepticum (three strains); Mycoplasma synoviae (three strains) and Ornithobacter rhinotracheale, were used as templates for the qRT-PCR.
Table 2. AMPV-A and AMPV-B strains used in this study and results of their analyses by qRT-PCR.
A comparison of crossing point (Cp) values obtained from dilutions of RNA of AMPV subtype A or subtype B, and from a mixture of RNA of subtype A + B dilutions, was also performed.
The RNA extracted from swabs collected from specific pathogen-free chickens and medium from non-infected tracheal organ cultures and VERO cells were used as negative controls.
RNA and DNA extraction
All RNA samples used in the present study were extracted using the QIAamp® Viral RNA Mini Kit (QIAGEN S.r.l., Milan, Italy) according to the manufacturer's instructions.
The DNA from the templates reported above was extracted using a QIAamp® DNA Mini Kit (QIAGEN S.r.l., Milan, Italy) according to the manufacturer's instructions.
Lower limit of detection of the assay
The lower limit of detection of the assay is the smallest amount of analyte in a sample that can be detected by a direct detection assay in at least 50% of the replicates for each dilution, in a dilution series of analyte in matrix (OIE, Citation2012). The limit of detection was evaluated using 10-fold serial dilutions of titrated virus suspensions of the IT/Ty/A/259-01/03 (AMPV-A) and IT/Ty/B/Vr240/87 (AMPV-B) strains. The IT/Ty/A/259-01/03 strain was titrated on VERO cells while the other strain was titrated on tracheal organ cultures (Catelli et al., Citation1998). Their titres were calculated using the Reed–Muench method (Reed & Muench, Citation1938). The IT/Ty/B/Vr240/87 strain had titres of 104.41 median tissue culture infectious dose (TCID50)/ml while the IT/Ty/A/259-01/03 strain had a titre of 105.15 TCID50/ml.
Each viral suspension was split into two aliquots. Each aliquot was 10-fold serially diluted in triplicate by two operators (six replicates). The RNA was extracted from each replicate and used as templates in two qRT-PCRs carried out by two operators (12 replicates).
Efficiency error and linearity
A standard curve was generated for each virus tested using the Cp values resulting from the qRT-PCR carried out on the 12 RNAs extracted from each virus dilution obtained as described above. A perfect amplification reaction would produce a standard curve with an efficiency of 2 because the amount of target nucleic acid would double with each amplification cycle (according to the formula: Tn = T0×2n). The error value is a measure of the accuracy of the quantification result based on the standard curve. An acceptable value would be <0.2. LightCycler® 480 software automatically calculates the efficiency and the error value. The linearity of the assay was also determined by regression curve analysis using the Cp values (y axis) and known concentrations of viral RNA (x axis).
Furthermore, the efficiency, error and linearity of the qRT-PCR were calculated, generating a standard curve, also used as a template in the duplicate 10-fold serial dilutions of RNA extracted from AMPV A (IT/Ty/A/259-01/03) and AMPV-B (IT/Ty/B/Vr240/87).
Repeatability of the assay
The RNA extracted from AMPV-A (IT/Ty/A/259-01/03) and AMPV-B (IT/Ty/B/Vr240/87) was serially 10-fold diluted by two operators. High and low dilutions (e.g. 10−1 and 10−4) were used as templates for the qRT-PCR in three different experiments performed weekly to assess the repeatability of the assay.
Statistical analysis was carried out with respect to the dilution using the t test for dependent samples, using Cp as the continuous dependent variable, and the operator, day of experiment and AMPV subtype as factors.
The coefficient of variation (CV) was also calculated to quantify the variability of the assay among different runs. P<0.05 was chosen as threshold of the level of significance. All statistical analyses were carried out using SPSS for Windows (Release 12.0.1; SPSS, Inc., Chicago, IL, USA).
Quantitative RT-PCR detection of AMPV from experimentally infected birds
Two experimental trials were carried out in isolation units. Ten 1-day-old specific pathogen-free chickens were inoculated with AMPV-A (Falchieri et al., Citation2013) at a dose of 104 TCID50 and were sampled at 4, 5, 6, 7 and 8 days post infection. Eight 21-day-old commercial turkeys were inoculated with AMPV-B IT/Ty/Vr240/87 strain at a dose of 103.77 TCID50 and were sampled at days 3, 5, 7 and 10 days post infection. In both trials, rhino-pharyngeal swabs were collected; the RNA was extracted as reported above and was used as a template for the qRT-PCR.
Comparative evaluation of the qRT-PCR and a conventional subtype A-specific and B-specific RT nested-PCR
The RNAs extracted from the AMPV-A IT/Ty/A/259-01/03 strain and the AMPV-B IT/Ty/B/Vr240/87 strain were diluted 10-fold and were analysed with both a qRT-PCR and an RT nested-PCR designed by Naylor et al. (Citation1997). The RNA extracted from the swabs collected from the experimentally infected birds was similarly tested using both methods.
Results
Assay optimization
Different reaction volumes, primer and probe concentrations and temperature–time profiles were tested. Adjusting the primer and probe concentrations and varying the thermal amplification profile did not significantly influence the efficiency and error; the best values (data not shown) were obtained using a 0.5 µmol primer and a 0.75 µmol probe in a final volume of 10 µl, and the following RT and amplification profile: 30 min at 45°C (reverse transcription); 2 min at 95°C (initial PCR activation step), 50 cycles at 95°C for 15 sec (denaturation), at 45°C for 30 sec (annealing) and at 72°C for 5 sec (extension); with fluorescence data collected at the end of each annealing step. The temperature profile was adapted from the manufacturer's instructions of the Superscript® III Platinum® One-Step Quantitative RT-PCR System (Invitrogen, Carlsbad, California, USA); a longer reverse transcription step (30 min instead of 15 min) and a lower temperature for this step (45°C instead 50°C) were especially crucial with regard to the sensitivity of the new qRT-PCR.
Specificity
All the AMPV subtype A and subtype B strains tested were amplified and specifically detected, generating Cp values ranging from 14.39 to 29.83 (). No heterologous signal was read by the specific FAM and HEX detectors. No amplification occurred when AMPV subtype C, other avian non-AMPV pathogens and negative controls were tested.
No substantial differences were observed for dilutions of RNA extracted from AMPV subtype A or subtype B, and from a mixture of both subtypes ().
Table 3. Comparison of Cp values obtained from dilutions of RNA of AMPV subtype A or subtype B, and from a mixture of RNA of subtype A + B dilutions.
Lower limit of detection of the assay
The detection limit of the assay was 10−0.41 TICD50/ml and 101.15 TCID50/ml when the AMPV-B IT/Ty/B/Vr240/87 strain and the AMPV-A IT/Ty/A/259-01/03 strain were used as templates, respectively. Cp values were also generated at higher dilutions of the viruses but in less than 50% of the replicates.
Efficiency, error and linearity
In all cases, the amplification efficiency was approximately 2 and the error values were <0.2 (). The dose/effect curves generated with either viral template or RNA exhibited significant linearity ( and ).
Figure 1. Standard dose/response curves of virus (1a) or RNA (1b) dilutions of representative virus strains belonging to AMPV subtype A (IT/Ty/A/259-01/03) and subtype B (IT/Ty/B/Vr240/87).
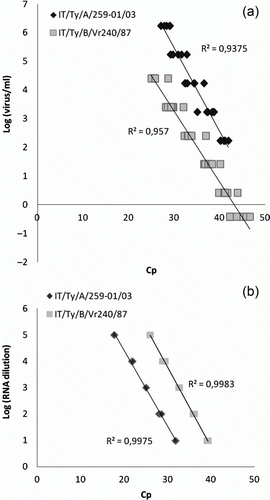
Table 4. Efficiency, error, linearity and detection limit of the qRT-PCR.
Repeatability of the assay
Even though a significant effect regarding the day of experiment was observed, the CV values of the assay demonstrated very good repeatability for both templates at both high and low RNA concentrations (AMPV-A CV range = 2.0 to 2.2%; AMPV-B CV range = 2.1 to 2.1%) ().
Table 5. Results of the repeatability of the assay.
The significance regarding the day of experiment factor (week 1 vs. week 2: dilution 10–1, P = 0.18 and dilution 10–4, P < 0.01; week 2 vs. week 3: dilution 10−1,<0.01 and dilution –4, P = 0.79; and week 1 vs. week 3: dilution −1, <0.01 and dilution −4, <0.01) was due to the relatively low variability regarding the days of the experiment and could be explained by the use of the same aliquot of RNA in all the three experiments and its possible degradation.
Detection of AMPV from experimentally infected birds, and comparative evaluation of qRT-PCR and conventional subtype A-specific and B-specific RT nested-PCR
The analysis of the RNA extracted from swabs confirmed the ability of the qRT-PCR assay herein developed to detect AMPV from infected birds (). Moreover, the qRT-PCR showed greater sensitivity than a conventional subtype A and subtype B RT nested-PCR (). The analysis of the RNA extracted from virus isolates confirmed the greater sensitivity of the qRT-PCR assay over that of conventional RT-PCR ().
Table 6. Comparison of a qRT-PCR and an RT nested-PCR in the detection of AMPV from infected birds.
Table 7. Comparison of a qRT-PCR and an RT nested-PCR in the detection of AMPV from RNA.
Discussion
The present study reports the development of a qRT-PCR protocol for the simultaneous identification, quantitation and differentiation of AMPV subtypes A and B. The protocol was focused on these two subtypes because of their almost worldwide distribution (Gough & Jones, Citation2008).
A real-time PCR technique is a valuable alternative to the more time-consuming conventional PCR and is, in general, more sensitive and specific, especially when suitable molecular probes are used.
The results of the qRT-PCR for the specific detection of AMPV-A and AMPV-B using the molecular beacon probes developed in this study showed that it was specific and did not cross-react with other AMPV subtypes and non-target bird respiratory pathogens.
Contrary to what observed in the AMPV qRT-PCR protocol of Guionie et al. (Citation2007), late responses (Cp values) with the heterologous probes or controls were never observed in our study. We think that this characteristic of our test would make AMPV subtype diagnosis easy and unequivocal, especially in mixed infections (both subtypes can co-circulate in the field, as reported by Banet-Noach et al. [Citation2005] and Chacón et al. [Citation2011]) or when the virus load in the field samples is low due to improper sample timing. Moreover, confidence regarding the positivity of the sample is always maintained, even when high Cp values are found. The higher specificity of our test was probably due to the different design of the assay and the chemistry used. Although one unique pair of primers was used to target and amplify both AMPV subtypes, the design of the probe played a successful role. Due to the high thermal stability of the hairpin structure, molecular beacon probes have the advantage of having greater specificity and a lower background as compared with other probes, such as TaqMan®, since they are, if well designed, even capable of differentiating single base-pair mismatches (Tyagi et al., Citation1998). As the efficacy of molecular beacons depends largely on genetic stability, molecular evolution of the AMPV strains circulating in the field should be continuously monitored in order to detect mutations eventually occurring in probe target genomic sequences, which could result in false negative results, reducing the specificity of the test and altering the epidemiological survey. Furthermore, more AMPV subtype A strains recently isolated should be tested.
The sensitivity of our qRT-PCR appeared to be similar to that observed with other qRT-PCR protocols for AMPV-B by Guionie et al. (Citation2007) or AMPV-A by Ferreira et al. (Citation2009) while, when it was compared with a conventional widely used subtype A-specific and subtype B-specific AMPV RT nested-PCR (Naylor et al., Citation1997), it showed a greater sensitivity.
This is in contrast with the results reported by Ferreira et al. (Citation2009) who found their AMPV qRT-PCRs that targeted F and N genes to be as sensitive as an F-based conventional RT-PCR. As an explanation for this observation, the authors suggested the presence of a pyrimidine residue at the 3′ end of the targeting primers used in the RT-PCR. It will be interesting in the future to introduce similar nucleotide patterns in designing our primers in order to further increase the qRT-PCR sensitivity.
In non-segmented negative-strand RNA viruses, the genes closest to the promoter are believed to be the most abundantly transcribed (Barik, Citation1992). Recent studies of AMPV gene expression have given different results. The expected picture of expression declining progressively from the leader to the end was not observed (Falchieri et al., Citation2012). This could explain why the most successful PCRs for AMPV have been designed in genes located downstream in the genome (Jing et al., Citation1993; Mase et al., Citation1996; Naylor et al., Citation1997; Ali & Reynolds, Citation1999; Bayon-auboyer et al., Citation1999; Pedersen et al., Citation2000, Citation2001; Shin et al., Citation2000; Cecchinato et al., Citation2004a, Citationb; Hess et al., Citation2004).
Our choice to target sequences in the SH gene was based on the results of a previous study (Cecchinato et al., Citation2004b) and on the ease of finding a genome region conserved within AMPV-A and AMPV-B for a primer design, and inside this region, subtype A-specific and subtype B-specific nucleotide sequences for probe design.
The standard curves generated either using the serial dilution of an RNA suspension or RNA extracted from a serial dilution of titrated viral suspensions as templates, exhibited both very good linearity (R 2>0.9375) between Cp values and virus quantities making the assay herein designed reliable for quantification. The linearity appeared slightly poorer when RNAs extracted from virus dilutions were tested. This indicated that the RNA extraction step is probably capable of influencing this parameter. Nevertheless, due to its comprehensiveness, the standard curves generated with RNAs extracted from virus dilutions are preferred for quantification purposes.
Our qRT-PCR may therefore be a valuable tool in experimental studies, especially when virus quantification is required (Catelli et al., Citation2012a, Citationb).
In summary, the qRT-PCR assay developed in this study provided a rapid, sensitive and specific test for AMPV subtype A and subtype B diagnosis, with characteristics of use in epidemiological and experimental studies.
Acknowledgements
The work described here was supported by University of Padua grant (ex 60%, 2011).
References
- Abbassi , H. , Rossow , K. , Zeigler , A.F. & Faaberg , K.S. 2007 . Molecular diagnosis of avian metapneumovirus (aMPV) . In Proceedings of the 56th Western Poultry Disease Conference (p. 28 – 30 ). Omnipress , Madison, WI , , USA .
- Ali , A. and Reynolds , D.L. 1999 . A reverse transcription-polymerase chain reaction assay for the detection of avian pneumovirus (Colorado strain) . Avian Diseases , 43 : 600 – 603 . doi: 10.2307/1592663
- Banet-Noach , C. , Simanov , L. and Perk , S. 2005 . Characterization of Israeli avian metapneumovirus strains in turkeys and chickens . Avian Pathology , 34 : 220 – 226 . doi: 10.1080/03079450500112625
- Barik , S. 1992 . Transcription of human respiratory syncytial virus genome RNA in vitro: requirement of cellular factor(s) . Journal of Virology , 66 : 6813 – 6818 .
- Bayon-Auboyer , M.H. , Arnauld , C. , Toquin , D. and Eterradossi , N. 2000 . Nucleotide sequences of the F, L and G protein genes of two non-A/non-B avian pneumoviruses (APV) reveal a novel APV subgroup . The Journal of General Virology , 81 : 2723 – 2733 .
- Bayon-Auboyer , M.H. , Jestin , V. , Toquin , D. , Cherbonnel , M. and Eterradossi , N. 1999 . Comparison of F-, G- and N-based RT-PCR protocols with conventional virological procedures for the detection and typing of turkey rhinotracheitis virus . Archives of Virology , 144 : 1091 – 1109 . doi: 10.1007/s007050050572
- Catelli , E. , Cecchinato , M. , Delogu , M. , De Matteo , P. , Ortali , G. , Franciosi , C. , De Marco , M.A. and Naylor , C.J. 2004 . Avian Pneumovirus infection in turkey and broiler farms in Italy: a virological, molecular and serological field survey . Italian Journal of Animal Science , 3 : 286 – 292 .
- Catelli , E. , Cecchinato , M. , Savage , C.E. , Jones , R.C. and Naylor , C.J. 2006 . Demonstration of loss of attenuation and extended field persistence of a live avian metapneumovirus vaccine . Vaccine , 24 : 6476 – 6482 . doi: 10.1016/j.vaccine.2006.06.076
- Catelli , E. , Cook , J.K.A. , Chesher , J. , Orbell , S.J. , Woods , M.A. , Baxendale , W. and Huggins , M.B. 1998 . The use of virus isolation, histopathology and immunoperoxidase techniques to study the dissemination of a chicken isolate of avian pneumovirus in chickens . Avian Pathology , 27 : 632 – 640 . doi: 10.1080/03079459808419395
- Catelli , E. , Lupini , C. , Cecchinato , M. , Ricchizzi , E. , Brown , P. and Naylor , C.J. 2010 . Field avian metapneumovirus evolution avoiding vaccine induced immunity . Vaccine , 28 : 916 – 921 . doi: 10.1016/j.vaccine.2009.10.149
- Catelli , E. , Lupini , C. , Listorti , V. , Marziali , A. , De Matteo , P. , Naylor , C.J. & Cecchinato , M. ( 2012a ). The pigeon (Columba livia) is not sensitive to Avian Metapneumovirus subtype B and does not play any role in virus spread in experimental conditions . In M. Lierz , U. Heffels-Redmann , E.F. Kaleta , & J. Heckmann . Proceedings of the VII International Symposium on Avian Corona and Pneumovirus Infections (p. 285 – 291 ). Rauischholzhausen , Germany .
- Catelli , E. , Lupini , C. , Listorti , V. , Muñoz Pogoreltseva , O.S. , Terregino , C. , Cecchettin , K. & Cecchinato , M. ( 2012b ). Vaccine interaction and protection against virulent Avian Metapneumovirus (AMPV) challenge after combined administration of Newcastle disease and AMPV live vaccine to one-day old turkeys . In Proceedings of the 61st Western Poultry Disease Conference (p. 38 – 40 ). Scottsdale , Arizona , USA .
- Catelli , E. , Terregino , C. , De Marco , M.A. , Delogu , M. and Guberti , V. 2001 . Serological evidence of avian pneumovirus infection in reared and free-living pheasants . The Veterinary Record , 149 : 56 – 58 . doi: 10.1136/vr.149.2.56
- Cecchinato , M. , Catelli , E. , Lupini , C. , Ricchizzi , E. , Clubbe , J. , Battilani , M. and Naylor , C.J. 2010 . Avian metapneumovirus (AMPV) attachment protein involvement in probable virus evolution concurrent with mass live vaccine introduction . Veterinary Microbiology , 146 : 24 – 34 . doi: 10.1016/j.vetmic.2010.04.014
- Cecchinato , M. , Catelli , E. , Savage , C.E. , Jones , R.C. and Naylor , C.J. 2004a . Design, validation, and absolute sensitivity of a novel test for the molecular detection of avian pneumovirus . Journal of Veterinary Diagnostic Investigation , 16 : 582 – 585 . doi: 10.1177/104063870401600616
- Cecchinato , M. , Catelli , E. , Savage , C.E. , Pesente , P. , Jones , R.C. & Naylor C.J. ( 2004b ). Design and sensitivity of PCR tests for avian pneumovirus . In U. Heffels-Redmann , & E.F. Kaleta . Proceedings of the IV International Symposium on Avian Corona and Pneumovirus Infections (p. 294 – 301 ). Rauischholzhausen , Germany .
- Cecchinato , M. , Lupini , C. , Ricchizzi , E. , Falchieri , M. , Meini , A. , Jones , R.C. and Catelli , E. 2012 . Italian field survey reveals a high diffusion of avian metapneumovirus subtype B in layers and weaknesses in the vaccination strategy applied . Avian Diseases , 56 : 720 – 724 . doi: 10.1637/10202-041312-Reg.1
- Chacón , J.L. , Mizuma , M. , Vejarano , M.P. , Toquín , D. , Eterradossi , N. , Patnayak , D.P. , Goyal , S.M. and Ferreira , A.J. 2011 . Avian metapneumovirus subtypes circulating in Brazilian vaccinated and non vaccinated chicken and turkey farms . Avian Diseases , 55 : 82 – 89 . doi: 10.1637/9501-081310-Reg.1
- Cook , J.K.A. and Cavanagh , D. 2002 . Detection and differentiation of avian pneumoviruses (metapneumoviruses) . Avian Patholology , 31 : 117 – 132 . doi: 10.1080/03079450120118603
- Easton , A.J. , Domachowske , J.B. and Rosenberg , H.F. 2004 . Animal pneumoviruses: molecular genetics and pathogenesis . Clinical Microbiology Reviews , 17 : 390 – 412 . doi: 10.1128/CMR.17.2.390-412.2004
- Falchieri , M. , Cecchinato , M. & Naylor , C.J. 2012 . Unexpected pattern in expression of AMPV proteins: possible explanations and implications . In M. Lierz , U. Heffels-Redmann , E.F. Kaleta , & J. Heckmann . Proceedings of the VII International Symposium on Avian Corona and Pneumovirus Infections (p. 325 ). Rauischholzhausen , Germany .
- Falchieri , M. , Lupini , C. , Cecchinato , M. , Catelli , E. , Kontolaimou , M. & Naylor , C.J. 2013 . Avian metapneumoviruses expressing Infectious Bronchitis virus genes are stable and induce protection . Vaccine , in press , doi: doi: 10.1016/j.vaccine.2013.03.055
- Ferreira , H.L , Spilki , F.R. , Santos , M.M.A. , de Almeida , R.S. and Arns , C.W. 2009 . Comparative evaluation of conventional RT-PCR and real-time RT-PCR (RRT-PCR) for detection of avian metapneumovirus subtype A . Ciência Rural, Santa Maria , 39 : 1445 – 1451 .
- Gough , R.E. and Jones , R.C. 2008 . “ Avian Metapneumoviruses ” . In Diseases of Poultry , 12th edn , Edited by: Saif , Y.M. , Fadly , A.M. , Glisson , J.R. , McDougald , L.R. , Nolan , L.K and Swayne , D.E. 100 – 110 . Ames : Blackwell Publishing .
- Guionie , O. , Toquin , D. , Sellal , E. , Bouley , S. , Zwingelstein , F. , Allée , C. , Bougeard , S. , Lemière , S. and Eterradossi , N. 2007 . Laboratory evaluation of a quantitative real-time reverse transcription PCR assay for the detection and identification of the four subgroups of avian metapneumovirus . Journal of Virological Methods , 139 : 150 – 158 . doi: 10.1016/j.jviromet.2006.09.022
- Hess , M. , Huggins , M.B. , Mudzamiri , R. and Heincz , U. 2004 . Avian metapneumovirus excretion in vaccinated and non-vaccinated specified pathogen free laying chickens . Avian Pathology , 33 : 35 – 40 . doi: 10.1080/0307945031000163273
- Jing , L. , Cook , J.K.A. , David , T. , Brown , K. , Shaw , K. and Cavanagh , D. 1993 . Detection of turkey rhinotracheitis virus in turkeys using the polymerase chain reaction . Avian Pathology , 22 : 771 – 783 . doi: 10.1080/03079459308418963
- Kles , V. , Morin , M. , Picault , J. , Drouin , P. , Guittet , M. and Bennejean , G. 1987 . Isolement d'un virus dans un cas de syndrome infectioux du gonflement de la tete avec coryza chez la pintade . Isolation of a virus during an outbreak of coryza and Swollen Head Syndrome in guinea fowl. L'Aviculteur , 483 : 112
- Kwon , J.S. , Lee , H.J. , Jeong , S.H. , Park , J.Y. , Hong , Y.H. , Lee , Y.J. , Youn , H.S. , Lee , D.W. , Do , S.H. , Park , S.Y. , Choi , I.S. , Lee , J.B. and Song , C.S. 2010 . Isolation and characterization of avian metapneumovirus from chickens in Korea . Journal of Veterinary Science , 11 : 59 – 66 . doi: 10.4142/jvs.2010.11.1.59
- Lee , E. , Song , M.S. , Shin , J.Y. , Lee , Y.M. , Kim , C.J. , Lee , Y.S. , Kim , H. and Choi , Y.K. 2007 . Genetic characterization of avian metapneumovirus subtype C isolated from pheasants in a live bird market . Virus Research , 128 : 18 – 25 . doi: 10.1016/j.virusres.2007.03.029
- Lupini , C. , Cecchinato , M. , Ricchizzi , E. , Naylor , C.J. and Catelli , E. 2011 . A turkey rhinotracheitis outbreak caused by the environmental spread of a vaccine-derived avian metapneumovirus . Avian Pathology , 40 : 525 – 530 . doi: 10.1080/03079457.2011.607428
- Mase , M. , Asahi , S. , Imai , K. , Nakamura , K. and Yamaguchi , S. 1996 . Detection of turkey rhinotracheitis virus from chickens with swollen head syndrome by reverse transcriptase-polymerase chain reaction (RT-PCR) . The Journal of Veterinary Medical Science , 58 : 359 – 361 . doi: 10.1292/jvms.58.359
- Naylor , C.J. and Jones , R.C. 1993 . Turkey rinotracheitis virus: a review . Veterinary Bulletin , 63 : 439 – 449 .
- Naylor , C. , Shaw , K. , Britton , P. and Cavanagh , D. 1997 . Appearance of type B avian pneumovirus in Great Britain . Avian Pathology , 26 : 327 – 338 . doi: 10.1080/03079459708419215
- OIE – World Organization of Animal Health . 2012 . Principles and methods of validation of diagnostic assay for infectious diseases . In Terrestrial Animal Health Code , Chapter 1.1.5, available at: http://www.oie.int/fileadmin/Home/eng/Health_standards/tahm/1.01.05_VALIDATION.pdf .
- Pedersen , J.C. , Reynolds , D.L. and Ali , A. 2000 . The sensitivity and specificity of a reverse transcription-polymerase chain reaction assay for the avian pneumovirus (Colorado strain) . Avian Diseases , 44 : 681 – 685 . doi: 10.2307/1593111
- Pedersen , J.C. , Senne , D.A. , Panigrahy , B. and Reynolds , D.L. 2001 . Detection of avian pneumovirus in tissues and swab specimens from infected turkeys . Avian Diseases , 45 : 581 – 592 . doi: 10.2307/1592898
- Reed , L.J. and Muench , H. 1938 . A simple method of estimating fifty percent end points . American Journal of Hygiene , 27 : 493 – 497 .
- Senne , D.A. , Edson . R.K. , Pederson , J.C. & Panigrahy , B. 1997 . Avian pneumovirus update . In AVMA (Ed.). Proceedings of the 134th Annual Congress of American Veterinary Medical Association (p. 190 ). Nevada , USA .
- Shin , H.J. , Rajashekara , G. , Jirjis , F.F. , Shaw , D.P. , Goyal , S.M. , Halvorson , D.A. and Nagaraja , K.V. 2000 . Specific detection of avian pneumovirus (APV) US isolates by RT-PCR . Archives of Virology , 145 : 1239 – 1246 . doi: 10.1007/s007050070123
- Tan , W. , Fang , X. , Li , J. and Liu , X. 2000 . Molecular beacons: a novel DNA probe for nucleic acid and protein studies . Chemistry , 6 : 1107 – 1111 . doi: 10.1002/(SICI)1521-3765(20000403)6:7%3C1107::AID-CHEM1107%3E3.3.CO;2-0
- Toquin , D. , Bayon-Auboyer , M.H. , Eterradossi , N. and Jestin , V. 1999 . Isolation of a pneumovirus from a Muscovy duck . The Veterinary Record , 145 : 680
- Toquin , D. , Guionie , O. , Jestin , V. , Zwingelstein , F. , Allee , C. and Eterradossi , N. 2006 . European and American subgroup C isolates of avian metapneumovirus belong to different genetic lineages . Virus Genes , 32 : 97 – 103 . doi: 10.1007/s11262-005-5850-3
- Tyagi , S. , Bratu , D.P. and Kramer , F.R. 1998 . Multicolor molecular beacons for allele discrimination . Nature Biotechnology , 16 : 49 – 53 . doi: 10.1038/nbt0198-49
- Velayudhan , B.T. , McComb , B. , Bennett , R.S. , Lopes , V.C. , Shaw , D. , Halvorson , D.A. and Nagaraja , K.V. 2005 . Emergence of a virulent type C avian metapneumovirus in turkeys in Minnesota . Avian Diseases , 49 : 520 – 526 . doi: 10.1637/7388-052805R.1