Abstract
The present study evaluated the adjuvant effect of live attenuated salmonella organisms expressing the heat-labile toxin of Escherichia coli B subunit (LTB) on the efficacy of an avian pathogenic Escherichia coli (APEC) vaccine. The Asd+ (aspartate semialdehyde dehydrogenase) plasmid pMMP906 containing the LTB gene was introduced into a Salmonella enterica Typhimurium strain lacking the lon, cpxR and asd genes to generate the adjuvant strain. Live recombinant Salmonella-delivered APEC vaccine candidates were used for this study. The birds were divided into three groups: group A, non-vaccinated controls; group B, immunized with vaccine candidates only; and group C, immunized with vaccine candidates and the LTB strain. The immune responses were measured and the birds were challenged at 21 days of age with a virulent APEC strain. Group C showed a significant increase in plasma IgG and intestinal IgA levels and a significantly higher lymphocyte proliferation response compared with the other groups. Upon challenge with the virulent APEC strain, group C showed effective protection whereas group B did not. We also attempted to optimize the effective dose of the adjuvant. The birds were immunized with the vaccine candidates together with 1×107 or 1×108 colony-forming units of the LTB strain and were subsequently challenged at 3 weeks of age. The 1×107 colony-forming units of the LTB strain showed a greater adjuvant effect with increased levels of serum IgG, intestinal IgA and a potent lymphocyte proliferation response, and yielded higher protection against challenge. Overall, the LTB strain increased the efficacy of the Salmonella -delivered APEC vaccine, indicating that vaccination for APEC along with the LTB strain appears to increase the efficacy for protection against colibacillosis in broiler chickens.
Introduction
Avian pathogenic Escherichia coli (APEC) cause a number of extra-intestinal diseases in poultry, collectively known as colibacillosis, which typically are characterized by airsacculitis, omphalitis, pericarditis, perihepatitis and peritonitis (Pourbakhsh et al., Citation1997a; Barnes et al., Citation2008). A number of virulence factors, including P-fimbriae (pap gene cluster), CS31A adhesin (clpG), and iron regulated aerobactin receptor (iutA), have been associated with APEC (Pourbakhsh et al., Citation1997b; Stordeur et al., Citation2002; Dziva & Stevens, Citation2008). The prevention of APEC infection by vaccination is a valuable control measure. Several attempts to develop a vaccine for the prevention of APEC infection have been demonstrated experimentally (Arp, Citation1980; Melamed et al., Citation1991; Frommer et al., Citation1994; Kwaga et al., Citation1994; Peighambari et al., Citation2002). More recently, we have successfully shown that the live attenuated Salmonella-based APEC vaccine has the ability to offer protection against APEC bearing multiple virulence factors (Chaudhari et al., Citation2013). Since APEC is capable of surviving inside macrophages (Bastiani et al., Citation2005) and is resistant to phagocytosis (Pourbakhsh et al., 1997b), a vaccine that elicits mucosal as well as cellular immune responses would be more effective against colibacillosis. Reinforcing this notion, we have recently demonstrated that a live form of Salmonella-delivered APEC vaccine not only induces cellular immunity, but also produces gut-associated mucosal immunity after oral administration (Chaudhari et al., Citation2013).
A suitable route of immunization is important for the delivery of such vaccines. The oral route of vaccination is convenient and safe (Ogra et al., Citation2001; Chaudhari et al., Citation2013), but large doses of antigens are needed to obtain a desired immune response. Therefore, immune responses following oral immunization are dependent upon the co-administration of appropriate adjuvant that can initiate and promote a greater immune response (Freytag & Clements, Citation2005). Adjuvant substances, when administered with antigen, not only increase the potency of the humoral and cellular immune responses against the antigen, but also prolong the immune responses (da Silva Ramos Rocha et al., Citation2008). In animal models, the most commonly used mucosal adjuvants are bacterial toxins, such as cholera toxin (CT), E. coli heat-labile enterotoxin (LT) and their derivatives (Freytag & Clement, Citation1999; Rask et al., Citation2000; McCluskie et al., Citation2001; Millar et al., Citation2001; Yamamoto et al., Citation2001).
LT is a potential adjuvant molecule and is composed of one enzymatically active A subunit (LTA) and five molecules of non-toxic B subunits (LTB). The LTB molecules have been used as an adjuvant for foreign antigens (Ogra et al., Citation2001). LTB is a potent mucosal and parenteral adjuvant and induces a strong immune response against co-administered antigens (de Haan et al., Citation1998; Conceição et al., Citation2006; Fingerut et al., Citation2006) and also activates the lymphocyte population (Williams, Citation2000). LTB protein has been successfully expressed in a variety of live attenuated bacterial hosts and was able to enhance immunogenicity in animal models (Clements et al., Citation1986; Maskell et al., Citation1987; Hayward et al., Citation1999; Khan et al., Citation2007). Hur & Lee (Citation2011) previously reported that a live attenuated Salmonella strain expressing LTB, when co-administered with a novel live Salmonella vaccine in which virulence genes had been deleted, elicited efficient immune responses.
In the present study, we evaluated the adjuvant effect of Salmonella-based heat labile B subunits toxin of Escherichia coli on the efficacy of live Salmonella -delivered APEC vaccines. For this, a live Salmonella enterica Typhimurium strain harbouring a plasmid vector containing the gene eltB encoding for LTB (Hur & Lee, Citation2011) was used as an adjuvant for the live recombinant vaccines that express genes for various virulence factors such as PapA, PapG, Iut-A and CS31A (Chaudhari et al., Citation2013).
Materials and Methods
Bacterial strains, construction of LTB and vaccine strains and growth conditions
The bacterial strains used in this study are listed in . A wild-type S. Typhimurium isolate from a chicken was used as the donor strain for construction of LTB and vaccine strains and was designated as JOL401 (Hur & Lee, Citation2011). JOL 718, a virulent wild-type APEC isolate, was isolated from liver of a chicken that died of colisepticaemia and was used as the challenge strain (Chaudhari et al., Citation2013). Bacterial strains were grown at 37°C in Luria–Bertani media (Difco™, Sparks, MD, USA) or tryptic soy broth (Bacto) with or without antibiotics. Diaminopimelic acid (Sigma, St Louis, Missouri, USA) was added for the growth of strains with Asd requirement. Phosphate-buffered saline (PBS, pH 7.0) was used for suspension of the vaccine and challenge strains. The LTB strain was constructed as described earlier (Hur & Lee, Citation2011). Briefly, the eltb gene was inserted into the plasmid with Asd requirement, pMMP65, and the resultant plasmid was indicated as pMMP65-LTB. The pMMP65-LTB plasmid containing the LTB gene was introduced into the JOL912 strain to generate the adjuvant strain, JOL 906. All four APEC vaccine candidates were constructed as described earlier (Chaudhari et al., Citation2013). Briefly, papA, papG, iutA, and clpG genes were cloned into the pBP244 vector () and the resultant plasmids were transformed into an attenuated the S. Typhimurium strain JOL912.
Table 1. Bacterial strains and plasmids used in this study.
Immunization and challenge experiments
The bird experiments in this study were conducted with the approval of the Chonbuk National University Animal Ethics Committee (CBU 2011–0017) and were in accordance with the guidelines of the Korean Council on Animal Care. One-day-old Arbor Acres Plus-S broiler chicks were used. The birds were provided water and antibiotic-free feed ad libitum. To investigate the adjuvant effect of LTB, the broiler chicks were divided into three groups (n = 25/group), two vaccinated groups and one non-vaccinated control group. The groups were designated as: group A, non-vaccinated controls who received PBS only via the oral route; group B, immunized orally with vaccine candidates only; and group C, immunized orally with vaccine candidates and the LTB strain. The birds were immunized at day 1 of age. The dose used for vaccination was 1×107 colony-forming units (CFU) for each Salmonella strain delivering APEC antigens (JOL 924, JOL 928, JOL 930 and JOL 954) and the LTB strain (JOL 906) in 100 µl PBS suspension. Five birds from each group were sacrificed at 3 weeks of age for the measurement of antibody response and lymphocyte proliferative responses as described below in the text. At 21 days of age, the birds (n = 20) were injected via the air sac route with 100 µl suspension containing 1×106 CFU of the APEC virulent strain JOL 718 (Chaudhari et al., Citation2013). The birds were subsequently monitored for mortality and were sacrificed 7 days post challenge.
We further sought to optimize the effective dose of the adjuvant strain. Seventy-five broiler chicks were randomly divided into three groups (n = 25/group). The birds were inoculated via the oral route at 1 day of age with 100 µl of bacterial suspension in sterile PBS containing 1×107 CFU of the overnight culture of each Salmonella -delivered APEC vaccine candidate. Groups were designated as: group I, non-vaccinated controls (PBS instead of LTB); group II, immunized with 1×107 CFU LTB along with all four Salmonella -delivered APEC vaccine candidates; and group III, immunized with 1×108 CFU LTB along with the Salmonella-delivered APEC vaccine candidates.
Observations of mortality, body weight gain, gross lesions and bacteraemia post challenge
After challenge with the virulent APEC strain, the mortality rate was assessed daily for 7 days. After the apparent recovery of all remaining birds, all chicks were sacrificed on the seventh day post challenge. The body weight of all groups of birds was measured before challenge and considered as the body weight (in grams) of each individual bird at day 0. The body weight of surviving birds (n = 8/group) was measured on day 7 post challenge. Finally, the body weight gain was measured as the difference between body weights on day 0 and on the seventh day post challenge for each bird in each group. Gross lesions were assigned to the air sac, heart and liver (Gyimah et al., Citation1986; Chaudhari et al., Citation2013). A score of 0 indicated no lesion and higher scores such as 1, 2 and 3 meant more severe lesions. The lesion score for the air sac was considered moderate or severe depending on the cloudiness and thickness with the presence of serous or fibrinopurulent exudate. The presence of cloudy and fibrinopurulent exudate was also examined in the pericardial cavity. The presence of a whitish perihepatic membrane on the liver was considered the characteristic APEC lesion for the liver. The mean lesion score per group was determined and the differences among the groups were compared statistically. To study bacteraemia, blood samples were collected at 6, 24 and 48 h post inoculation from groups A, B and C birds (n = 5). The same birds were used for the blood collection for bacterial recovery. Five hundred microlitres of blood were collected and the presence of bacteria in the blood sample was determined by spreading the samples on eosin–methylene blue agar (Difco) and incubating at 37°C for 24 h. Polymerase chain reaction confirmation using specific primers for the tsh and iss genes was performed on one to several typical colonies for the APEC strain after bacterial recovery on eosin–methylene blue agar (data not shown).
Collection of plasma and samples for intestinal sIgA analysis
Samples were collected from five birds per group in order to examine the immune responses. Plasma samples collected at 3 weeks of age were separated by centrifugation of the peripheral blood collected from the jugular vein of each bird. The birds were sacrificed and intestinal washings were collected by washing a 10 cm segment of small intestine at the Meckel's diverticulum with 1 ml of PBS, which was then centrifuged at 16,000×g for 3 min at 4°C. One millilitre of the supernatant was added to 10 µl of 100 mM phenylmethanesulfonyl fluoride (Sigma-Aldrich, St Louis, Missouri, USA) solution, 10 µl of 1% (w/v) sodium azide, and 5% (w/v) of bovine serum albumin. The samples were stored at −20°C until use.
Enzyme-linked immunosorbent assay
The presence of plasma IgG and intestinal IgA specific to antigens was determined using chicken IgG and IgA ELISA Quantitation kits (Bethyl Laboratories, Montgomery, Texas, USA) according to the manufacturer's instructions. Wells of Microlon® ELISA plates (Greiner Bio-One GmbH, Frickenhausen, Germany) were coated with 100 µl of purified E. coli antigens (PapA, PapG, Iut-A, CS31A) at a concentration of 5 µg/ml (Chaudhari et al., Citation2013). The values for plasma IgG and intestinal IgA were determined as the mean plus standard deviation (SD) for five samples assayed at a dilution of 1:80 and 1:8 in PBS, respectively. A standard curve describing the relation between the concentration of standards and their absorbance value was generated, and the concentration of antibody for each sample was indicated in nanograms per millilitre. The absorbance of the developed colour was measured at 492 nm. All assays were performed in duplicate.
Lymphocyte proliferation assay
Twenty-one days after immunization, peripheral lymphocytes were separated from five randomly selected chickens per group using the gentle swirl technique (Gogal et al., Citation1997). The lymphocytes were washed twice with complete RPMI-1640 media (Sigma-Aldrich) supplemented with 10% foetal calf serum, 2 mM l-glutamine, 50 units/ml of penicillin, 50 µg/ml of streptomycin, and 2 µg/ml of fungizone. One hundred microlitres of the cell suspension (1×105 cells) was incubated in triplicate in 96-well tissue culture plates with 50 µl of medium alone or with medium containing 4.0 µg of purified PapA and IutA (Chaudhari et al., Citation2013) at 40°C in a humidified 5% CO2 atmosphere for 72 h. The proliferation of stimulated lymphocytes was measured using adenosine triphosphate bioluminescence as a marker of cell viability with the ViaLight® Plus Kit (Lonza, Rockland, Maine, USA), which provides an estimation of mitochondrial activity (Crouch et al., Citation1993), according to the product information. The emitted light intensity was measured using a luminometer (TriStarLB941; Berthold Technologies GmbH & Co.; Bad Wildbad, Germany) with an integrated programme for 1 sec. The blastogenic response against a specific antigen was expressed as the mean stimulation index as previously reported (Chaudhari et al., Citation2013).
Statistical analysis
All data are expressed as mean±SD unless otherwise specified. Analyses were performed with SPSS 16.0 (SPSS Inc., Chicago, Illinois, USA). A non-parametric chi-square test was used to analyse significant differences in mortality, morbidity and lesion scores. One-way analysis of variance with post-hoc Bonferroni adjustments was used to analyse statistical differences in immune responses between the immunized groups and the unimmunized control group. Differences between the immunized groups and the unimmunized control group were considered statistically significant when P ≤ 0.05 or P ≤ 0.01.
Results
Evaluation of the adjuvant effect of the LTB strain on Salmonella-delivered APEC vaccine efficacy
We examined the adjuvant effect of the LTB strain on the efficacy of APEC vaccines. The birds were immunized at 1 day of age with the live Salmonella-delivered APEC vaccines with and without the LTB strain and all birds were subsequently challenged at 3 weeks of age. The vaccination patterns, mortality rates, number of affected birds showing gross lesions, mean lesion scores, body weight gain and bacteraemia post challenge are presented in . Group C, which was immunized along with the LTB strain, showed 10% acute mortality, whereas group A, the non-vaccinated controls, showed 60% mortality. Group B, which was vaccinated orally with only the vaccines, showed 25% mortality.
Table 2. Evaluation of adjuvant effect of the LTB strain on efficacy of Salmonella-delivered APEC vaccine in chickens.
The surviving birds were sacrificed 7 days post challenge and scores were assigned for gross lesions. Immunization with Salmonella -delivered APEC vaccines together with the LTB strain showed significantly higher protection than immunization with only the vaccine candidates (). Each acute mortality and gross lesion score was considered to determine the number of affected birds in each group, expressed as the morbidity rate. Group C had significantly fewer infected birds (30%) than group B (45%) and group A (100%). Moreover, group C showed significant body weight gain compared with the other groups.
To elucidate the systemic protective effect of vaccination, bacteraemia was monitored 6, 24 and 48 h post challenge. Bacteria were isolated from the blood 6 h post challenge in all groups. The bacteraemia decreased over time in groups B and C (). These differences were significant 24 and 48 h post challenge. High-level bacteraemia was still observed 48 h post challenge in group A. In contrast, none of the birds in group C showed bacteraemia 48 h after challenge.
Optimization of LTB dose
The LTB strain was further evaluated to determine the optimized dose. Birds were vaccinated at 1 day of age with the Salmonella -delivered APEC vaccines along with 1×107 or 1×108 CFU of the LTB strain and subsequently challenged at 21 days of age with the virulent APEC strain. Groups were designated as described in the Materials and Methods section. The mortality rate, morbidity and body weight gain are presented in . Upon challenge, group I and group III showed 40% mortality whereas group II showed 20% acute mortality. Surviving birds were sacrificed after 7 days of challenge and examined to determine the gross lesion score, indicating conditions such as pericarditis, perihepatitis and airsacculitis. Four out of 16 surviving birds in group II had a mild lesion score. Interestingly, group III, which received oral immunization with a higher LTB dose, showed similar lesion scores to the non-vaccinated control group (group I). The body weight gain was measured to monitor the general condition of the birds post challenge. Group II showed significantly higher body weight gain compared with the other groups ().
Table 3. Mortality and lesion scores in chickens immunized with Salmonella-delivered APEC vaccine along with LTB adjuvant at different doses.
Immunization with Salmonella -delivered APEC vaccine along with the LTB strain significantly increased the plasma IgG and intestinal IgA levels. The levels of plasma IgG against specific antigens were examined when the birds were 3 weeks of age. The data specific for antigens including P-fimbrial antigens (PapA and PapG), aerobactin receptor (IutA) and adhesion (CS31A) are shown in . a indicates the results for plasma IgG levels in birds that received APEC vaccination with and without the LTB strain. Group C showed significantly increased plasma IgG levels compared with group B and group A (a). b represents the plasma IgG levels for groups I, II and III. Group II, which was immunized with the Salmonella -delivered APEC vaccine and 1×107 CFU of LTB strain, showed a significantly greater rise in plasma IgG levels than the non-vaccinated controls and group III, which was immunized with the Salmonella -based APEC vaccine and 1×108 CFU of LTB (b).
Figure 1. Antigen-specific plasma levels of IgG by indirect enzyme-linked immunosorbent assay in vaccinated and non-vaccinated groups. 1a: plasma IgG concentration measured after APEC immunization with or without LTB adjuvant. 1b: plasma IgG levels (ng/ml) at 3 weeks post immunization with APEC vaccine along with LTB at two different doses, expressed as mean±SD. Antibody levels were considered significant if P≤0.05 or P≤0.01. *P<0.05, **P<0.01. Group A, non-vaccinated controls; group B, immunized orally with vaccine candidates only; and group C, immunized orally with vaccine candidates and the LTB strain; group I, non-vaccinated controls; group II, immunized with 1×107 CFU LTB along with all four Salmonella-delivered APEC vaccine candidates; and group III, immunized with 1×108 CFU LTB along with the Salmonella-delivered APEC vaccine candidates.
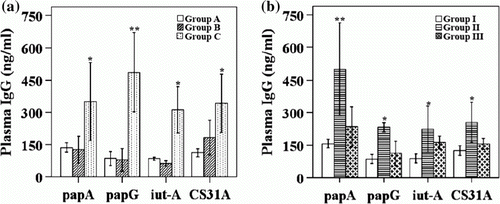
The levels of antigen-specific intestinal sIgA were also examined at the third week after immunization. The results presented in a are the intestinal sIgA levels after immunization with and without the LTB strain. Although an increase in intestinal sIgA levels in group B was observed, group C, which was immunized with the LTB strain, showed a significant rise in intestinal IgA levels at the third week after immunization (a). Group II, which was immunized with the APEC vaccine and 1×107 CFU LTB, showed significantly increased levels of IgA compared with groups I and III (b). The use of 8×107 CFU of LTB did not yield an increase in intestinal IgA levels.
Figure 2. Intestinal IgA antibody response to specific antigens. 2a: sIgA antibody levels expressed as mean±SD at 3 weeks of age after APEC immunization with or without LTB adjuvant. 2b: intestinal washings were examined for levels of sIgA against specific antigens after immunization with APEC vaccine along with LTB adjuvant at two different doses. Antibody levels were considered significant if P≤0.05 or P≤0.01. *P<0.05, **P<0.01. Group A, non-vaccinated controls; group B, immunized orally with vaccine candidates only; and group C, immunized orally with vaccine candidates and the LTB strain; group I, non-vaccinated controls; group II, immunized with 1×107 CFU LTB along with all four Salmonella-delivered APEC vaccine candidates; and group III, immunized with 1×108 CFU LTB along with the Salmonella-delivered APEC vaccine candidates.
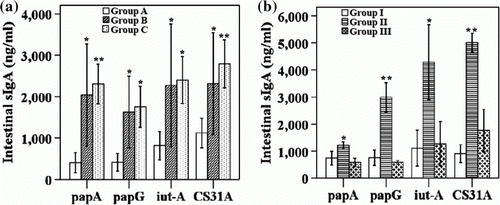
Antigen-specific lymphocyte proliferation assay
Cellular immune responses to specific antigens after vaccination with and without the LTB strain were further investigated. a shows the results of the lymphocyte proliferation assay when the birds were immunized with and without the LTB strain. Group C showed a sharp increase in lymphocyte proliferation in response to the vaccine antigens with stimulation indices of 3.4 and 3.1 for PapA and iut-A, respectively, compared with group B. b represents the lymphocyte proliferation responses for groups I, II and III. As shown in b, group II, which received the Salmonella -delivered APEC vaccine along with 1×107 CFU of the LTB strain, showed a significantly higher stimulation index than groups I and III at 3 weeks of age.
Figure 3. Antigen-specific lymphocyte stimulation responses in immunized chicks at 3 weeks of age. 3a: lymphocyte stimulation responses in chicks immunized with APEC vaccine with or without LTB adjuvant. 3b: stimulation index of lymphocyte sample from the chickens by peripheral lymphocyte proliferation assay using PapA and iutA antigens at 21 days post immunization with APEC vaccine along with LTB adjuvant at two different doses. *P<0.05, **P<0.01 vs. non-vaccinated control. Group A, non-vaccinated controls; group B, immunized orally with vaccine candidates only; and group C, immunized orally with vaccine candidates and the LTB strain; group I, non-vaccinated controls; group II, immunized with 1×107 CFU LTB along with all four Salmonella-delivered APEC vaccine candidates; and group III, immunized with 1×108 CFU LTB along with the Salmonella-delivered APEC vaccine candidates.
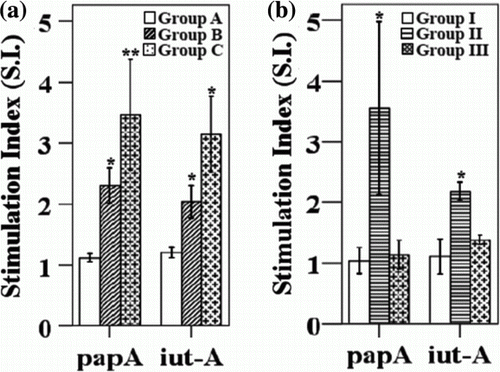
Discussion
The mucosal surfaces of the gastrointestinal tracts are the primary sites of infection for enteric pathogens; mucosal immunization (especially by the oral route) that can elicit protective immunity therefore has immense importance (Holmgren et al., Citation2003). The immune responses induced by oral immunization are frequently dependent upon the co-administration of appropriate mucosal adjuvants (Freytag & Clements, Citation2005). Adjuvants are substances that when co-administered with antigen can modify or increase the potency of the antigen-specific humoral and cellular immune responses (da Silva Ramos Rocha et al., Citation2008). The present study evaluated the adjuvant effect of live attenuated Salmonella organisms expressing LTB for Salmonella -delivered APEC vaccine candidates. Oral vaccine inoculation along with cMIA I, consisting of diadzein, lactic acid bacteria and sodium fluoride, and cMIA II, consisting of CpG and sodium fluoride, increased the mucosal and systemic immune responses and the protective effect against the Newcastle disease virus infection in chickens (Zhao et al., Citation2006). A few adjuvants have been shown to induce both systemic and mucosal immune responses in the context of a viral infection (Zhao et al., Citation2006; Zhang et al., Citation2007, Citation2008). However, few studies of adjuvants have been attempted for bacterial infections. LTB is one of the most commonly used mucosal adjuvants in animal models (Freytag & Clements, Citation2005). LTB has been reported as a safe and effective adjuvant that functions efficiently after mucosal immunization, especially by the oral route (Freytag & Clements, Citation2005; Hur & Lee, Citation2011).
In the present study we demonstrated that use of the LTB strain during APEC vaccination is more effective than vaccination without LTB. Upon virulent APEC challenge, immunization along with LTB adjuvant strain offered efficient protection. Oral immunization without LTB adjuvant strain offered 75% protection against virulent challenge whereas immunization along with adjuvant strain efficiently increased the protection rate to 90% (). The oral administration of LTB adjuvant along with influenza vaccine has been shown to increase the efficacy of the vaccine against virulent infection (Haan et al., Citation2001). In a mouse model, oral immunization with LTB as an adjuvant also efficiently protected against Helicobacter pylori challenge (Weltzin et al., Citation2000). Very few studies have explored the adjuvant effect of LTB in chickens (Khoury & Meinersmann, Citation1995; Fingerut et al., Citation2005, Citation2006), and this adjuvant effect of LTB was evaluated only in terms of immunogenicity while protection against virulent infection was not investigated in detail. Moreover, the results of these studies were based on observations after supplementation with a recombinant LTB protein, which has a lesser adjuvant effect due to possible destruction of the protein during passage in the intestinal tract of the chicken. In contrast, the present study utilized a live attenuated Salmonella LTB strain, which showed an efficient adjuvant effect possibly due to release of the LTB protein in the gut. Our study also demonstrated that the Salmonella LTB strain efficiently enhanced the antigen-specific plasma IgG and intestinal sIgA responses when co-administered orally.
The adjuvant effect of LTB on mucosal and humoral immune responses has been previously reported in several other studies (Khoury & Meinersmann, Citation1995; Khan et al., Citation2007; da Silva Ramos Rocha et al., Citation2008; Hur & Lee, Citation2011). Immunization with S. Typhi harbouring a chromosomally integrated copy of eltB encoding LTB induced high IgG and IgA responses after oral delivery to humans (Khan et al., Citation2007). In a piglet study, the use of a live attenuated Salmonella strain expressing LTB, when co-administered with a live vaccine containing Salmonella lacking virulence genes, elicited higher IgG and sIgA responses (Hur & Lee, Citation2011). In addition, the present study also demonstrated that immunization with LTB adjuvant strain induced significantly higher antigen-specific lymphocyte proliferation responses (a). The evaluation of lymphocyte proliferation responses after immunization is considered an indicator of immune response at the cellular level (Chaudhari et al., Citation2013). Since APEC bacteria survive inside macrophages (Bastiani et al., Citation2005), a vaccine that can effectively reach macrophages and induce cellular immunity is required to protect against this pathogen. The higher stimulation index for the birds who received Salmonella -delivered APEC vaccines orally along with LTB strain suggests that LTB adjuvant efficiently enhanced the immune response at the cellular level. As a mechanism of action, LTB binds to the GM1 ganglioside receptor of host cells and allows cellular uptake of the co-administered antigen (Fingerut et al., Citation2006), which results in an enhanced immune response (Williams, Citation2000) possibly because of the efficient presentation of the antigen to the macrophages and naïve B cells (Holmgren et al., Citation2003).
In our previous report, we demonstrated that single immunization with only Salmonella -delivered APEC vaccine offered partial protection after oral immunization (Chaudhari et al., Citation2013). In the present study, our results demonstrated that oral immunization with live Salmonella -delivered APEC vaccine along with LTB not only increased mucosal and humoral immune responses, but also promoted cellular immunity. These findings may be due to the increased exposure of the APEC vaccine to the immune system.
We further investigated the LTB strain to determine the optimized dose. Interestingly, when the birds were vaccinated along with 1×108 CFU LTB, neither an increase in the immune response nor protection against APEC challenge was observed. It is well known that oral administration of low doses of antigen favour active cellular suppression whereas high doses favour clonal anergy as a mechanism of oral tolerance (Faria & Weiner, Citation2005). One of the primary mechanisms of the active cellular suppression of immune responses after administration of low doses of antigen is the production of suppressive cytokines such as transforming growth factor β, interleukin-4 and interleukin-10 (Miller et al., Citation1992). When higher doses of antigen are fed, clonal anergy results and causes systemic hyporesponsiveness by deletion of Th1 cells (Whitacre et al., Citation1991; Melamed & Friedman, Citation1993). Thus, administration of large doses of antigen causes clonal anergy and inhibits the Th1 responses, which can be broadly defined as oral tolerance (Miller et al., Citation1992). This might be the reason why APEC immunization with 1×108 CFU of LTB did not enhance the immune response or induce efficient protection. It is possible that 1×108 CFU of LTB is involved in induction of the clonal anergy that might have resulted in the reduced immune response, although further clarification is required. In addition, use of 1×108 CFU LTB might have been involved in the over-production of antibodies against LTB instead of the specific APEC antigens. Although the results of the present study did not clarify this phenomenon, they provide useful information in terms of the fact that use of 1×107 CFU of LTB may provide the optimum adjuvant effect for Salmonella -delivered APEC vaccine.
We have previously demonstrated that single immunization with the Salmonella -delivered APEC vaccine induced partial protection against virulent challenge in broiler chickens (Chaudhari et al., Citation2013). In the present study we report that the LTB strain effectively increased the efficacy of the Salmonella -delivered APEC vaccine after single oral immunization. Vaccination for APEC along with the LTB strain thus appears to increase the efficacy of protection against colibacillosis in broiler chickens.
Acknowledgements
This work was supported by the Mid-career Researcher Program through a National Research Foundation grant funded by the Ministry of Education, Science and Technology (No. 2012-R1A2A4A01002318), Republic of Korea.
References
- Arp , L.H. 1980 . Consequences of active or passive immunization of turkeys against Escherichia coli O78 . Avian Diseases , 24 , 808 – 815 doi: 10.2307/1589958
- Barnes , H.J. , Nolan , L.K. & Vaillancourt , J.P. 2008 . Colibacillosis . In Y.M. Saif , A.M. Fadly , J.R. Glisson , L.R. McDougald , L.K. Nolan , & D.E. Swayne . (2008) . Diseases of Poultry , 12th edn ; pp. 691 – 732 . Ames , IA : Blackwell publishing .
- Bastiani , M. , Vidotto , M.C. & Horn , F. 2005 . An avian pathogenic Escherichia coli isolate induces caspase 3/7 activation in J774 macrophages . FEMS Microbiology Letters , 253 , 133 – 140 doi: 10.1016/j.femsle.2005.09.024
- Chaudhari , A.A. , Matsuda , K. & Lee , J.H. 2013 . Construction of an attenuated Salmonella delivery system harboring genes encoding various virulence factors of avian pathogenic Esherichia coli and its potential as a candidate vaccine for chicken colibacillosis . Avian Diseases , 57 , 88 – 96 .
- Clements , J.D. , Lyon , F.L. , Lowe , K.L. , Farrand , A.L. & El-Morshidy , S. 1986 . Oral immunization of mice with attenuated Salmonella enteritidis containing a recombinant plasmid which codes for production of the B subunit of heat-labile Escherichia coli enterotoxin . Infection and Immunity , 53 , 685 – 692 .
- Conceição , F.R. , Moreira , A.N. & Dellagostin , O.A. 2006 . A recombinant chimera composed of R1 repeat region of Mycoplasma hyopneumoniae P97 adhesin with Escherichia coli heat-labile enterotoxin B subunit elicits immune response in mice . Vaccine , 24 ( 29–30 ), 5734 – 5743 .
- Crouch , S.P. , Kozlowski , R. , Slater , K.J. & Fletcher , J. 1993 . The use of ATP bioluminescence as a measure of cell proliferation and cytotoxicity . Journal of Immunological Methods , 160 , 81 – 88 doi: 10.1016/0022-1759(93)90011-U
- da Silva Ramos Rocha , A. , Conceição , F.R. , Grassmann , A.A. , Lagranha , V.L. & Dellagostin , O.A. 2008 . B subunit of Escherichia coli heat-labile enterotoxin as adjuvant of humoral immune response in recombinant BCG vaccination . Canadian Journal of Microbiology , 54 , 677 – 686 doi: 10.1139/W08-056
- de Haan , L. , Verweij , W.R. , Feil , I.K. , Holtrop , M. , Hol , W.G. , Agsteribbe , E. & Wilschut , J. 1998 . Role of GM1 binding in the mucosal immunogenicity and adjuvant activity of the Escherichia coli heat-labile enterotoxin and its B subunit . Immunology , 94 , 424 – 430 doi: 10.1046/j.1365-2567.1998.00535.x
- Dziva , F. & Stevens , M.P. 2008 . Colibacillosis in poultry: unravelling the molecular basis of virulence of avian pathogenic Escherichia coli in their natural hosts . Avian Pathology , 37 , 355 – 366 doi: 10.1080/03079450802216652
- Faria , A.M. & Weiner , H.L. 2005 . Oral tolerance . Immunology Reviews , 206 , 232 – 259 doi: 10.1111/j.0105-2896.2005.00280.x
- Fingerut , E. , Gutter , B. , Goldway , M. , Eliahoo , D. & Pitcovski J. 2006 . B subunit of E. coli enterotoxin as adjuvant and carrier in oral and skin vaccination . Veterinary Immunology and Immunopathology , 112 ( 3–4 ), 253 – 263 doi: 10.1016/j.vetimm.2006.03.005
- Fingerut , E. , Gutter , B. , Meir , R. , Eliahoo , D. & Pitcovski , J. 2005 . Vaccine and adjuvant activity of recombinant subunit B of E. coli enterotoxin produced in yeast . Vaccine , 23 , 4685 – 4696 doi: 10.1016/j.vaccine.2005.03.050
- Freytag , L.C. & Clements , J.D. 1999 . Bacterial toxins as mucosal adjuvants . Current Topics in Microbiology and Immunology , 236 , 215 – 236 .
- Freytag , L.C. & Clements , J.D. 2005 . Mucosal adjuvants . Vaccine , 23 , 1804 – 1813 doi: 10.1016/j.vaccine.2004.11.010
- Frommer , A. , Freidlin , P.J. , Bock , R.R. , Leitner , G. , Chaffer , M. & Heller , E.D. 1994 . Experimental vaccination of young chickens with a live, non-pathogenic strain of Escherichia coli . Avian Pathology , 23 , 425 – 433 doi: 10.1080/03079459408419013
- Gogal , R.M. Jr , Ahmed , S.A. & Larsen , C.T. 1997 . Analysis of avian lymphocyte proliferation by a new, simple, nonradioactive assay (lympho-pro) . Avian Diseases , 41 , 714 – 725 doi: 10.2307/1592166
- Gyimah , J.E. , Panigrahy , B. & Williams , J.D. 1986 . Immunogenicity of an Escherichia coli multivalent pilus vaccine in chickens . Avian Diseases , 30 , 687 – 689 doi: 10.2307/1590569
- Haan , L. , Verweij , W.R. , Holtrop , M. , Brands , R. , van Scharrenburg , G.J. , Palache , A.M. , Agsteribbe , E. & Wilschut , J. 2001 . Nasal or intramuscular immunization of mice with influenza subunit antigen and the B subunit of Escherichia coli heat-labile toxin induces IgA- or IgG-mediated protective mucosal immunity . Vaccine , 19 , 2898 – 2907 doi: 10.1016/S0264-410X(00)00556-9
- Hayward , C.M.M. , O'Gaora , P. , Young , D.B. , Griffin , G.E. , Thole , J. , Hirst , T.R. , Castello-Branco , L.R. & Lewis , D.J. 1999 . Construction and murine immunogenicity of recombinant Bacille Calmette Guerin vaccine expressing the B subunit of Escherichia coli heat labile Enterotoxin . Vaccine , 17 ( 9–10 ), 1272 – 1281 doi: 10.1016/S0264-410X(98)00350-8
- Holmgren , J. , Czerkinsky , C. , Eriksson , K. & Mharandi , A. 2003 . Mucosal immunisation and adjuvants: a brief overview of recent advances and challenges . Vaccine , 21 , S89 – S95 doi: 10.1016/S0264-410X(03)00206-8
- Hur , J. & Lee , J.H. 2011 . Enhancement of immune responses by an attenuated Salmonella enterica serovar Typhimurium strain secreting an Escherichia coli heat-labile enterotoxin B subunit protein as an adjuvant for a live Salmonella vaccine candidate . Clinical and Vaccine Immunology , 18 , 203 – 209 doi: 10.1128/CVI.00407-10
- Khan , S. , Chatfield , S. , Stratford , R. , Bedwell , J. , Bentley , M. , Sulsh , S. , Giemza , R. , Smith , S. , Bongard , E. , Cosgrove , C.A. , Johnson , J. , Dougan , G. , Griffin , G.E. , Makin , J. & Lewis , D.J. 2007 . Ability of SPI2 mutant of S. typhi to effectively induce antibody responses to the mucosal antigen enterotoxigenic E. coli heat labile toxin B subunit after oral delivery to humans . Vaccine , 25 , 4175 – 4182 doi: 10.1016/j.vaccine.2007.03.007
- Khoury , C.A. & Meinersmann , R.J. 1995 . A genetic hybrid of the Campylobacter jejuni flaA gene with LT-B of Escherichia coli and assessment of the efficacy of the hybrid protein as an oral chicken vaccine . Avian Diseases , 39 , 812 – 820 doi: 10.2307/1592418
- Kwaga , J.K. , Allan , B.J. , van der Hurk , J.V. , Seida , H. & Potter , A.A. 1994 . A carAB mutant of avian pathogenic Escherichia coli serogroup O2 is attenuated and effective as a live oral vaccine against colibacillosis in turkeys . Infection and Immunity , 62 , 3766 – 3772 .
- Maskell , D.J. , Sweeney , K.J. , O'Callaghan , D. , Hormaeche , C.E. , Liew , F.Y. & Dougan , G. 1987 . Salmonella typhimurium aroA mutants as carriers of the Escherichia coli heat-labile enterotoxin B subunit to the murine secretory and systemic immune systems . Microbial Pathogenesis , 2 , 211 – 221 doi: 10.1016/0882-4010(87)90022-2
- McCluskie , M.J. , Weeratna , R.D. , Clements , J.D. & Davis , H.L. 2001 . Mucosal immunization of mice using CpG DNA and/or mutants of the heat-labile enterotoxin of Escherichia coli as adjuvants . Vaccine , 19 , 3759 – 3768 doi: 10.1016/S0264-410X(01)00088-3
- Melamed , D. & Friedman , A. 1993 . Direct evidence for anergy in T lymphocytes tolerized by oral administration of ovalbumin . European Journal of Immunology , 23 , 935 – 942 doi: 10.1002/eji.1830230426
- Melamed , D. , Leitner , G. & Heller , E.D. 1991 . A vaccine against avian colibacillosis based on ultrasonic inactivation of Escherichia coli . Avian Diseases , 35 , 17 – 22 doi: 10.2307/1591289
- Millar , D.G. , Hirst , T.R. & Snider , D.P. 2001 . Escherichia coli heat-labile enterotoxin B subunit is a more potent mucosal adjuvant than its closely related homologue, the B subunit of cholera toxin . Infection and Immunity , 69 , 3476 – 3482 doi: 10.1128/IAI.69.5.3476-3482.2001
- Miller , A. , Lider , O. , Roberts , A.B. , Sporn , M.B. & Weiner , H.L. 1992 . Suppressor T cells generated by oral tolerization to myelin basic protein suppress both in vitro and in vivo immune responses by the release of transforming growth factor β after antigen-specific triggering . Proceedings of the National Academy of Sciences of USA , 89 , 421 – 425 doi: 10.1073/pnas.89.1.421
- Ogra , P.L. , Faden , H. & Welliver , R.C. 2001 . Vaccination strategies for mucosal immune responses . Clinical Microbiology Reviews , 14 , 430 – 445 doi: 10.1128/CMR.14.2.430-445.2001
- Peighambari , S.M. , Hunter , D.B. , Shewen , P.E. & Gyles , C.L. 2002 . Safety, immunogenicity, and efficacy of two Escherichia coli cya crp mutants as vaccines for broilers . Avian Diseases , 46 , 287 – 297 doi: 10.1637/0005-2086(2002)046[0287:SIAEOT]2.0.CO;2
- Pourbakhsh , S.A. , Boulianne , M. , Martineau-Doizé , B. & Fairbrother , J.M. 1997b . Virulence mechanisms of avian fimbriated Escherichia coli in experimentally inoculated chickens . Veterinary Microbiology , 58 , 195 – 213 doi: 10.1016/S0378-1135(97)00163-6
- Pourbakhsh , S.A. , Dho-Moulin , M. , Brée , A. , Desautels , C. , Martineau-Doize , B. & Fairbrother , J.M. 1997a . Localization of the in vivo expression of P and F1 fimbriae in chickens experimentally inoculated with pathogenic Escherichia coli . Microbial Pathogenesis , 22 , 331 – 341 doi: 10.1006/mpat.1996.0116
- Rask , C. , Fredriksson , M. , Lindblad , M. , Czerkinsky , C. & Holmgren , J. 2000 . Mucosal and systemic antibody responses after peroral or intranasal immunization: effects of conjugation to enterotoxin B subunits and/or of co-administration with free toxin as adjuvant . Acta Pathologica, Microbiologica et Immunologica Scandinavica , 108 , 178 – 186 doi: 10.1034/j.1600-0463.2000.d01-42.x
- Stordeur , P. , Marlier , D. , Blanco , J. , Oswald , E. , Biet , F. , Dho-Moulin , M. & Mainil , J. 2002 . Examination of Escherichia coli from poultry for selected adhesin genes important in disease caused by mammalian pathogenic E. coli . Veterinary Microbiology , 84 , 231 – 241 doi: 10.1016/S0378-1135(01)00464-3
- Weltzin , R. , Guy , B. , Thomas , W.D. Jr , Giannasca , P.J. & Monath , T.P. 2000 . Parenteral adjuvant activities of Escherichia coli heat-labile toxin and its B subunit for immunization of mice against gastric Helicobacter pylori infection . Infection and Immunity , 68 , 2775 – 2782 doi: 10.1128/IAI.68.5.2775-2782.2000
- Whitacre , C.C. , Gienapp , I.E. , Orosz , C.G. & Bitar , D.M. 1991 . Oral tolerance in experimental autoimmune encephalomyelitis. III. Evidence for clonal anergy . Journal of Immunology , 147 , 2155 – 2163 .
- Williams , N.A. 2000 . Immune modulation by the cholera-like enterotoxin B-subunits: from adjuvant to immunotherapeutic . International Journal of Medical Microbiology , 290 , 447 – 453 doi: 10.1016/S1438-4221(00)80062-4
- Yamamoto , M. , McGhee , J.R. , Hagiwara , Y. , Otake , S. & Kiyono , H. 2001 . Genetically manipulated bacterial toxin as a new generation mucosal adjuvant . Scandinavian Journal of Immunology , 53 , 211 – 217 .
- Zhang , L. , Zhang , M. , Li , J. , Cao , T. , Tian , X. & Zhou , F. 2008 . Enhancement of mucosal immune responses by intranasal co-delivery of Newcastle disease vaccine plus CpG oligonucleotide in SPF chickens in vivo . Research in Veterinary Science , 85 , 495 – 502 .
- Zhang , X. , Zhang , X. & Yang , Q. 2007 . Effect of compound mucosal immune adjuvant on mucosal and systemic immune responses in chicken orally vaccinated with attenuated Newcastle-disease vaccine . Vaccine , 25 , 3254 – 3262 .
- Zhao , X. , Zhang , M. , Li , Z. & Frankel , F.R. 2006 . Vaginal protection and immunity after oral immunization of mice with a novel vaccine strain of Listeria monocytogenes expressing human immunodeficiency virus type 1 gag . Journal of Virology , 80 , 8880 – 8890 doi: 10.1128/JVI.00894-06